CHAPTER 98 Total Artificial Heart
HISTORY
Landmark Cases
Long sequences of ideas and inventions from many individuals and many disciplines have made bridge to transplantation with a total artificial heart (TAH) possible. In 1953, Gibbon performed the first open heart surgery with the use of an extracorporeal cardiopulmonary bypass and cardiac arrest.1 In 1957, at the Cleveland Clinic, Akutsu and Kolff successfully implanted a TAH in a dog that survived for 90 minutes.2 Using a bovine model in 1963, survival rates of greater than 30 hours were documented. Calves became the experimental model of choice because of better tolerance of cardiopulmonary bypass and less thrombogenicity. These experiments led to testimony in 1963 before Congress that allowed the development of a permanent TAH. In 1964, the National Heart Initiative instituted an order to produce a TAH.
In 1969, Dr. Denton Cooley was the first to implant a TAH, the Liotta TAH, in a human as a bridge to transplant (Table 98-1).3 The patient was supported for 64 hours on the device and then died of pneumonia and sepsis 32 hours after transplantation. The next TAH implantation was in 1981, again by Dr. Cooley.4 A 36-year-old man with cardiac failure after coronary artery bypass grafting was implanted with the Akutsu TAH for 53 hours until transplantation. He died 8 days after transplantation, from sepsis. In 1981, Drs. DeVries, Kolff, and Jarvik received U.S. Food and Drug Administration (FDA) approval to permanently implant the Jarvik-7 TAH at the University of Utah. In 1982, their first recipient was Dr. Barney Clark, who lived for 112 days.5
Table 98–1 Total Artificial Heart World Experience18
Year of First Implant | Type of Device | Implants (N) |
---|---|---|
1969 | Liotta3 | 1 |
1981 | Akutsu19 | 1 |
!982 | Jarvik-7 (100 mL)5 | 44 |
1985 | Phoenix6 | 1 |
1985 | Penn State20 | 4 |
1985 | Jarvik-7 (70 mL)6 | 159 |
1986 | Berlin21 | 7 |
1986 | Unger22 | 4 |
1987 | Poisk23 | 16 |
1988 | Brno24 | 6 |
1989 | Vienna25 | 2 |
1993 | CardioWest6 | 534 |
1998 | Phoenix-726 | 2 |
2001 | AbioCor9 | 14 |
In 1985, Dr. Jack Copeland of the University of Arizona, attempting to save a patient who had cardiac graft failure, implanted the unapproved Phoenix TAH as a bridge to transplant. The use of an unapproved heart caused controversy, but it resulted in acceptance by the FDA of the one-time use of unapproved devices in true emergencies. This patient died of sepsis after a second transplant. On August 29, 1985, Copeland and his team successfully bridged a young man to transplantation after 9 days of TAH support with a Jarvik-7-100 (with a stroke volume of 100 mL).6 This patient survived for more than 5 years, eventually dying of post-transplantation lymphoproliferative disease. This initial success was followed by other successful implants of the 100-mL device before the introduction in 1986 of a smaller device that fit most patients and had a stroke volume of 70 mL, the Jarvik-7-70.
At that time, little was known about prevention of the two major complications of mechanical circulatory support devices, thromboembolism and infection. In 1990, the FDA, realizing that critical follow-up information was not being provided by Symbion, the manufacturer of the Jarvik-7, shut down the investigational device exemption (IDE) study being conducted in the United States. At about the same time, a promising study was released from La Pitié Hospital in Paris7 which reported no neurologic complications in 60 consecutive Jarvik-7 patients with the use of a multidrug anticoagulation protocol.
In 2004, the CardioWest TAH-t (“t” indicating that the device was approved for temporary use as a bridge to transplantation) was the first and only TAH to be approved by the FDA for use as a bridge to transplant, as reported in the New England Journal of Medicine in 2004.8 In international multi-institutional experience, the CardioWest TAH has proved to be an excellent surgical therapy for patients who would otherwise die while awaiting a heart transplant.
