Fig. 15.1
(a) Illustration of the head showing locations of the acoustic windows for transcranial Doppler examination except transforaminal approach . The transtemporal window for transcranial Doppler and color-coded duplex insonation may be found above the zygomatic arch at the (1) middle, (2) posterior, and (3) anterior probe positions. (b) Depiction of the Circle of Willis with time-of-flight MRA (grey image) and TCCD color flow imaging (colored image). Note the hypoechoic butterfly-shaped mesencephalic brainstem (asterisk). Normal spectral Doppler waveforms obtained through the (b) transtemporal as well as (c) submandibular and transorbital windows
Transcranial Color-Coded Duplex
Transcranial color-coded duplex (TCCD) is performed with a phased array transducer using a carrier frequency of 1.5–2.5 MHz and imaging frequency of 4 MHz. It offers a two-dimensional B-mode image that is able to visualize anatomic landmarks in the brain, and the spatial relationship of the vessels can be used for identification of the arteries in the cerebral vasculature [3–7]. TCCD is particularly useful in the detection of distal arterial lesions and tracing the course of tortuous vessels. Also, TCCD enables reliable identification of arterial branches, differentiation of the terminal vertebral arteries from each other (left and right), and tracing of the basilar artery (BA) along its entire course. Using color flow imaging, regions of disturbed flow, suggestive of focal stenoses or occlusions , and occasionally arteriovenous malformations or recurrences of intracranial aneurysms after endovascular therapy can be detected [8, 9]. At bedside, B-mode imaging is able to demonstrate the midline shift with the malignant middle cerebral artery (MCA) infarctions or hematoma growth with intracerebral hemorrhages [10, 11].
However, fewer diagnostic criteria are available for the detection and grading of intracranial disease with TCCD, and although TCCD provides several potential advantages over TCD (the most important being the opportunity for angle correction), a single-channel spectral Doppler interrogation, derivable from both TCD and TCCD, remains the mainstay of diagnosis.
Examination Techniques
There are four acoustic windows for insonation: temporal, orbital, foraminal, and submandibular (Figs. 15.1 and 15.2) [12–14]. The transtemporal approach allows insonation of the MCA, terminal ICA, anterior cerebral (ACA), posterior cerebral (PCA), and communicating arteries. The transorbital approach is used to insonate the ophthalmic artery (OA) and ICA siphon. The transforaminal approach allows the intracranial portion of the vertebral artery (VA) and BA to be insonated through the foramen magnum. The submandibular approach is used to obtain extracranial ICA velocities as they enter the skull. To shorten the time necessary to find the window and different arterial segments, the TCD examination should begin with the maximum power and gate settings (i.e., power 100%, gate 15 mm; if using PMD-TCD, use the smallest gate 3 mm). A complete TCD/TCCD examination should cover all proximal intracranial arterial segments of the Circle of Willis and should identify and store the highest velocity signals and any abnormal or unusual waveforms for each segment. Table 15.1 summarizes normal depths, directions, and mean flow velocities at assumed zero degree angle of insonation for the arteries of the Circle of Willis.


Fig. 15.2
(a) Illustration of the back of the head showing locations of the transforaminal acoustic windows for transcranial Doppler and color-coded duplex insonation. Commonly, stepwise adjustment of the transducer’s angulation and positioning by moving laterally is necessary to trace the proximal, middle, and distal segments of the vertebrobasilar arteries. (b) Depiction of the Y-shaped vertebrobasilar junction with time-of-flight MRA (grey image) and TCCD color flow imaging (colored image). Note the hypoechoic rotund structure close to the transducer (asterisk) corresponding to the foramen magnum. (c) Normal spectral Doppler waveforms obtained from the terminal vertebral and basilar arteries are shown
Table 15.1
Normal depth, direction, and mean flow velocities at assumed zero degree angle of insonation for the arteries of the Circle of Willis
Artery | Depth (mm, adults) | Direction | Childrena (cm/s) | Adults (cm/s) |
---|---|---|---|---|
M2 MCA | 30–45 | Bidirectional | <170 | <80 |
M1 MCA | 45–65 | Toward | <170 | <80 |
A1 ACA | 62–75 | Away | <150 | <80 |
A2 ACA | 45–65 | Toward | N/A | <80 |
ICA siphon | 60–65 | Bidirectional | <130 | <70 |
OA | 40–60 | Toward | Variable | Variable |
PCA | 55–70 | Bidirectional | <100 | <60 |
BA | 80–100+ | Away | <100 | <60 |
VA | 45–80 | Away | <80 | <50 |
Transtemporal Insonation Steps (Applicable to TCD and TCCD)
When using TCCD for transtemporal insonation , firstly set the B-mode insonation depth at 16 cm and aim toward the contralateral window. Visualization of the contralateral concave-shaped hyperechoic skull may indicate a sufficient transtemporal bone window. Decrease the maximum depth to 10–12 cm and aim the probe slightly downward until the butterfly-shaped hypoechoic mesencephalic brainstem can be identified in the axial plane. While sweeping the ultrasound beam slightly up- and downward, color Doppler imaging now enables visualization of the major branches of the Circle of Willis in part and, in some cases, in whole (Fig. 15.1). Adjust the color box size to cover at least the ipsilateral portion of the Circle of Willis and the color velocity scale to ±10–20 cm/s. Increase the color gain until “bleeding” of color occurs into the surrounding tissue. Although the following steps refer to a complete TCD examination, these are also applicable to TCCD study.
