Rachel D. Vanderlaan and Christopher A. Caldarone Hospital for Sick Children, University of Toronto, Toronto, Ontario, Canada Total anomalous pulmonary venous connection (TAPVC) constitutes a heterogeneous group of lesions in which all of the pulmonary veins are connected to the right atrium or its systemic venous tributaries. A concomitant right‐to‐left shunt, commonly via an interatrial communication, is required for survival after birth. Significant obstruction to pulmonary venous drainage results in pulmonary edema and cardiogenic shock, which is rapidly lethal if untreated. Obstructed TAPVC is one of the few true surgical emergencies in congenital heart disease. Surgical results for the treatment of TAPVC have greatly improved over the last three decades, reducing operative mortality to less than 10% in most centers. Strategies at the initial operation (sutureless repair) to prevent the need for reoperation secondary to pulmonary vein stenosis are ongoing. Successful connection between the pulmonary venous system and the left atrium is the result of three distinct processes that involve the formation of the primitive lung and the primitive left atrium. At first, primitive lung buds originate as outpouchings from the trachea on day 26–27 of gestation. A plexus of vessels, the splanchnic plexus, develops around the lung buds and allows the drainage of blood into the anterior cardinal veins, which will become the primordial superior caval vein (SVC), coronary sinus, and azygous vein, and into the umbilicovitelline veins, which develop into the portal system. Various hypotheses have been proposed for pulmonary vein development. It remains unclear whether or not the pulmonary vein is derived from the systemic venous sinus [1, 2]. All agree that the mid‐pharyngeal strand canalizes to become the pulmonary vein [3]. The canalizing strand then uses the dorsal mesocardium to gain entrance to the developing left atrium. The pulmonary veins themselves are formed within the pulmonary parenchyma and eventually lose their connection with the splanchnic venous plexus. The pulmonary veins incorporate into the roof of the left atrium after the completion of ventricular septation. It is only subsequent to the completion of septation that it becomes possible to recognize the superior interatrial fold. This fold is lacking in the setting of TAPVC [4]. Failure of all the pulmonary veins to divide from the splanchnic venous system is hypothesized to be the primary defect in embryological development responsible for TAPVC [3–7]. Therefore, supracardiac TAPVC results from failure of the pulmonary vein to separate from the right cardinal venous system, cardiac TAPVC is failure to separate from the left cardinal venous system, and infracardiac TAPVC represents failure of the pulmonary vein to separate from the umbilicovitelline system. Unilateral lesions produced at the same stage (partial anomalous pulmonary venous connection), lesions occurring at a later stage (atresia of common pulmonary vein), or lesions as a result of abnormal reabsorption of the common vein into the left atrium (congenital pulmonary vein stenosis and an abnormal number of pulmonary veins) are important related pulmonary venous anomalies. TAPVC is a rare malformation that accounts for approximately 1.5% of all cases of congenital heart disease [8]. Typically, anomalous pulmonary venous connection occurs in sporadic isolated cases and, similar to most congenital heart defects, the etiology is likely multifactorial, with a complex interplay between environmental and genetic factors. TAPVC can present as an isolated malformation, but it can also be associated with syndromes such as cat‐eye, right atrial isomerism, and Holt‐Oram [8]. There are familial nonsyndromic cases reported in the literature that support an autosomal dominant pattern of inheritance with variable penetrance and expression. An analysis of a large Scottish family identified a TAPVC‐1 region found on chromosome 4q12, with candidate genes KDR and PDGFRA implicated in the development of TAPVC [9, 10], In another study, ANKRD1 overexpression was associated with TAPVC in a family with two affected siblings [11]. Alterations in