Fig. 36.1
Two potential approaches for the delivery of a transcatheter pulmonic valve : (A) transvenously into the right atrium, then through the tricuspid valve, or (B) transapically through the right ventricular wall. The later approach would require a minimally invasive surgery
The needs of congenital heart patients inspired the original creation of the transcatheter-delivered stented valve. As noted above, Philipp Bonhoeffer is the pioneer of this technology. One example of such a replacement system is the Melody® Transcatheter Pulmonary Valve (Medtronic, Inc., Minneapolis, MN, USA) [3]. The stent supporting the valve is composed of a platinum iridium alloy, which is expanded during delivery by balloons located within the delivery system. The valve is that of a native bovine jugular valve isolated from the vein, which is sewn to the stent. The Melody valve is delivered using the Ensemble® Transcatheter Delivery System (Medtronic, Inc.) through the cardiovascular system, eliminating the need to open the patient’s chest (Fig. 36.2). The overall procedure minimizes trauma and offers a quicker recovery than traditional surgical procedures. Furthermore, it is considered that such procedures: (1) could reduce the total number of surgeries required by these patients during their lifetimes (e.g., by postponing time to surgery while restoring pulmonic function), (2) would allow for earlier intervention and potentially better outcomes for patients while avoiding surgical complications, (3) avoid the risks of bleeding and infection associated with reoperation, and/or (4) reduce costs by avoiding postoperative intensive care [3, 6, 7].
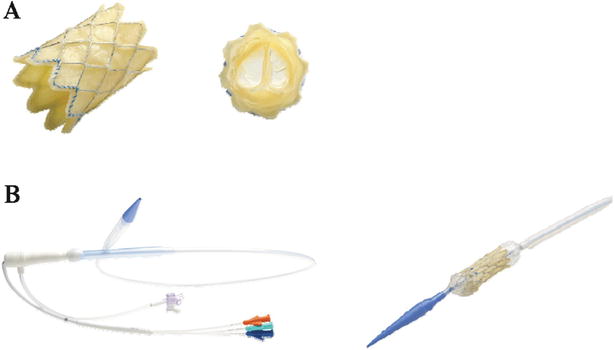
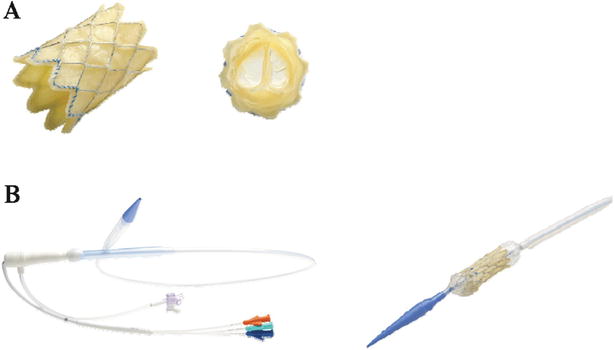
Fig. 36.2
The Melody® Transcatheter Pulmonary Valve and Ensemble® Transcatheter Delivery System (Medtronic, Inc.) has received CE mark approval and is available for distribution in Europe. Additionally, a Medical Device License has been granted and the system is available for distribution in Canada. Products are available for sale in the United States for patients that have congenital heart disease. The system consists of a bovine jugular valve vein sewn inside a platinum iridium stent (A) and delivered via a sheathed balloon-in-balloon delivery catheter (B)
Currently, there are two commercially available valves that have been used for transcatheter pulmonary valve replacements: the Melody (Medtronic, Inc.) and SAPIEN XT (Edwards Lifesciences, Irvine, CA, USA). To date, the Melody valve is FDA approved for patients with congential heart disease in the United States and CE marked in Europe, whereas the SAPIEN XT valve is CE marked in Europe. The largest difference between these two balloon-expandable valves is their sizing, with the Melody® valve being 18–22 mm in diameter and the Sapien XT being 23 or 26 mm. In a recent clinical assessment, both valves performed very comparably [8].
It is common that developers of these technologies partner with leading congenital interventional cardiologists and cardiac surgeons. Furthermore, managing these complex congenital heart disease patients requires a cohesive team approach. It is likely that such patients will be treated in the hybrid catheter lab/operating room by a “heart team,” including an interventional cardiologist and cardiac surgeon.