- 1.
Set the depth at 50 mm (midpoint of the M1 MCA). Place the probe above the zygomatic arch and aim it slightly upward and anterior to the contralateral ear/window. Find any flow signal and avoid too anterior and too posterior angulations. Find a flow signal directed toward the probe that resembles MCA flow (low-resistance waveform). Store the highest velocity at this depth.
- 2.
Follow the flow signal until it disappears at shallow (30–40 mm) depths (M2 MCA branches). Store any abnormal signal (avoid sampling meningeal arteries at these depths). Return to the midpoint of the M1 MCA.
- 3.
Follow the M1 MCA stem to its origin at 60–70 mm (insonation of the terminal ICA is possible at this depth). Find the ICA bifurcation at approximately 65 mm and obtain both proximal M1 MCA and A1 ACA (away from the probe) signals. Store a bidirectional signal of the bifurcation.
- 4.
Follow the A1 ACA signal to 70–75 mm depths. Store the distal A1 ACA signal at 70 mm.
- 5.
Find the terminal ICA signal just inferior and sometimes slightly posterior to the bifurcation at 60–65 mm. Store any abnormal signal.
- 6.
Return to the ICA bifurcation and slowly turn the transducer posterior by 10–30° (usually there is a flow gap between the bifurcation and PCA signals). Find PCA signals directed toward (P1) and away (P2) from the probe at a depth range of 55–75 mm. Store the PCA signals with the highest velocity.
Transorbital Insonation Steps (Applicable to TCD)
- 1.
Decrease power to the minimum (17 mW) or 10%. Set the depth at 50–52 mm and place the transducer over the eyelid and angle it slightly medially. Determine flow pulsatility and direction in the distal OA (high pulsatility flow toward the probe). Store the OA signals.
- 2.
Set the depth at 60–64 mm and find the ICA siphon flow signals (usually located medially in the orbital window). Store bidirectional or unidirectional signals directed toward (lower limb of the siphon) and/or away (upper limb of the siphon) from the probe.
Transforaminal Insonatio n Steps (Applicable to TCD and TCCD)
On TCCD, the vertebrobasilar arteries are examined in the axial imaging plane. Patients should be placed in a sitting position with the head bent forward as much as possible. In patients not able to sit (e.g., due to an acute neurological deficit), the examination can be performed in the side posture instead, but the head still bent forward. Place the transducer approximately 2–3 cm below the occipital protuberance in the midline of the neck and slide the transducer’s sound beam slightly toward the bridge of the nose. Once the foramen magnum is visualized as hypoechoic rotund structure close to the transducer on B-mode, color Doppler imaging enables visualization of the vertebrobasilar vasculature (ideally in the form of a Y – “Y-sign”) (Fig. 15.2). Commonly, the VA cannot be visualized along its entire course on a single plane, thus, requiring stepwise adjustment of the transducer’s angulation and positioning by moving laterally to completely trace all segments of the vertebrobasilar arteries. If the distal BA cannot be detected with TCCD, TCD may facilitate examination of the BA along its rostral course.
The following steps refer to a complete TCD examination but are also applicable to TCCD study.
- 1.
Use full power and set the depth at 75 mm (distal VA/proximal BA). Place the transducer at midline one inch below the edge of the skull and aim it at the bridge of the nose. Identify a flow signal directed away from the probe (i.e., find the window).
- 2.
Increasing the depth, follow the flow directed away from the probe. Store the proximal BA signal arbitrarily assigned to the depth of 80 mm (mid-BA to 90 mm, distal BA to >100 mm). Bidirectional signals may be found at various depths resembling cerebellar arteries. Store the highest velocity signal obtained at the most distal BA.
- 3.
Set the depth at 60 mm and place the transducer about one inch laterally to the midline and aim toward the bridge of the nose or slightly toward the contralateral eye. Find the VA flow signal directed away from the probe and follow its intracranial course from 40 to 80 mm. Store the VA signal at 60 mm (or at the depth with the highest velocity). Repeat the examination on the contralateral side.
Submandibular Insonation Steps (Applicable to TCD)
- 1.
Set the depth at 50–60 mm, place the probe laterally under the jaw, and aim it upward and slightly medially. Find a low-resistance flow directed away from the probe. Store the distal ICA signal at the depth that shows the highest velocity. This approach is reserved for patients with subarachnoid hemorrhage (SAH) when vasospasm grading requires calculation of the Lindegaard index.
The TCD/TCCD interpretation consists of the assessment of:
- 1.
Flow mean and peak velocity changes (focal or global)
- 2.
Asymmetry of flow parameters (side-to-side, segmental)
- 3.
Pulsatility (high or low resistance)
- 4.
Waveform/sound pattern recognition of flow changes
A normal TCD/TCCD examination may reveal a wide range of depths of insonation, velocity values, and waveforms.
Criteria for Normal TCD/TCCD Findings
- 1.
Good windows of insonation, all proximal arterial segments found.
- 2.
Directions of flow and depths of insonation are given in Table 15.1.
- 3.
The differences between flow velocities and pulsatility indices in the homologous arterial segments are usually less than 30%: 15% is attributable to the difference in angle of insonation and another 15% to breathing cycles. Normal variations of up to 100% in flow velocities between homologous segments such as PCA or VA can be attributed to a tortuous vessel course, anatomic variants of the circle of Willis, and subdominance/hypoplasia.