Semaphorin 3d and retinoic acid signaling also have been associated with TAPVC in animal genetic models and a corresponding point mutation in Semaphorin 3d was discovered in an individual with partial anomalous pulmonary vein connection [12, 13]. Further large‐cohort studies are required to understand the genetic contributions to anomalous pulmonary vein development. Figure 35.1 Examples of the more common forms of TAPVC. (A) Supracardiac connection with drainage of the common pulmonary vein (CPV) through a vertical vein (VV) into the left innominate vein (LIV). (B) Cardiac‐level connection to the coronary sinus (CS). (C) Infracardiac connection of the common pulmonary vein to the portal venous (PV) system by a descending vertical vein. DV, ductus venosus; HV, hepatic vein; IVC, inferior caval vein; LA, left atrium; LV, left ventricle; RA, right atrium; RV, right ventricle; SVC, superior caval vein. Source: Mavroudis C, Backer CL 2003 / with permission of Elsevier. TAPVC classification is based on the level of connection of the anomalous connecting vein and the degree of obstruction [14]. The Darling classification, based on autopsy examination, has become the standard classification scheme [6, 15]. In type 1, or supracardiac, the common vein connects to structures above the heart (right SVC, azygos vein, left SVC, or innominate vein). In type 2, or cardiac, the connecting vein is attached to cardiac structures (e.g., the right atrium or the coronary sinus). In type 3, or infracardiac, the connection is situated below the diaphragm to the inferior caval vein (IVC)/portal vein system. Type 4, mixed, represents a combination of these conditions (Figure 35.1). TAPVC is also stratified by the presence/absence of obstruction to pulmonary venous drainage [16, 17]. Obstructions can be found at the level of the connecting vein, due to intrinsic narrowing or extrinsic compression, or at the level of the interatrial communication (Table 35.1). The presence/absence of obstruction should be mentioned and the nature and level of obstruction should be added as the last element to complete the diagnosis (Table 35.2) [5]. Table 35.1 Mechanisms of obstruction in total anomalous pulmonary venous connection (TAPVC). Table 35.2 Congenital Heart Surgery Nomenclature and Database Project: total anomalous pulmonary venous connection (TAPVC). A communication between the systemic and the pulmonary venous return is necessary for survival after birth. In the overwhelming majority of cases this is ensured by an interatrial communication. Rarely, the communication can be one or multiple ventricular septal defects or a patent arterial duct [18, 19]. Atrial‐level shunts are typically of adequate size and the interatrial gradient is usually low [20, 21]. The absent connection of the pulmonary vein to the left atrium results in an absent myocardial sleeve surrounding the pulmonary veins and also failure of complete development of the inner smooth muscle cell layer in the left atrium [22]. Another consequence is a small left atrium body, due to failure of incorporation of the pulmonary veins into the body of the left atrium [23]. The right atrium can be thick and enlarged. In most cases, the left ventricle has normal size and muscular mass [24, 25]. On the contrary, the right ventricle can be enlarged and hypertrophied, especially in supradiaphragmatic nonobstructive lesions [26]. TAPVC by definition is always present in the setting of right atrial isomerism, since the pulmonary veins must connect anomalously in the presence of bilateral right atrial appendages. Tetralogy of Fallot, double‐outlet right ventricle, and interrupted aortic arch have also been reported as associated lesions [18, 27–29]. The clinical presentation of TAPVC depends on two critical elements: the degree of obstruction to the pulmonary venous drainage and the degree of obstruction to the atrial‐level right‐to‐left shunt. Left untreated, the mortality of TAPVC is greater than 80% [16]. At one end of the spectrum, there are neonates with completely unobstructed circulation (typically supracardiac and cardiac types). These patients present with signs and symptoms of a large left‐to‐right shunt. Tachypnea