36.3 Aortic Valve
Currently, transcatheter aortic valve replacement is considered for high- or extreme-risk patients with severe calcific aortic stenosis; these patients are not considered as appropriate candidates for conventional surgical valve replacements. In general, these procedures have similar benefits as those mentioned above, such as eliminating the need for cardiopulmonary bypass and allowing for shorter patient recovery times. It is conceivable that a transcatheter aortic valve could be delivered by one of four different approaches: (1) transarterially (e.g., via the femoral artery, subclavian artery, or the ascending aorta), (2) transseptally via the right heart, (3) transatrially through the left atrial wall or a port in the atrial appendage, or (4) transapically through the left ventricular wall (Fig. 36.3).
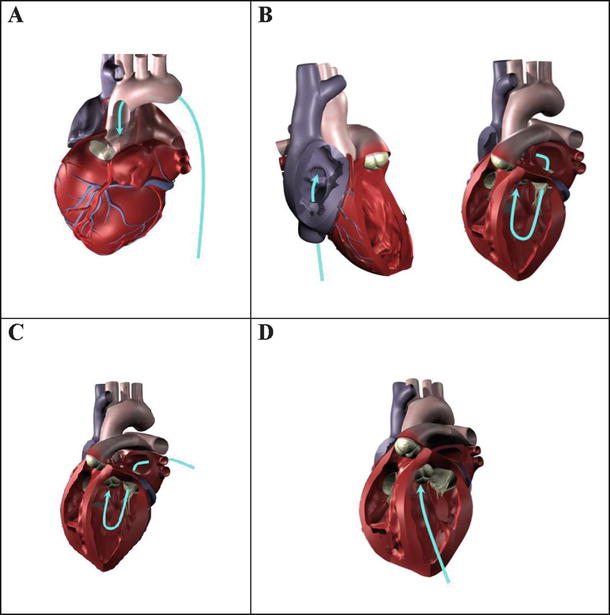
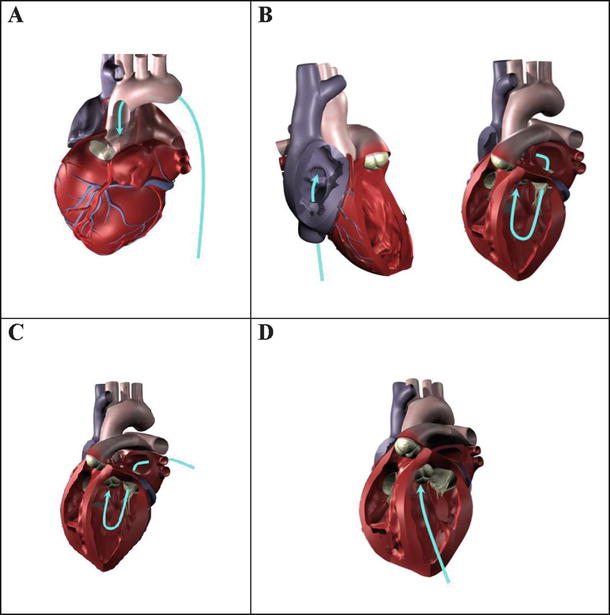
Fig. 36.3
Four potential approaches for the delivery of a transcatheter aortic valve or repair tool: (A) transarterially (e.g., via femoral artery access); (B) transseptally from the right heart (transvenous access) into the left atrium, then through the mitral valve; (C) transatrially through the left atrial wall or through a port in the left atrial appendage, then through the mitral valve; or (D) transapically through the left ventricular wall. The latter two approaches would currently require a minimally invasive surgical procedure
Currently, the transfemoral approach for the delivery of transcatheter aortic valves is the most common access route; this approach is used 69–91 % of the time [9, 10] and is the preferred access route. Primary alternate access routes are transapical (through the apex of the heart in the myocardium), transaortic (through the aorta), and subclavian (subclavian artery) routes. These alternate routes are typically used when the transfemoral approach is deemed not possible due to: (1) the size of the patient’s arteries; (2) the tortuosity of the arteries and/or aorta; and/or (3) the degree of calcification within the aorta, some which could potentially be knocked free by the delivery system. Note that the transfemoral, transaortic, and subclavian routes all require retrograde delivery, while transapical requires an antegrade approach.