- 4.
A normal MCA mean flow velocity (MFV) is in the range of 80–100 cm/s in adults free of anemia and does not exceed 170 cm/s in children with sickle cell disease.
- 5.
A normal velocity ratio: MCA ≥ ACA ≥ siphon ≥ PCA ≥ BA ≥ VA.
- 6.
A positive end-diastolic flow velocity (EDV) of 25–50% of the peak systolic velocity (PSV) values and a low-resistance pulsatility index (PI) of 0.6–1.1 are present in all intracranial arteries while breathing room air. A normal high-resistance flow pattern (PI ≥ 1.2) is seen in the OAs only.
- 7.
Abnormal high-resistance flow patterns can be seen in patent cerebral arteries with aging, chronic hypertension, increased cardiac output, and during hyperventilation.
- 8.
Flow velocities obtained with TCCD should be angle corrected (preferably close to zero degree) and may yield more accurate velocity measurements than with TCD alone.
Cerebrovascular Resistance and Hemodynamic Indices
Cerebrovascular resistance (CVR) is determined by several factors that impede cerebral blood flow (CBF) and thus determine MFV: the vessel radius, length, and blood viscosity. The Hagen-Poiseuille law is often used to describe the impact of resistance on blood flow. It states that the flow of a fluid through a tube is directly proportional to the pressure difference that exists between two ends of a tube and to the fourth power of the vessel radius and inversely proportional to the length of the tube and fluid viscosity. Thus, the diameter of a blood vessel, which is mostly accomplished by arterial-arteriolar constriction and dilatation, is the most significant contributor of all to the rate of blood flow. Under normal conditions, the brain is a low-resistance arterial system, and the ICA branches have considerably greater end-diastolic flow than the external carotid artery branches. The resistance to flow is described by the pulsatility (PI) and the resistance index (RI):
PI (Gosling − King) = PSV − EDV/MFV (normal values in the range of 0.6 – 1.1).
RI (Pourcelot) = PSV − EDV/PSV (normal values are in the range of 0.4 – 90.63) [15].
These indices quantitatively reflect CVR and depend largely on the strength of the signal recorded as well as the envelope and time averaging software. Therefore “weak signals,” “poor” windows, incomplete examination, and individual variations may produce substantial drawbacks. Additional information can be gained by visual flow pattern recognition. Pathologically increased pulsatility indices (≥1.2) are seen with increased ICP and decreased cerebral perfusion pressure due to decreased EDV. Further increased PIs can be detected proximal to a severe arterial obstruction, in patients with chronic hypertension and during episodes of hypocapnia. Pathologically low pulsatility indices (≤0.5) are mainly seen in the arteries feeding arteriovenous malformations since direct shunting of blood into the venous system results in abnormally low CVR [16]. Low PIs may also be seen distal to a severe extra- or intracranial stenosis indicating compensatory vasodilation and during hypoventilation. Although these indices per se may not be sufficient to diagnose extra- or intracranial cerebrovascular diseases, they should be used in a battery of TCD parameters allowing integrated assessment of intracranial circulation.
The hemispheric index (also known as the Lindegaard ratio ) compares MCA MFV to the ipsilateral ICA and may help to differentiate an MCA MFV increase due to vasospasm and/or hyperperfusion [17]. The M1 MCA vasospasm after subarachnoid hemorrhage (SAH) is usually associated with a Lindegaard ratio ≥3, while a severe M1 MCA vasospasm has been shown to correlate with values ≥6.
Other factors that affect CBF and CVR include age, gender, cardiac function, hematocrit, fibrinogen, and vasodilatory or constricting medications [15]. TCD measurement of flow velocities, however, does not allow calculation of CBF in ml/min because of the variable and unknown arterial diameter and peripheral resistance [18]. However, the area under the waveform envelope, intensity of the signal, and relative change in the mean flow velocities determined with the same angle of insonation are usually proportional to regional CBF changes [19]. For example, MFV changes due to vasomotor reactivity with different vasodilatory stimuli reflect CBF changes assuming that the perfused territory remains constant during investigation [20]. The use of TCD for monitoring physiological responses to vasodilatory substances is based on the concept of cerebral vasomotor reactivity (VMR) in order to identify patients at a particular risk for cerebral ischemic events [21].
Clinical Indications and Diagnostic Criteria
Most clinical indications are established for TCD as corresponding validation studies were conducted when TCCD was not readily available or broadly utilized. However, since most of the diagnostic criteria refer to Doppler spectra analysis and waveform interpretation, these criteria are also applicable to TCCD examination . In the following we detail established clinical indications and diagnostic criteria for TCD/TCCD in routine clinical practice (see also Table 15.2) [22, 23].