and arterial desaturation are present to various degrees, together with signs of right ventricular volume overload. If untreated, this condition can lead to congestive heart failure. Late sequelae include pulmonary edema, pulmonary hypertension, and cyanosis. At the other end of the neonatal clinical spectrum are neonates with severe pulmonary venous flow obstruction. In these cases, the resulting pulmonary venous and pulmonary arterial hypertension produces severe acute pulmonary edema, right ventricular antegrade flow reduction due to the excessive increase in afterload, extreme hypoxemia, and circulatory collapse. The presence of a restrictive atrial septal defect results in reduced preload to the left ventricle, diminished systemic cardiac output, and severe cardiogenic shock. Obstructed TAPVC is rapidly lethal and presents formidable challenges to treatment. Unobstructed TAPVC is sometimes difficult to diagnose in the first few days of life because patients may have very mild cyanosis and the absence of a murmur. After the first few months of life, infants may present with tachypnea, mild cyanosis, failure to thrive, and feeding difficulties. On examination, a loud and continuously split S2 together with a systolic ejection murmur over the pulmonary valve are common findings. Hepatomegaly, venous congestion, and fine crackles on lung auscultation are possible in cases of noncompensated cardiac failure. Neonates with an obstructed circulation become critically ill immediately after birth. In those cases hypoxia and profound cyanosis, hypotension, and metabolic acidosis are commonly encountered [29, 30]. Figure 35.2 Preoperative computed tomography (CT) images of a 2‐month‐old with total anomalous pulmonary venous connection to the coronary sinus. (A) Coronal reformation. (B) Volume‐rendered posterior image. Courtesy of Dr. Cynthia K. Rigsby. Chest radiographs of unobstructed TAPVC show plethoric lungs and a large cardiac silhouette. A typical finding is the so‐called snowman silhouette, where the heart and the mediastinal enlargement due to prominent pulmonary artery, vertical vein, and SVC form a “figure‐of‐8.” The snowman sign is characteristic of supracardiac TAPVC, but is generally not appreciable before the age of 6 months [31]. Obstructed neonatal TAPVC shows severe pulmonary edema in the presence of a normal size and shape of the heart. Two‐dimensional and Doppler echocardiography are the primary diagnostic tools for most patients. Diagnostic findings are a distended right ventricle, a vascular confluence coupled with absent venous drainage to the left atrium on the Doppler interrogation, a dilated coronary sinus, and turbulent flow in the right atrium with a right‐to‐left shunt. Echocardiography has shown excellent sensitivity and specificity in fetal diagnosis and as a prognostic tool [32–34]. In general, echocardiography has supplanted angiography in the vast majority of cases [30, 31, 35–37]. Cardiac catheterization is avoided in unstable neonates to reduce the delay to treatment and contrast‐related renal injury. It can be useful in nonobstructive cases to better define connections of mixed type and to identify possible associated anomalies. The site of the anomalous connection can be precisely located with a step‐up in oxygen saturation along the systemic vein system. Intracardiac oxygen saturation measurements characteristically demonstrate equivalent saturations in all cardiac chambers, the pulmonary artery, and the aorta. In nonemergency cases of TAPVC, contrast‐enhanced magnetic resonance angiography (MRA) or computed tomography (CTA) can be used to provide a complete anatomic diagnosis, with MRA providing additional functional information [38]. From an anatomic point of view, CTA and MRA offer superior visualization of all pulmonary veins and other pulmonary‐to‐systemic venous connections, compared to echocardiography (Figure 35.2) [39]. From a functional point of view, the amount of anomalous drainage and the resulting Qp/Qs can be calculated by using phase‐contrast MRA, which depicts the velocity, volume, and pattern of pulmonary venous blood flow. Moreover, a description of the secondary effects on pulmonary arterial