In current practice, both the transapical and transaortic approaches require a minimally invasive surgery (see Chap. 35), yet they provide the most direct anatomical approach. The transapical approach must go through the left ventricular wall (myocardium) which can be difficult to close if a large delivery system is used and/or if the myocardial tissue is abnormally frail. The transaortic approach also requires closure of a hole that is placed into the ascending aorta. This can be especially difficult if the aorta is extremely calcified, a condition known as a porcelain aorta . As technology advances, the need for minimally invasive surgery with these approaches may be eliminated. The transseptal approach , which has the advantage of employing transvenous access for the delivery system, has the potential drawback that if the system passing through the interatrial wall is large, the physician may create a septal defect which will require a subsequent repair (see Chap. 37). Additionally, the system must make a turn of approximately 180 degrees in the left ventricle, after passing through the complex structures of the mitral valve, to be positioned in the aortic valve, a maneuver that can be technically challenging. Anatomically, inferior access via a femoral vein is considered advantageous due to the proximity of the inferior vena caval ostium and the fossa ovalis, which is the preferred transseptal puncture location.
It has been reported that rapid ventricular burst pacing can be employed to facilitate transcatheter heart valve implantation. Pacing rates between 150 and 220 bpm with durations of 12 ± 3 s were relatively well tolerated (n = 40) when cautiously used. Rapid pacing was associated with a rapid and effective reduction in systemic blood pressure, pulse pressure, transvascular flow, as well as cardiac and catheter motion [11].
As can be observed in Fig. 36.3, the potential pathways/approaches to place a transcatheter-delivered valve in the aortic position are quite varied and have dramatic differences in the angles and anatomical features that would be required to be navigated within or through, e.g., vessels, chamber walls, valves, or chordae tendineae. Due to this complexity, the flexibility of the delivery system (with the valve loaded inside) will be a major factor to consider when selecting a delivery approach; furthermore, the patient’s cardiac anatomy is a major consideration. This is true for the delivery of the system and also for the positioning of the valve stent into the aortic position, as one must prevent obstruction to the ostia of the coronary arteries.
Currently, there are several transcatheter aortic valves available for clinical use, in clinical trials, or in animal testing. The CoreValve (Medtronic, Inc.) and the SAPIEN XT (Edwards Lifesciences) are FDA approved for high- and extreme-risk patients, and to date, several hundred thousand devices have been implanted in patients worldwide (Fig. 36.4). More specifically, for the transarterial placement of the SAPIEN XT transcatheter heart valve, the surgeon uses the NovaFlex+ delivery system (Edwards Lifesciences), whereas he/she uses the Ascendra+ delivery system (Edwards Lifesciences) for a transapical placement. The CoreValve system is also delivered via a transarterial approach in a specialized delivery catheter. In addition, several other companies that have developed (and continue to develop) competing technologies, including Boston Scientific (Marlborough, MA, USA) and St. Jude Medical (St. Paul, MN, USA) as well as smaller companies such as Direct Flow Medical, Inc. (Santa Rosa, CA, USA) and Heart Leaflet Technologies Inc. (Maple Grove, MN, USA). Nevertheless, nearly all the major players in cardiac valve replacement have a keen interest in these technologies and clinical approaches (Fig. 36.5) [12].