Table 15.2
Established clinical indications for and expected outcomes of TCD testing
Broad indication | Specific indications | Expected outcomes |
---|---|---|
Sickle cell disease | Children | Robust first-ever stroke risk reduction based on TCD criteria for the need of blood transfusion and continuing use of blood transfusions |
Ischemic stroke or TIA | Patients with acute ischemic symptoms who had cranial CT or MRI | TCD can identify patients with proximal arterial obstructions in the anterior and posterior circulation, identifying patients amenable to reperfusion therapies (intravenous thrombolysis or endovascular therapy) |
Ischemic stroke or TIA | Patients with subacute ischemic symptoms who had cranial CT or MRI | TCD helps determine stroke pathogenic mechanism that in turn determines secondary stroke prevention treatment. TCD also helps to localize and grade intracranial atheromatous disease process, (anterior vs. posterior vessels, diffuse vs. local disease, ≥70% stenosis that indicate high risk of stroke recurrence) |
Ischemic stroke or TIA | Symptomatic patient at any time window who underwent carotid duplex scanning | Carotid duplex ultrasound may explain only 15–25% of all ischemic events since the prevalence of ≥50% proximal ICA stenosis is low. TCD has the ability to further refine stroke mechanism detection by determining the presence of intracranial steno-occlusive disease, embolization, shunting, and impaired vasomotor reactivity |
Ischemic stroke or TIA | Patients with undetermined stroke mechanism, recurrent TIAs, artery-to-artery versus cardiac source of embolism, suspected arterial dissections | TCD is the gold standard test to detect, localize, and quantify cerebral embolism in real time. No other modality offers spatial and time resolution to detect microembolic activity, localize its source (artery vs. heart), and confirm vascular etiology of patient symptoms |
Ischemic stroke or TIA | Patients with suspected paradoxical embolism with negative echocardiography | TCD is equal or superior in its sensitivity to the presence of any right-to-left shunt compared to echocardiography (Valsalva maneuver is best accomplished during TCD; extracardiac shunting can be detected with TCD but not TEE) |
Ischemic stroke or TIA | Follow-up | TCD is an inexpensive noninvasive follow-up tool that can detect progression or regression in the severity of extra- and intracranial stenosis through direct velocity measurements, collaterals, and vasomotor reactivity assessment |
Asymptomatic or symptomatic carotid artery stenosis or occlusion | Patients who have ICA stenosis or occlusion on carotid duplex or angiography | TCD can help identify patients at highest risk of first-ever or recurrent stroke in the setting of an ICA stenosis of variable degree or complete occlusion. TCD findings of artery-to-artery embolization and impaired vasomotor reactivity indicate three-–fourfold higher risk of stroke compared to patients with similar degree of ICA stenosis and normal TCD findings |
Subarachnoid hemorrhage | Day 2–5 | TCD can detect the development of vasospasm days before it can become clinically apparent, and this information can be used by intensivists to step up with hemodynamic management of these patients |
Day 5–12 | TCD can detect progression to the severe phase of spasm when development of the delayed ischemic deficit due to perfusion failure through the residual lumen is the greatest. This information can help planning interventions | |
Day 12–end of ICU stay | TCD can document spasm resolution after treatment or intervention, sustainability of vessel patency, and infrequent cases of late or rebound vasospasm development at the end of the second or into the third week after subarachnoid hemorrhage | |
Suspected brain death | Increased intracranial pressure | TCD can rule out cerebral circulatory arrest if positive diastolic flow is detected at any ICP values. TCD can confirm clinical diagnosis of brain death by demonstrating complete cerebral circulatory arrest in anterior and posterior circulation |
Peri-procedural or surgical monitoring | Carotid endarterectomy or stenting | TCD can detect all major causes of perioperative complications, i.e., embolism, thrombosis, hypoperfusion, and hyperperfusion. TCD detects real-time flow changes that precede the development of neurological deficits or changes on electroencephalography |
Sickle Cell Disease
Transcranial Doppler constitutes the modality of choice to identify and monitor children who are at highest risk of incidental stroke and in need of blood transfusion for primary stroke prevention. In a pivotal trial by Adams et al., blood transfusion resulted in 90% relative risk reduction of first stroke in children with elevated TCD time-averaged mean of the maximum velocities [TAMM] (abnormal: TAMM ≥200 cm/s) on two separate examinations [24]. Moreover, long-term follow-up data from the Stroke Prevention Trial in Sickle Cell Anemia (STOP) trial indicated that persistent elevation in TCD velocities are associated with an ongoing stroke risk, and children who were initially selected by TCD for blood transfusion should stay on transfusion schedule to sustain the benefit in stroke risk reduction [25, 26]. However, previously published long-term data from the newborn-CHIC-Créteil-cohort indicated that in a subset of children on hydroxyurea treatment blood transfusions can be stopped when TCD velocities are normalized (normal: TAMM <170 cm/s) but should undergo trimestrial Doppler follow-up and receive transfusions in case of relapse into abnormal TCD findings [27]. Table 15.1 details normal TAMM velocity values in the cerebral arteries for children with sickle cell disease [28].
Cerebral Vasospasm and Hyperperfusion
Up to 30% of patients with subarachnoid hemorrhage (SAH) experience clinical deterioration caused by delayed cerebral ischemia mostly between days 4 and 10 after the index event [29, 30]. Delayed cerebral ischemia usually occurs when arterial vasospasm results in a severe (≤1 mm) intracranial arterial narrowing producing flow depletion with extremely high velocities [31]. It may affect the proximal and distal segments of intracranial arteries with the most common locations being:
- 1.
MCA/TICA ± ACA
- 2.
Bilateral ACAs
- 3.
BA ± terminal VA
- 4.