Comparison between AbioCor Implantable Replacement Heart and CardioWest TAH-t
The CardioWest TAH-t could be used as a long-term or “permanent” device, but it has not been since the time of DeVries’ four cases and Dr. Semb’s one case in Sweden. The AbioCor (Fig. 98-1) was designed as a permanent artificial heart for patients who are not candidates for any conventional operative therapy or for heart transplantation.
There was one long-term AbioCor survivor out of hospital. Problems with this device have included its large size, its weight, a high incidence of thromboembolism, limited durability, and extremely high negative pressures in the atria (−40 mm Hg). These negative pressures have caused air embolism at the time of implantation and led to a new abnormal physiology called atrial suck-down, in which the walls of the atria are literally sucked into the atrioventricular (AV) valves, causing obstruction and embolism. The device is approved for humanitarian use by the FDA, but there have been no implants since 2004.9
The current console is large and mobile within a medical facility. The console, of which there are only 38 in the world, is made up of two pneumatic drives, transport batteries, air tanks, and an alarm and monitoring system. Once the beat rate, “percent systole” (percentage of the cardiac cycle occupied by systole), left and right driving pressures, and vacuum are set manually, it is rare to need to change them. Each artificial ventricle primary driver is set for full ejection of blood. To achieve full ejection, the right ventricle is set to 30 mm Hg greater than the pulmonary artery pressure, and the systemic drive pressure is set to 60 mm Hg higher than the systemic pressure. The console controls are set to fill the ventricles to approximately 50 to 60 mL per beat. This allows the possibility of an increased venous return of 10 to 20 mL (total of 70 mL) in circumstances such as exercise with increased venous return and consequent increase in stroke volume and cardiac output. Thus, the artificial heart, like the native heart, follows the Starling principle (Fig. 98-2).
Two new and much smaller portable consoles exist: the Companion I and Freedom (Fig. 98-3). The Companion I weighs 40 pounds and can be pulled like a suitcase on a modular caddie. It can also be plugged into a hospital console with a bigger screen and a more stable outer structure. The Companion I will also drive biventricular assist devices (BiVADs), univentricular VADs, and an intra-aortic balloon pump. It is scheduled for comparative studies with the large console starting in the third quarter of 2009. The plan is to completely replace all large consoles with the Companion I and to have hundreds of these consoles available worldwide by 2010. The Freedom is the size of a coffee tin and weighs 4 pounds. It contains two drivers, one for continuous use and one for backup. Clinical testing for this very portable driver is scheduled for 2009.
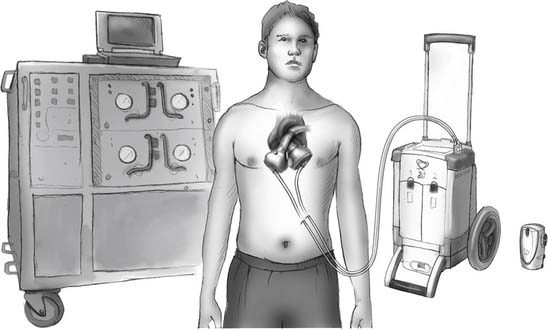
Figure 98–3 CardioWest drivers showing from left to right: the current driver, the Companion I, and the Freedom.