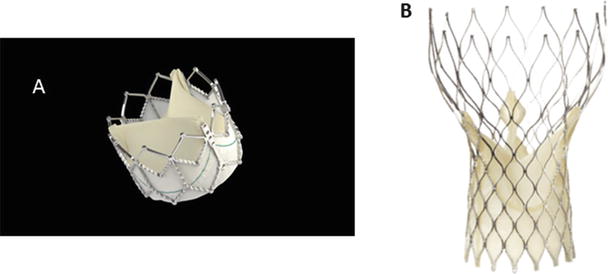
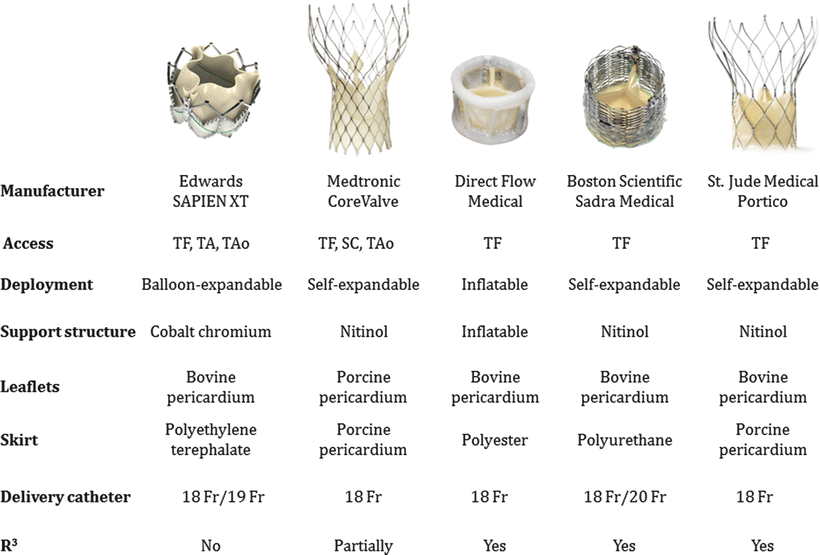
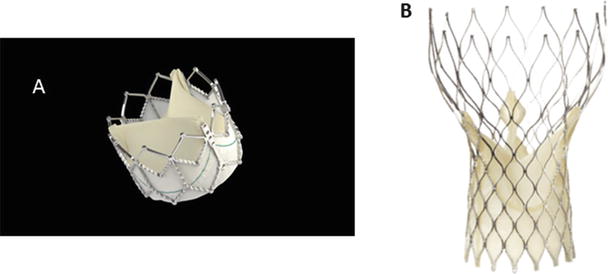
Fig. 36.4
(A) The Edwards Lifesciences SAPIEN XT transcatheter heart valve consists of a cobalt chromium balloon-expandable stent with an attached pericardial valve. It has been designed for transfemoral placement with the NovaFlex+ delivery system or for transapical placement with the Ascendra delivery system. It has been approved for high- and extreme-risk surgical patients in the United States and Europe. (B) The ReValving System by CoreValve, Inc. (Medtronic, Inc.), consists of a self-expandable nitinol stent with an attached pericardial valve. It is designed for transarterial delivery and is currently approved for high- and extreme-risk cases in the United States and Europe
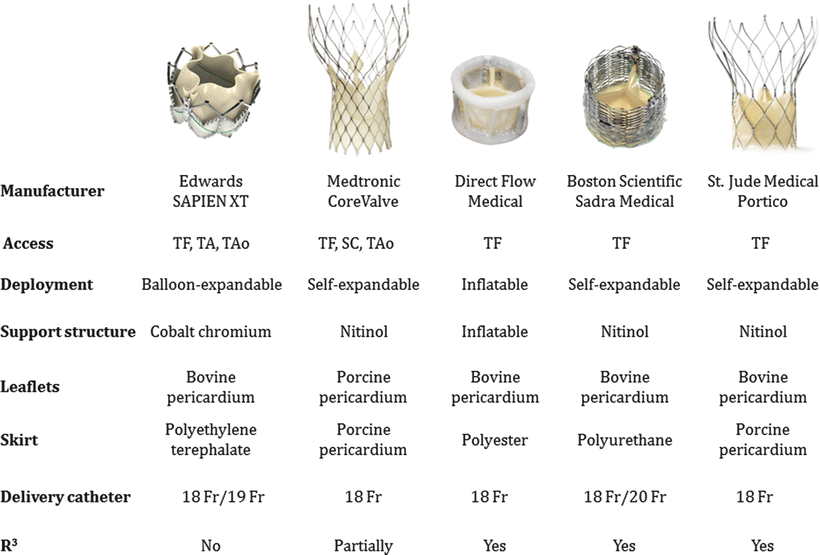
Fig. 36.5
This figure shows a variety of transcatheter aortic valves that are currently working through the regulatory process. It provides an insight into the design and delivery of the valve as well [12]. SC subclavian, TA transapical, TAo transaortic, TF transfemoral. ©2013 Heart Valves: From Design to Clinical Implantation, Transcatheter aortic valve implantation, Piazza N, Mylotte D, Martucci G. With kind permission of Springer Science+Business Media, New York
It is important to note that, currently, a percentage of patients that have received transcatheter-delivered aortic valves have elicited conduction abnormalities which may include heart block. Most of the self-expanding or balloon-expanded valve prostheses exert radial forces on the interventricular septal wall and surrounding structures which is required to maintain proper position and to minimize paravalvular leaks. This force, coupled with the close anatomic proximity of the atrioventricular node, the bundle of His, and/or the left bundle branch with the basal annulus of the aortic valve, is the likely explanation for this phenomenon (Fig. 36.6) (see Chap. 13).