Distal branches [32]
Although quantitative criteria have been studied extensively, grading arterial vasospasm severity remains difficult and the interpretation of TCD findings should be individualized. Daily TCD examinations (from the first day after SAH onward) may detect considerable flow velocity and pulsatility changes that may be attributed to early development of vasospasm and progression to a severe stage [23, 33–41]. For example, an MCA MFV increase by 50 cm/s may indicate a 20% diameter reduction of the vessel, and since flow velocity is inversely proportionate to the vessel radius, a 50% diameter reduction usually doubles the velocity on TCD [42]. Therefore TCD may be more sensitive to changes in intracranial artery diameter than angiography particularly at the early phases of vasospasm development. Since TCD is a screening tool, the criteria should be adjusted to a higher sensitivity to detect any degree of vasospasm in order to institute components of hemodynamic therapy (i.e., controlled hypervolemia and hypertension). At the same time, a higher specificity threshold should be used for severe vasospasm to minimize the number of false-negative angiograms, particularly if TCD is used to select patients for endovascular therapy. The use of Lindegaard ratio may help to minimize false-positive TCD results and predict better the diameter of the residual lumen on angiography [17, 42]. While TCD has its highest sensitivity f or detecting severe arterial vasospasm around 8 after SAH, its sensitivity for diagnosing delayed cerebral ischemia is lower [41]. However, TCD findings should be related to the patient clinical condition, medications, blood pressure, and time after day zero and ICP findings.
In general, we use the following criteria for arterial vasospasm grading:
- 1.
Proximal vasospasm in any intracranial artery results in a focal (or present along one or two branching segments) elevation of mean flow velocities without a parallel velocity increase in the feeding extracranial arteries (intracranial/extracranial vessel ratio ≥3).
- 2.
Distal vasospasm in any intracranial artery may produce a focal pulsatile flow (PI ≥ 1.2) indicating increased resistance distal to the site of insonation. No MFV increase may be found since vasospasm is located distal to the site of insonation.
Additional findings may include daily changes in velocities, ratios, and PIs during the first 2 weeks but are particularly pronounced during the critical days 3–7 after the onset of SAH. MCA vasospasm specific criteria are shown in Table 15.3. The differential diagnosis includes hyperemia, combination of vasospasm and hyperemia in the same vessel (particularly when SAH patients receive some part of hypertension-hypervolemia therapy), residual vasospasm, and hyperemia. Arterial vasospasm may also coexist with hydrocephalus and edema.
Table 15.3
TCD criteria for grading vasospasm in the proximal MCA
Mean flow velocity (cm/s) | MCA/ICA MFV ratio | Interpretation |
---|---|---|
<120 | ≤3 | Hyperemia |
≥120 | 3–4 | Development of mild spasm + hyperemia |
≥120 | 4–5 | Moderate spasm + hyperemia |
≥120 | 5–6 | Moderate spasm |
≥180 | 6 | Moderate-to-severe spasm |
≥200 | ≥6 | Severe spasm |
>200 | 4–6 | Moderate spasm + hyperemia |
>200 | 3–4 | Hyperemia + mild (often residual) spasm |
>200 | <3 | Hyperemia |
Prognostically unfavorable signs include an early appearance of MCA MFV ≥180 cm/s, a rapid (>20% or >65 cm/s) daily MFV increase during critical days 3–7, an MCA/ICA ratio ≥6, and the abrupt appearance of high-resistance PI ≥1.2 of flow in two or more arteries indicating increased ICP and/or distal vasospasm [30].
Grading vasospasm severity in the arteries other than MCA is difficult [22]. Sloan et al. showed that TCD is highly specific for VA and BA vasospasm when mean flow velocities are ≥80 and ≥95 cm/s, respectively, and suggested reporting vasospasm in these arteries as possible, probable, and definite (Table 15.4) [34]. Also, the use of the ratio >3 between the BA MFV and the extracranial VA MFV increases sensitivity and specificity for TCD detection of BA vasospasm [37–39].
Table 15.4
Sloan’s optimized criteria for grading vasospasm (VSP) in intracranial arteries
Artery/MFV | Possible VSP | Probable VSP | Definite VSP |
---|---|---|---|
ICA | >80 | >110 | >130 |
ACA | >90 | >110 | >120 |
PCA | >60 | >80 | >90 |
BA | >70 | >90 | >100 |
VA | >60 | >80 | >90 |
Other hyperperfusion syndromes may occur after carotid endarterectomy (CEA) or carotid artery stenting (i.e., post-revascularization hyperemia) usually characterized by a ≥30% increase in MCA MFV unilateral to the reconstructed carotid artery and low-pulsatility waveforms compared with the contralateral side or >100% increase in MCA MFV from pre- to post-intervention, indicating decreased capacity of distal vasculature to regulate the reestablished flow [43–46]. Hyperemic reperfusion may also develop with intravenous thrombolysis and endovascular therapy for acute ischemic stroke [47].
Transcranial ultrasound may also play a role in monitoring of patients with cerebral vasoconstriction syndrome with elevated MFV >120 cm/s and Lindegaard index >3 being predictive of developing posterior leukoencephalopathy or ischemic strokes [48].