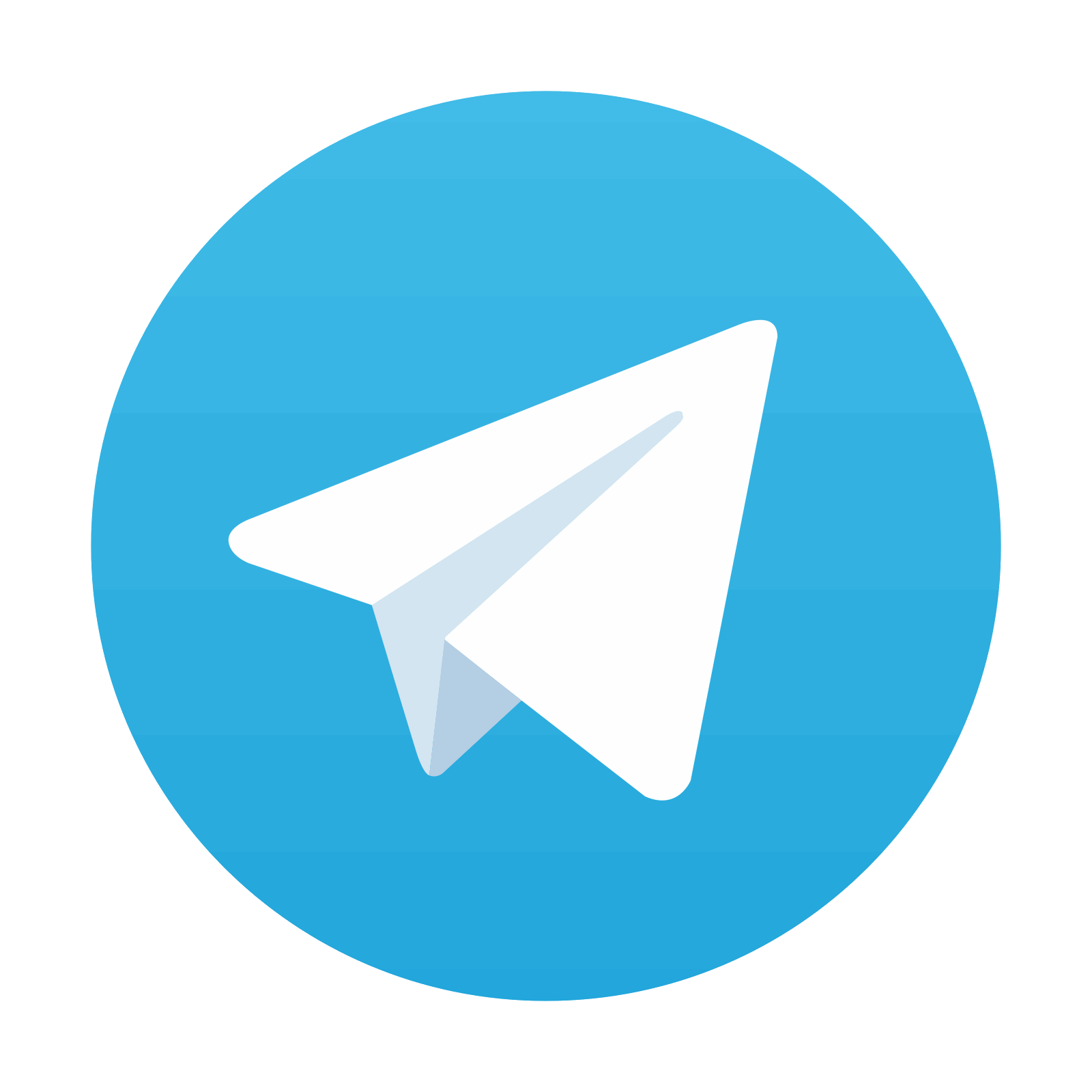
Stay updated, free articles. Join our Telegram channel

Full access? Get Clinical Tree
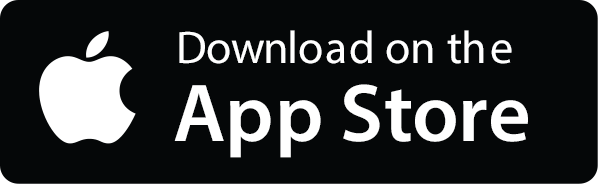
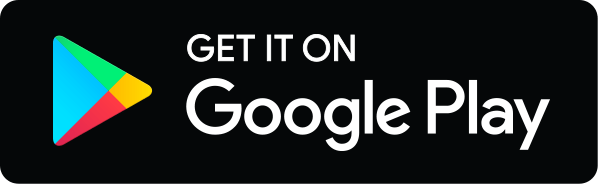