size and blood flow, ventricular size, and function are obtainable in the same study. This important information is difficult or impossible to obtain using other modalities [38, 40, 41]. Despite the significant differences in physiology and clinical presentation, both obstructed and nonobstructed TAPVC pose an absolute indication for surgical repair, given the poor prognosis recorded in untreated cases. In patients with evidence of pulmonary venous obstruction, surgical repair is conducted promptly after diagnosis [42, 43]. In our institution, patients with unobstructed TAPVC are electively repaired at 2–4 months of age. Alternatively, other programs choose to repair unobstructed TAPVC at the time of presentation during the neonatal period. Medical management of patients with nonobstructed pulmonary venous connection is directed at compensating right ventricular failure, hypoxia, and congestive heart failure with mild inotropic support, diuresis, and avoidance of high levels of inspired oxygen. Assisted ventilation is rarely needed. Medical maneuvers in patients with obstructed TAPVC are limited in terms of efficacy. Neonates with obstructed TAPVC often require intubation, 100% oxygen, and hyperventilation to maintain PaCO2 below 30 mmHg (promoting respiratory alkalosis). Sodium bicarbonate is used to correct metabolic acidosis and enhance the action of inotropic and diuretic treatments, which is often necessary to support the failing ventricles. Prostaglandin E1 can be infused to maintain patency of the arterial duct, which has the effect of improving systemic cardiac output through a right‐to‐left shunt, although this often occurs at the expense of an already reduced pulmonary circulation in conditions of severe hypoxia. Medical efforts are minimally effective in correcting the ensuing hemodynamic and metabolic crisis and their use is limited to providing some short‐lived palliation until definitive surgical repair is accomplished. In some centers cardiac catheterization has been used in critically ill patients for diagnosis and percutaneous palliation of obstructed TAPVC. A restrictive interatrial communication can be relieved with balloon septostomy. Moreover, case reports have documented the use of percutaneous angioplasty and stenting of the obstructed common vein to palliate shock and improve preoperative optimization of the metabolic state (Figures 35.3 and 35.4) [44, 45]. Repeated stent implantation for in‐stent restenosis and the use of extracorporeal membrane oxygenation (ECMO) to stabilize the patient during the procedure has been reported [46, 47]. These initial experiences have yielded promising results in obtaining hemodynamic stabilization in preparation for surgery. Figure 35.3 Total anomalous pulmonary venous connection of supracardiac type to the innominate vein. (A) Both right and left pulmonary veins are unobstructed. The vertical vein, however, is severely stenotic at the junction with the innominate vein. (B) Emergency percutaneous stent dilation was performed with acceptable short‐term result. Courtesy of Dr. Shi‐Joon Yoo and Mr. Omar Thabit. Source: Reproduced by permission from Viola N, Caldarone CA. In: Mavroudis C, Backer CL (eds.), Pediatric Cardiac Surgery, 4th ed. Oxford: Wiley‐Blackwell; 2013, pp. 659–673. Figure 35.4 Infracardiac total anomalous pulmonary venous connection. The vertical vein is stenosed at the insertion in the venous duct (arrow). Courtesy of Dr. Shi‐Joon Yoo and Mr. Omar Thabit. Source: Reproduced by permission from Viola N, Caldarone CA. In: Mavroudis C, Backer CL (eds.), Pediatric Cardiac Surgery, 4th ed. Oxford: Wiley‐Blackwell; 2013, pp. 659–673. Some centers have reported good results using ECMO in the critically ill neonate as preparation for surgery [48, 49]. Postoperative ECMO is used in patients with severe residual pulmonary hypertension [50, 51]. Once in the operating room, the anesthetic management is directed toward reducing the pulmonary vascular resistance by the use of fentanyl in high doses, and aggressive correction of hypocalcemia, hypoglycemia, and lactic acidosis. Isoproterenol may be useful in normotensive patients for afterload reduction. Inhaled nitric oxide will also