Acute Cerebral Ischemia
Transcranial Doppler can be used to identify the presence and location of an intracranial steno-occlusive lesion confirming the vascular etiology of the patient’s neurological symptoms, assess collateral flow to the vascular territory distal to the steno-occlusive lesion, and identify underlying stroke pathogenic mechanisms (i.e., large-vessel stenosis or artery-to-artery embolism as opposed to a lacunar stroke or cardiac embolism source) [48–56]. For instance, in patients with suspected extracranial carotid artery stenosis, carotid duplex findings should be always interpreted in the context of intracranial TCD findings. Notably, findings such as unilateral downstream flow changes (e.g., delayed systolic flow acceleration) and/or intracranial collaterals detectable by TCD help to accurately grade the degree of extracranial carotid artery stenosis and eventually decide upon revascularization therapy [57]. Carotid duplex alone can miss a significant (≥50%) carotid stenosis or occlusion, particularly when regions of interest are not accessible for duplex ultrasound examination due to a tortuous course of the carotid artery or high bifurcation, or the stenotic lesion is obscured by calcified plaque (resulting in a hypoechoic shadow).
For the most general homodynamic model that is frequently used to interpret Doppler velocity findings in a variety of arterial lesions and circulatory conditions, see the Spencer’s curve (Fig. 15.3) [58].


Fig. 15.3
The Spencer’s curve : this hemodynamic model is frequently used to interpret velocity findings in a variety of arterial lesions and circulatory conditions. Stenotic ranges from normal/mild to moderate/severe and near-occlusive states (a–c) are shown with corresponding velocity and waveform findings. (a) With normal vessels or lesions less than 50% stenoses, normal waveforms and normal velocity ranges are expected as well as normal ranges of applicable ratios, i.e., target vessel to feeding vessel. (b) With moderate ≥50% stenoses and severe lesions, focal velocity increases are expected with applicable ratios exceeding 2 or greater values. If abnormally high velocity is detected with hyperperfusion/hyperemia, this can be differentiated from a focal stenosis by a low ratio. Bruit (white arrow) can be present, but these do not necessarily identify stenoses versus hyperemia. (c) With near-occlusive lesions, the velocity paradoxically drops (“the other side of the Spencer’s curve”). These lesions can be differentiated from normal vessels and mild disease by the presence of abnormal waveforms or disproportionately abnormal ratios
Middle Cerebral Artery Stenosis
Primary findings for any significant ≥50% MCA stenosis include a focal significant MFV or PSV increase (≥100 cm/s or ≥140 cm/s, respectively), or interhemispheric MFV difference of 1:2 in adults free of cerebrovascular disease [59–68]. A ≥70% MCA stenosis could produce higher velocities, and in our laboratory we use an MFV cutoff >120 cm/s [69]. This velocity increase should be further associated with a ratio to a homologous segment of 1:3 or higher. If anemia, congestive heart failure, and other circulatory conditions associated with elevated or decreased velocities, a focal MFV difference ≥30% between neighboring or homologous arterial segments should be applied to see if the velocity increase reaches any significance. Adult patients with anemia or hyperthyroidism often have increased MCA mean flow velocities. In children with sickle cell disease, MCA TAMM ≥170–200 cm/s is considered conditional and ≥200 cm/s abnormal [22, 23].
Additional findings may include turbulence (occasionally a low-frequency noise produced by non-harmonic co-vibrations of the vessel wall and musical murmurs due to harmonic co-vibrations producing pure tones can be heard) or disturbed flow distal to the stenosis or presence of characteristic flow voids on PMD-TCD indicating a low-frequency bidirectional turbulent flow during systole; an increased unilateral ACA MFV may indicate compensatory flow diversion or A1 ACA or ICA bifurcation stenosis and microembolic signals (MES) found in the distal MCA.
If MFVs are increased throughout the M1 MCA, the differential diagnosis includes MCA stenosis, terminal ICA or siphon stenosis, hyperemia, or compensatory flow increase in the presence of contralateral ICA stenosis, ACA occlusion, and incorrect vessel identification.
A critical stenosis or obstruction with near-occlusive thrombus or embolus can either produce a focal velocity increase or a blunted MCA waveform with slow or delayed systolic acceleration, slow systolic flow deceleration, low velocities, and lower MFVs in the MCA than ACA or any other intracranial artery [53, 55]. Decreased or minimal flow velocities with slow systolic acceleration can be also found due to a tight elongated MCA stenosis. These focal lesions should be differentiated from a proximal ICA obstruction that can also produce delayed systolic flow acceleration in the MCA [49–56]. To confirm the presence of a flow-limiting lesion, arterial branches need to be evaluated. Note that an M1-M2 MCA near-occlusion is usually accompanied by flow diversion to the ACA and/or compensatory flow increase in the PCA, indicating transcortical collateralization [55, 70].
Anterior Cerebral Artery Stenosis
Primary findings for any significant ACA stenosis include a focal significant mean flow velocity increase (ACA > MCA) and/or a ≥30% difference between the proximal and distal ACA segments and/or interhemispheric ACA MFV difference of ≥30% (these are the original criteria, but in reality ACA MFV velocity should at least double the velocity of the reference vessel) [53, 65, 70]. According to a recent TCD validation study, a PSV of 120 cm/s combined with additional parameters (e.g., abnormal spectrum, side-to-side difference) was the most accurate criterion for detecting substantial ACA stenosis [71]. Collateralization via the anterior communicating artery (AComA) can be excluded by a normal contralateral ACA flow direction. The differential diagnosis includes anterior cross-filling due to a contralateral proximal carotid artery obstruction [49–52].