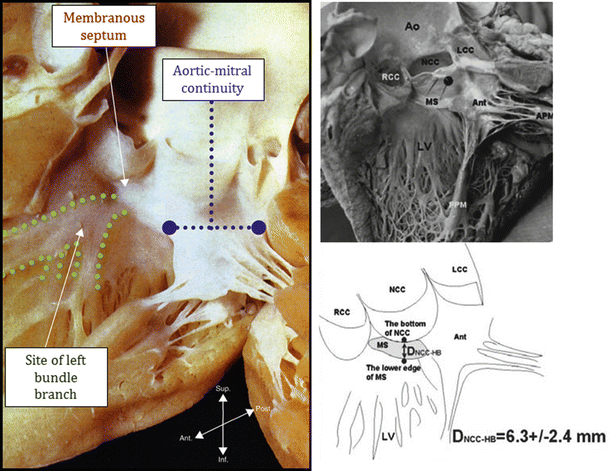
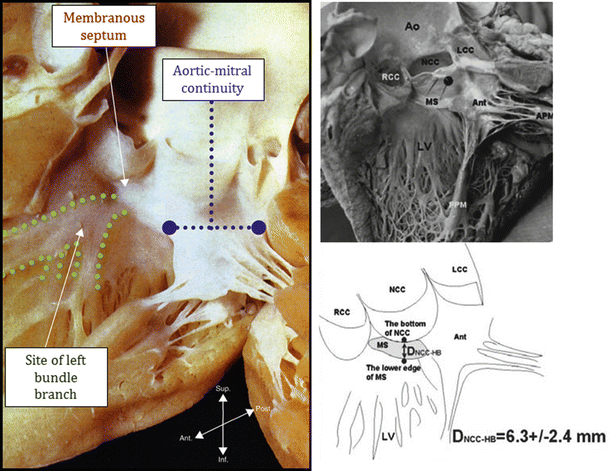
Fig. 36.6
A dissected human heart that shows the proximity of the aortic valve to the left bundle branch. The noncoronary cusp is 6.3 ± 2.4 mm from left bundle branch as depicted in the right picture. It is more common in self-expanding valves to experience issues with the conduction system in the heart [12], ©2013 Heart Valves: From Design to Clinical Implantation, Transcatheter aortic valve implantation, Piazza N, Mylotte D, Martucci G. With kind permission of Springer Science+Business Media, New York
36.4 Mitral Valve
Mitral valve dysfunction can be related to several factors including diseased leaflets [13], annular changes [14], abnormal or damaged chordae [15], and ventricular dilatation [16] causing displacement of the papillary muscles. Due to this large variability in disease process, a wide variety of transcatheter devices are being investigated for the mitral valve. These transcatheter devices can be subdivided into five general types: (1) devices for Alfieri-type edge-to-edge repair, (2) indirect annuloplasty devices deployed into the coronary sinus, (3) direct annuloplasty devices placed on or near the mitral annulus, (4) devices for dimensional control of the left ventricle or left atrium, and (5) devices for mitral valve replacement [17, 18].
The edge-to-edge technique involves placing a stitch to join the anterior and posterior leaflets at the location of regurgitation [19–21]. This technique is most commonly used in patients with A2 or P2 prolapse, and the simplicity of the edge-to-edge technique has led to opportunities for percutaneous valve repair [22–24]. More recently, the MitraClip repair system was designed to use transcatheter clips to grasp the ventricular sides of the anterior and posterior mitral leaflets, leaving the clip in place upon deployment (Abbott Vascular, Bloomington, IN, USA). This technology was FDA approved in 2013.