decrease the pulmonary vascular resistance. Surgical repair of supracardiac TAPVC is accomplished via sternotomy with two venous cannulae (IVC and SVC) and an aortic cannula. Immediately after the initiation of cardiopulmonary bypass, the arterial duct is ligated and divided. The patient is cooled to mild hypothermia at 28–30 °C or, rarely, deep hypothermia at 18–20 °C to allow deep hypothermic circulatory arrest. The aorta is cross‐clamped and cardiac arrest induced with antegrade cold‐blood cardioplegic solution. The heart is retracted cephalad and to the right, exposing the left posterior pericardial area [52]. This maneuver is enhanced by the absent connection between the pulmonary veins and the atrium. The vertical communicating vein is ligated near its connection with the innominate vein or SVC, to avoid tethering of the vein ostia near the site of planned anastomosis. When severe preoperative obstruction is present, leaving the vertical vein patent may contribute to the relief of postoperative pulmonary vein hypertension [53], although significant residual left‐to‐right shunt may require reintervention [54]. A longitudinal incision is made in the pulmonary vein confluence and the posterior wall of the left atrium. Because collateral pulmonary flow can be extensive, cardiopulmonary bypass flow rates may need to be decreased temporarily to facilitate visualization and placement of the incision. Small cardiotomy suction cannulas can then be placed in the pulmonary venous confluence and perfusion flow rates restored. Through the left atriotomy, the interatrial communication is closed either primarily or with a patch of autologous pericardium. The left atrium and the pulmonary vein confluence are then anastomosed to create the largest possible anastomosis. Some surgeons advocate the use of interrupted sutures, others the use of absorbable sutures, with inconclusive data to support either technique. The editors have used running absorbable sutures [55]. Attention must be paid to maintain adequate patency of the anastomosis without distortion of the ostium of the pulmonary veins (Figure 35.5). This approach, which involves a substantial displacement of the heart anteriorly and to the right, carries the potential for distortion of the atrium and confluence at the time of the repair and consequent postoperative stenosis. In order to avoid this problem, some centers advocate performing the posterior anastomosis across the roof of the left atrium between the SVC and the aortic root [56], so that the reciprocal position of the two chambers is left unchanged during the repair.
CHAPTER 35
Total Anomalous Pulmonary Venous Connection
Embryology
TAPVC and Genetics
Classification
Intrinsic
stenosis
Stenosis of the individual pulmonary veins
Stenosis of the confluence
Stenosis of the connecting vein (single or multiple)
Stenosis at the site of the abnormal connection drainage into a solid parenchymal organ
(infracardiac type)
Extrinsic
compression
Anatomical “vice” position (supracardiac type)
Between bronchus and pulmonary artery
Connecting vein anterior to aortic arch
(supracardiac type)
Mixed
compression
Restrictive
interatrial
communication
Small esophageal hiatus (infracardiac type)
Combination of intrinsic and extrinsic
mechanisms
Hierarchy level 1 Pulmonary vein anomaly
Hierarchy level 2 Darling’s type
Hierarchy level 3 Obstruction
Hierarchy level 4 Type of obstruction
TAPVC
Type 1 (Supracardiac)
Nonobstructed
Obstructed
Intrinsic narrowing
Extrinsic compression
Obstructive interatrial septum
Type 2 (Cardiac)
Nonobstructed
Obstructed
Intrinsic narrowing
Extrinsic compression
Obstructive interatrial septum
Type 3 (Infracardiac)
Nonobstructed
Obstructed
Intrinsic narrowing
Extrinsic compression
Obstructive interatrial septum
Type 4 (Mixed)
Nonobstructed
Obstructed
Intrinsic narrowing
Extrinsic compression
Obstructive interatrial septum
Cardiac Anatomy, Associated Lesions
Natural History
Diagnosis
Operative Management and Anesthetic Considerations
Surgical Repair of Supracardiac Type
Stay updated, free articles. Join our Telegram channel

Full access? Get Clinical Tree
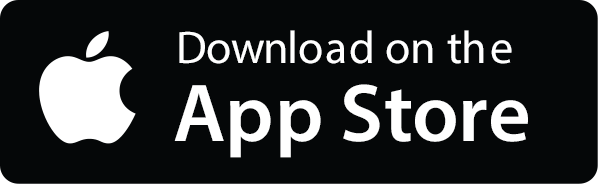
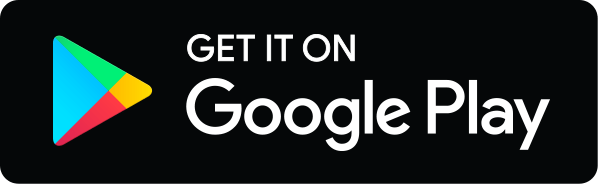