Additional findings may include turbulence and a flow diversion into the MCA and/or compensatory flow increase in the contralateral ACA. Decreased or minimal flow velocities at the A1 ACA origin may indicate a suboptimal angle of insonation from the unilateral temporal window, an atretic or tortuous A1 ACA segment, or A1 ACA near-occlusion. Since the A2 ACA segment cannot be assessed directly by TCD, its obstruction can be suspected only if a high-resistance flow is found in the distal dominant A1 ACA segment.
Common errors include incorrect vessel identification (terminal ICA vs. ACA), velocity underestimation (suboptimal angle of insonation, poor window, weak signals), and inability to differentiate a collateral flow from the stenosis.
Terminal Internal Carotid Artery and Siphon Stenosis
A terminal ICA (traceable through the temporal window) and ICA siphon (traceable through the transorbital window) stenosis produces a focal significant MFV increase (ICA > MCA, and/or an ICA MFV ≥70 cm/s, and/or ≥30% difference between arterial segments) [53, 65, 72, 73].
The differential diagnosis includes moderate proximal ICA stenosis and/or compensatory flow increase with contralateral significant ICA obstruction. Additional findings in the presence of an ICA stenosis may include turbulence, blunted unilateral MCA, OA MFV increase, and/or flow reversal with low pulsatility. The ICA siphon MFV may decrease due to siphon near-occlusion (a blunted siphon signal) or distal obstruction (i.e., MCA occlusion or increased ICP).
Common errors include incorrect vessel identification such as MCA versus terminal ICA via transtemporal approach or ACA versus ICA with deep transorbital insonation and consequently collateralization of flow via the anterior cross-filling misinterpreted as an arterial stenosis. In the absence of temporal windows, these findings may be the only yet confusing indicators of a significant carotid artery lesion.
Posterior Cerebral Artery Stenosis
A focally significant PCA stenosis results in an MFV increase PCA > ACA or ICA and/or a PCA MFV ≥50 cm/s in adults [56, 66, 74]. Additional findings may include turbulence and a compensatory flow increase in the MCA.
The differential diagnosis includes collateral flow via the posterior communicating artery (PComA) either toward the posterior circulation in case of BA occlusion or toward the anterior circulation in case of MCA, TICA or tandem extracranial ICA/MCA occlusions, and siphon obstruction. Of note, PComA is a tortuous vessel, and its flow direction on TCD does not necessarily indicate where the lesion is that requires collateralization. Using TCCD, it may be possible to differentiate PCA stenosis from collateralization of flow using PSV [75]. Common sources of error include unreliable vessel identification and a top-of-the-basilar stenosis.
Basilar Artery Stenosis
Primary findings in the presence of a BA stenosis include a focal significant MFV increase BA > MCA or ACA or ICA, and/or an MFV BA ≥60 cm/s in adults, and/or a ≥30% difference between arterial segments [56, 66]. The findings of the Stroke Outcomes and Neuroimaging of Intracranial Atherosclerosis (SONIA) trial indicate that a BA stenosis of ≥50% can be more reliably identified when BA MFV exceeds 80 cm/s [68]. To detect ≥70% intracranial vertebrobasilar stenosis, we use MFV increase >110 cm/s and a stenotic-pre-stenotic ratio ≥3 [69].
The differential diagnosis includes a terminal VA stenosis if elevated velocities are found proximally, i.e., at a depth of 70–80 mm. If elevated velocities are found throughout the entire BA course, the differential diagnosis includes a compensatory flow velocity increase, i.e., in case of a carotid artery obstruction. With the latter, velocities are increased in at least one of the VAs.
Basilar artery near-occlusion produces a focal MFV decrease (≤30% difference between neighboring arterial segments, and/or BA < VA) resulting in a blunted waveform [56]. The differential diagnosis includes a fusiform (dolichoectatic) BA with or without thrombus since an enlarged vessel diameter may reduce flow velocities. If the end-diastolic flow is absent, the differential diagnosis includes BA occlusion or tortuosity with branch insonation at a suboptimal angle.
Additional findings may include turbulence and disturbed flow signals distal to the stenosis, compensatory flow increase in VAs and posterior cerebellar inferior arteries indicating cerebellar collateralization, and a collateral supply via PComA to PCA with a reversed flow in the distal BA.
Common sources of error include a tortuous basilar (“not found” does not always mean obstructed), elongated BA obstruction, and distal BA lesions that were not reached by TCD or identified due to flow presence to the superior cerebellar arteries producing false-negative results. Application of ultrasound contrast and TCCD may help the detection of the distal BA segment, tortuosity, and distal branches [7, 56, 76, 77]. A collateral flow from the posterior to the anterior circulation in the presence of carotid lesions may increase flow velocity changes associated with mild stenosis and/or tortuosity. In the case of flow collateralization, the dominant VA velocities are also increased [78]. Reversed flow in the BA (low-resistance flow toward the probe) is characteristic for a proximal BA occlusion with retrograde filling of the distal BA through the PComA [56, 79]. The origin of the collateral flow in the anterior circulation can be confirmed with carotid artery tapping that can be transmitted to the reversed flow.
