Tom R. Karl1 and Jeffrey P. Jacobs2 1University of Queensland, Brisbane, Australia 2University of Florida, Gainesville, FL, USA Congenitally corrected transposition of the great arteries (ccTGA) has attracted a great deal of interest and some controversy over the years. The malformation is a complex one, featuring reversed (discordant) atrioventricular (AV) and ventriculo‐arterial (VA) connections. Alternate terms to describe ccTGA include corrected transposition, discordant transposition, AV and VA discordance, physiologically corrected transposition, double discordance, AV discordance with transposition, L‐transposition (L‐TGA), inverted transposition, and others. Standard segmental nomenclature has also been applied. A full review of the nomenclature and a proposed classification have been adopted for the Society of Thoracic Surgeons (STS) Database for Congenital Heart Surgery, and ccTGA has become a universally understood term, even if “corrected” is an overstatement [1]. Single or unbalanced ventricular variants with an L‐loop embryology but without a discordant AV connection are excluded from this discussion. Although the nomenclature is now well accepted, the relative rarity (approximately 1/33,000 live births, or approximately 0.05% of congenital heart malformations) and complexity of ccTGA have impeded our ability to establish a universally acceptable treatment strategy. The morphologic features common to hearts with ccTGA are as follows: As a result of discordant connections at both levels, blood flows in a “congenitally corrected” pathway, despite some rather extreme anatomic derangements (Figure 26.2). The “correction” is physiologic only, in the sense that in biventricular hearts with ccTGA a normal oxyhemoglobin saturation may be present (in cases without intracardiac shunts and LV outflow tract obstruction). From a segmental viewpoint, the most common configuration is atrial situs solitus with left ventricular loop and left TGA {S,L,L}. Less common is ccTGA with atrial situs inversus {I,D,D}. Discordance between the atrial situs and the position of the cardiac apex (i.e., ventricular apex points toward caval axis) is frequent in ccTGA (as compared to concordant TGA). Additional anomalies, albeit of varying severity, are present in 99% of cases of ccTGA (Figure 26.3). The three most commonly associated major anomalies in ccTGA are ventricular septal defect (VSD), pulmonary valve and/or subpulmonary stenosis (resulting in LV outflow tract obstruction), and TV malformations (resulting in systemic AV valve insufficiency). Although ccTGA has been reported to occur with AV septal defect, a common AV valve creates some ambiguity in applying a strict anatomic definition of AV discordance [2]. Associated abnormalities in ccTGA may affect the timing and mode of presentation, the severity of symptoms, the clinical evolution, and, ultimately, the approach to management. Figure 26.1 (A, B) Anatomy of congenitally corrected transposition of the great arteries (ccTGA). In this {S,L,L} heart, the aorta (Ao) arises from a left‐sided anatomic right ventricle (RV) and the pulmonary artery (PA) from a right‐sided anatomic left ventricle (LV), with the respective atrioventricular valves. In this case a large ventricular septal defect (VSD) is also present. The epicardial coronary branching pattern is 1R, 2LCx. MV, mitral valve; PV, pulmonary valve; RA, right atrium; TV, tricuspid valve. Source: Reproduced by permission from Karl TR, Cochrane AD. In: Pediatric Cardiac Surgery, 3rd ed., St. Louis, MO: Mosby, 2003, pp. 476–495. In ccTGA there is an anterior–superior location of the AV conduction axis, which is vulnerable to spontaneous or surgically induced heart block (Figure 26.4). Rarely, AV conduction tissue may reside in a more normal postero‐inferior position in ccTGA hearts with LV outflow tract obstruction [2, 3]. The LV outflow tract in ccTGA is deeply wedged between the TV and mitral valve (MV), creating a variable tendency to dynamic or fixed LV outflow tract obstruction in 30–50% of cases [4]. The presence of LV outflow tract obstruction exerts a major influence on the choice of surgical strategy. Coronary anomalies are also common in ccTGA, and may likewise complicate the surgical approach. In general, the coronary arteries arise from facing sinuses in a mirror‐image arrangement (1R, 2LCx by the Leiden classification) [5]. In an anatomic specimen study from Children’s Hospital of Philadelphia, 45% of ccTGA hearts had coronary artery