For patients with annular dilatation, many devices are currently being developed to simulate a traditional annuloplasty procedure. These products are classified as either indirect, which typically involves a transvenous coronary sinus approach , or direct, which involves placing the device in direct contact with the mitral annulus. Percutaneous, transvenous mitral annuloplasty is a technology that implants a metal bar with flexible ends and a stiff midsection to reshape the posterior leaflet; it is a reversible procedure (Viacor, Inc., Wilmington, MA, USA). The Monarc system features two self-expanding stents tethered together which reshape the posterior region of the annulus in 2–3 weeks (Edwards Lifesciences). Similar to the Monarc, Carillon XE (Cardiac Dimensions, Inc., Kirkland, WA, USA) utilizes tethered stents in the coronary sinus to reshape the posterior annulus. These technologies rely on the proximity of the coronary sinus to the posterior aspect of the mitral annulus. Additionally, direct mitral annuloplasty is also being investigated; these devices are in direct contact with the mitral annulus either temporarily or permanently. MiCardia Corporation (Irvine, CA, USA) is developing adjustable annuloplasty devices which are currently implanted surgically but can be adjusted on the beating heart. The Mitralign device (Mitralign, Inc., Tewksbury, MA, USA) places implants around the annulus and then cinches them closer together, thereby reducing the orifice area of the valve. Nevertheless, the goal of these devices is to restore valve coaptation and thus eliminate mitral regurgitation.
Indirect approaches to mitral valve repair that influence left ventricular or left atrial dimensions are also under investigation. By changing left ventricular dimensions, products such as Coapsys and iCoapsys (Myocor® Inc., Maple Grove, MN, USA) are designed to improve mitral valve and left ventricular performance. The PS3 system (Ample Medical, Inc., Foster City, CA, USA) shortens the left atrial dimension between the fossa ovalis and the great cardiac vein and consequently the septal-lateral dimension of the mitral annulus [25].
As described for the aortic valve , it is possible to deploy a transcatheter mitral valve or affect a transcatheter repair using four different approaches: (1) transarterial (e.g., via the femoral artery), (2) transseptal via the right heart, (3) transatrial through the left atrial wall or a port in the atrial appendage, or (4) transapical through the left ventricular wall (Fig. 36.7). The human mitral valve is a very complex, dynamic, and highly variable structure. Therefore, complications in the deployment of a replacement valve or repair device into this position will potentially interact with the (1) valve leaflets themselves, (2) the chordae tendineae, and/or (3) the papillary muscles. As mentioned earlier, the valvular and subvalvular anatomy can be quite variable, with some individuals eliciting bifurcated or trifurcated papillary muscles, distinct chordae tendineae patterns, and numerous scallops of each mitral valve leaflet. Due to the complexity of the mitral valve apparatus and underlying disease processes, it is likely that a combination of the aforementioned devices will be required to provide percutaneous solutions for mitral valve repair.
< div class='tao-gold-member'>
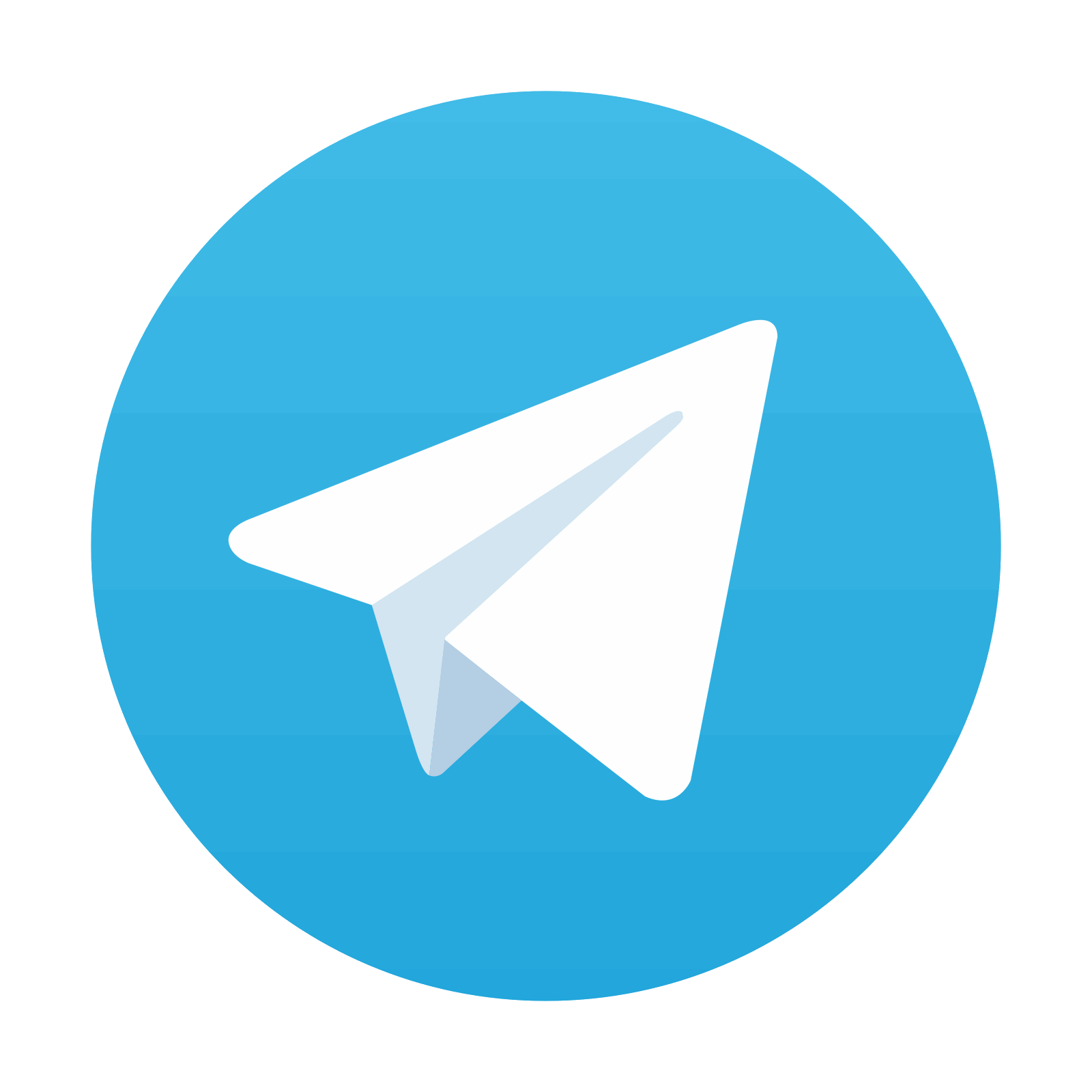
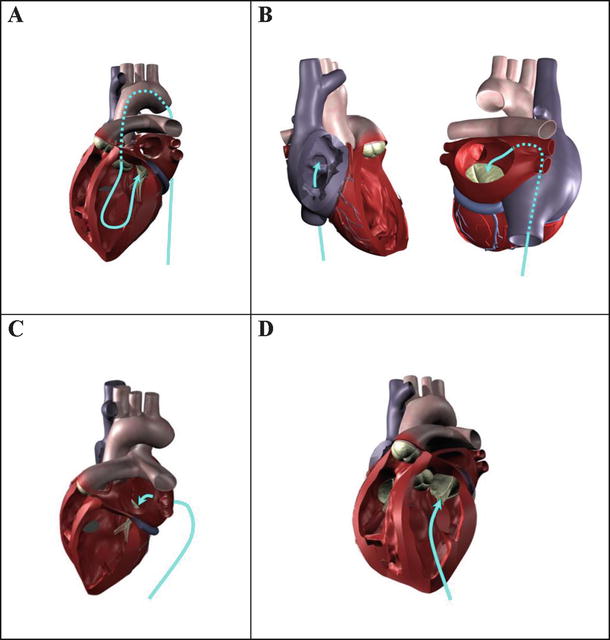
Only gold members can continue reading. Log In or Register a > to continue
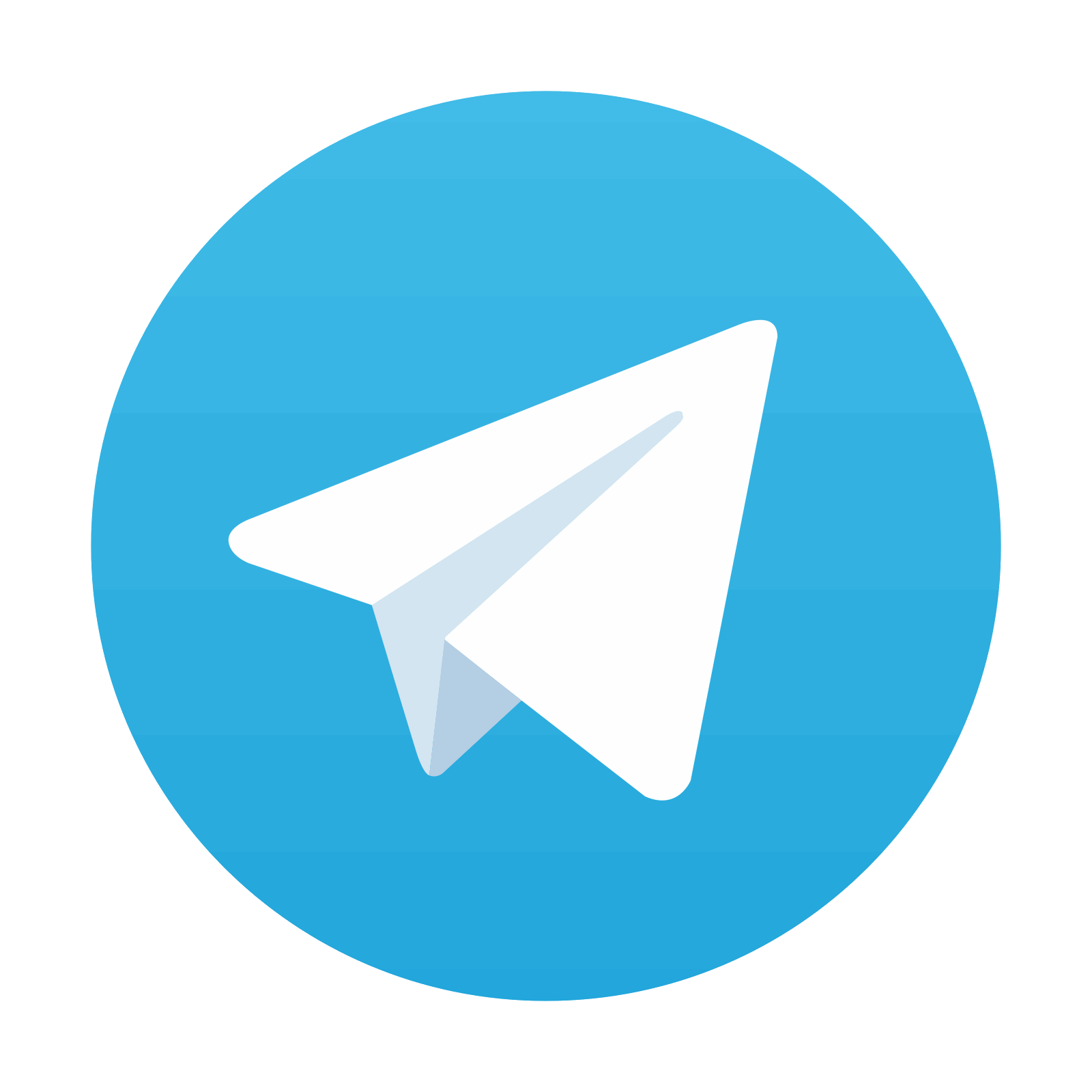
Stay updated, free articles. Join our Telegram channel

Full access? Get Clinical Tree
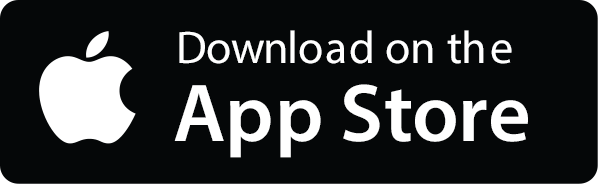
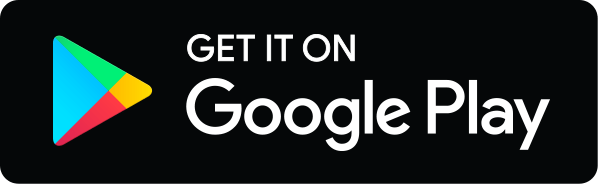