Intracranial Vertebral Artery Stenosis
Primary findings of an intracranial VA stenosis include a focal MFV increase VA > BA, and/or MFV VA ≥50 cm/s in adults, and/or a ≥30% difference between VAs or its neighboring segments [66, 80]. Recent findings have indicated that a ≥50% VA stenosis can be more reliably identified when VA MFV exceeds 80 cm/s and when the stenotic-to-normal MFV ratio is ≥2 [68]. A ≥70% VA stenosis could produce higher velocities, and in our laboratory we use a MFV cutoff >110 cm/s and a stenotic-to-normal MFV ratio of ≥3 [69]. On TCCD, minimal flow on color imaging with otherwise good visualization of the contralateral VA and significant reduction of the PSV as compared with the contralateral segment may indicate critical stenosis of the VA [81]. The differential diagnosis includes proximal BA or contralateral terminal VA stenoses and a compensatory flow increase in the presence of a contralateral VA occlusion or carotid lesion.
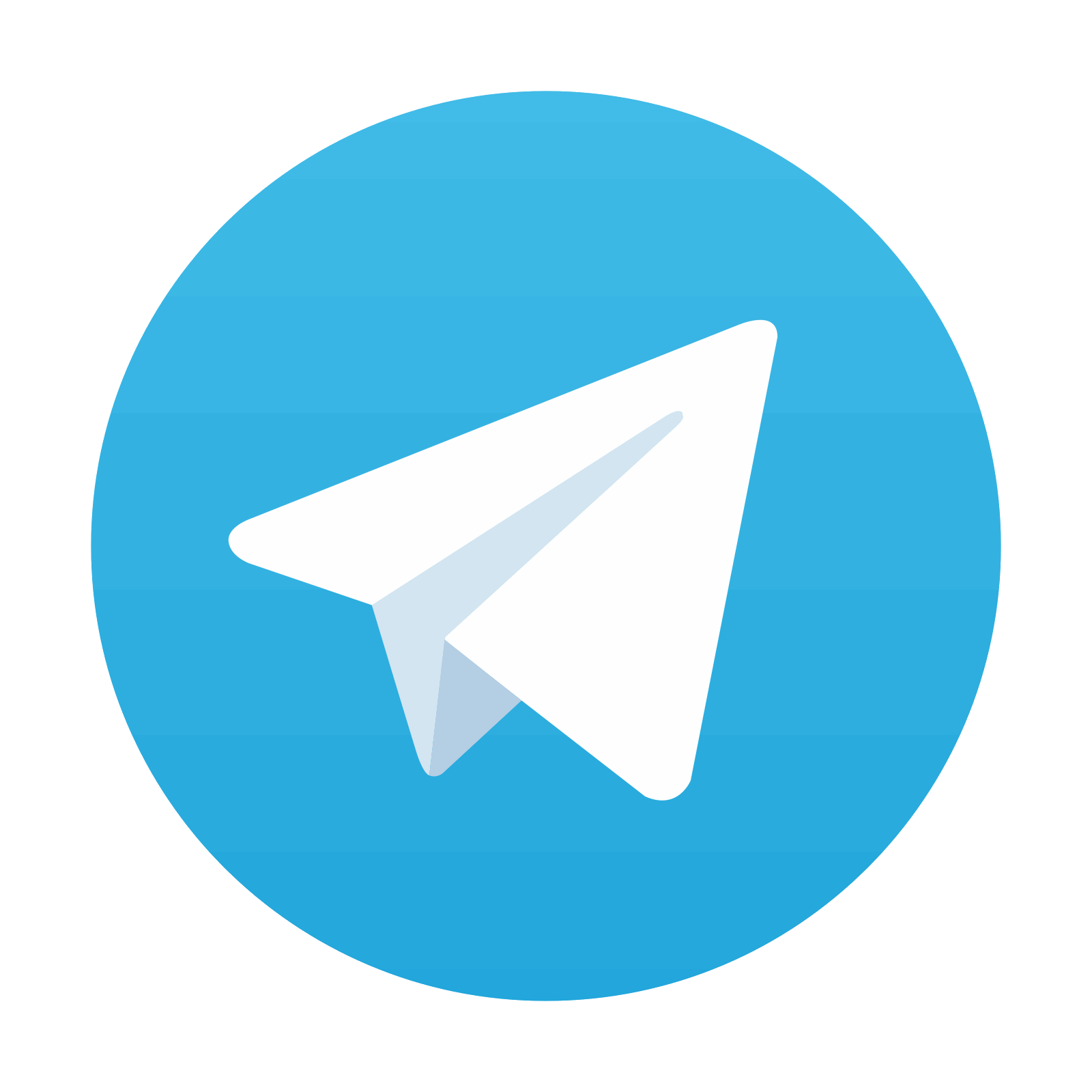
Stay updated, free articles. Join our Telegram channel

Full access? Get Clinical Tree
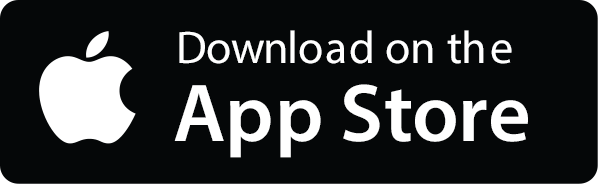
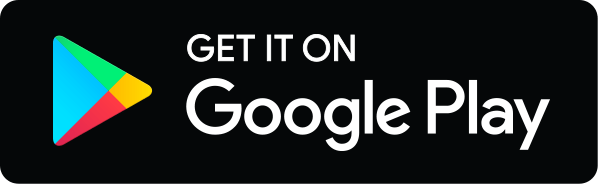