abnormalities that might add complexity to anatomic surgical repairs [6, 7]. In the initial Melbourne double‐switch experience, although 12/14 patients had a 1R, 2LCx pattern, 2/14 had a single coronary artery from a nonfacing sinus (a pattern not seen in the first 400 cases undergoing arterial switch operation for concordant TGA) (Figure 26.5) [6, 7]. The etiology of ccTGA is unknown, and there is no phylogenetic or ontogenetic precedent, as in some other forms of congenital heart disease. There is probably a link with abnormalities of visceral situs. Transposition of the great arteries (TGA), ccTGA, and heterotaxy can all be induced in a murine model by treatment with retinoic acid or inhibitors thereof [8]. It would appear that ccTGA is more likely to be a laterality defect rather than a conotruncal defect per se. Although primacy is always difficult to prove in a discipline of active minds, von Rokitansky (1875) was almost certainly the first to describe the pathologic entity now known as ccTGA [9]. Monckeberg (1913) noted the important anterior AV node position, and Walmsley (1931) described the mirror‐image coronary arterial branching pattern and the position of the central fibrous body [10, 11]. Schiebler and associates (1961) promoted the “ccTGA” terminology [12]. Anderson and Lillehei (1957) performed and documented the first (physiologic) surgical repairs [13]. Imai and colleagues (1989), and Mee and coworkers (1989), independently described various anatomic repair strategies, including what is now known as the double‐switch operation [14, 15]. Ilbawi (1990) described the Rastelli + atrial baffle approach to ccTGA with VSD and LV outflow tract obstruction [16]. The 1.5‐ventricle approach using a bidirectional cavopulmonary shunt (BCPS) (and subsequently incorporated into both physiologic and anatomic repairs) was first proposed by Mavroudis and Backer (1999) [17]. Aortic root and pulmonary root translocation in ccTGA have been described independently by multiple groups over the past two decades, based in part on the principles of the Nikaidoh operation for concordant TGA patients [18–21]. Figure 26.2 Blood flow in congenitally corrected transposition of the great arteries (ccTGA). The “correction” is physiologic only, in the sense that in biventricular hearts with ccTGA a normal oxyhemoglobin saturation may be present in cases without intracardiac shunts (ASD or VSD) and LV outflow tract obstruction (i.e., isolated atrioventricular and ventriculo‐arterial discordance). Ao, aorta; ASD, atrial septal defect; LA, left atrium; LV, left ventricle; LVOTO, left ventricular outflow tract obstruction; PA, pulmonary artery; RA, right atrium; RV, right ventricle; VSD, ventricular septal defect. Source: Reproduced by permission from Karl TR, Cochrane AD. In: Pediatric Cardiac Surgery, 3rd ed., St. Louis, MO: Mosby, 2003, pp. 476–495. Figure 26.3 Anomalies associated with congenitally corrected transposition of the great arteries (ccTGA). The majority of cases (99%) of ccTGA have anatomic abnormalities that could complicate the discordant atrioventricular (AV) and ventriculo‐arterial connections. These additional anomalies can have an important influence on the natural history, the timing of surgery, and the choice of surgical options. LV, left ventricle; LVOTO, left ventricular outflow tract obstruction; RV, right ventricle; TV, tricuspid valve; VSD, ventricular septal defect. The diagnosis of ccTGA and most anatomic details can usually be established accurately using clinical assessment and two‐dimensional echocardiography (Figure 26.6). Cardiac catheterization may provide important information about the hemodynamics, and further delineate anatomic relationships and PA morphology. Angiographic views should define the site and number of VSDs, the morphology of the ventricular chambers, the nature of the LV outflow tract obstruction, and all associated anomalies. The role of more contemporary imaging strategies such as computed tomography angiography (CTA), cardiac magnetic resonance imaging (cMRI), and three‐dimensional modeling in the diagnosis of ccTGA are extremely valuable for preoperative planning and preferences vary from center to center. Historical precedent suggests that unoperated cases of ccTGA have a 32% prevalence of heart failure and a 25% mortality by the fourth decade of life (Figure 26.7). However, in practice the natural history of ccTGA is extremely variable. Infants with severe pulmonary stenosis or pulmonary atresia and those with severe left AV valve insufficiency may present early in life. At the other extreme, some individuals (usually the 1–2% with no associated defects) are asymptomatic for many years and are diagnosed only late in life or at postmortem examination. Reports of untreated individuals with ccTGA surviving into the ninth and tenth decades prove this concept [22, 23]. Likewise, successful pregnancies (without long‐term sequelae) have been reported in women with ccTGA [24]. Signs and symptoms in ccTGA (when present) may be related to abnormal pulmonary blood flow, systemic AV valve insufficiency, bradycardia from heart block, or systemic (right) ventricular dysfunction. The RV function can begin to deteriorate early after certain surgical repairs, or spontaneously (most often during the second decade of life). In general, for both definitively operated and palliated patients, survival is significantly poorer than that of the normal population from early infancy onward. Figure 26.4 Anatomy of the atrioventricular (AV) conduction axis in congenitally corrected transposition of the great arteries (ccTGA), showing morphologic detail (A) and surgical view (B). The anterior and superior location is vulnerable to spontaneous or surgically induced heart block, especially during trans‐mitral valve (MV) ventricular septal defect (VSD) closure. The incidence of spontaneous heart block is 38% by the age of 40 years. ant., anterior; LV, left ventricle; morph., morphologic; MV, mitral valve; RA, right atrium; V, ventricular; VSD, ventricular septal defect. Source: (B) Reproduced by permission from Karl TR, Cochrane AD. In: Pediatric Cardiac Surgery, 3rd ed., St. Louis, MO: Mosby, 2003, pp. 476–495. Figure 26.5 Coronary anomaly in congenitally corrected transposition of the great arteries (ccTGA). This example shows a single coronary artery arising from a nonfacing sinus, an extremely rare variant in both concordant and discordant transposition of the great arteries. Ao, aorta; LA, left atrium; RA, right atrium. LV outflow tract obstruction in ccTGA can cause cyanosis in the newborn period when an intracardiac shunt is present. Some infants may require prostaglandin infusion and a systemic–pulmonary arterial shunt. Children with severe tricuspid insufficiency and/or a large VSD with minimal or no LV outflow tract obstruction may present in the first few weeks of life or any time thereafter with congestive heart failure. The most common presentations during childhood or adolescence are exercise intolerance or poor growth due to a left‐to‐right shunt, or increasing cyanosis and exercise intolerance due to pulmonary stenosis. Progressive systemic AV valve insufficiency may complicate these presentations, or occur as an isolated finding. Some patients present with spontaneous heart block and bradycardia. The proportion with block increases by 2% per year to reach 30% in adult life [25]. Tricuspid insufficiency is a central problem in ccTGA, in both operated and nonoperated patients. The mechanism is probably multifactorial, and not completely understood. Suggested mechanisms include the following: Figure 26.6 Two‐dimensional echocardiography imaging in congenitally corrected transposition of the great arteries (ccTGA). Four‐chamber (left) and subcostal images (right) from the same child are shown. The discordant atrioventricular (AV) connection is well seen. There is reverse offset of the AV valves (tricuspid valve more apically displaced than mitral valve). The great vessels have a parallel (rather than spiral) proximal course. A perimembranous ventricular septal defect (VSD) is also present. Ao, aorta; LA, left atrium; LPV, left pulmonary valve; LV, left ventricle; PA, pulmonary artery; RA, right atrium; RV, right ventricle. Courtesy of Dr. Thieu Nguyen. Figure 26.7 Natural history of congenitally corrected transposition of the great arteries (ccTGA). The course is highly variable and depends to a great extent on the lesions associated with atrioventricular (AV) to ventriculo‐arterial (VA) discordance in individual cases. All anatomy considered, unoperated patients have a 32% prevalence of congestive heart failure (CHF) and a 25% mortality by the fourth decade. Exceptional individuals have survived to an advanced age without treatment. The features of Ebstein anomaly as seen in concordant hearts (such as thinning of the atrialized portion of the RV and severe tethering of leaflets) are not generally present in ccTGA. Furthermore, the typical surgical treatments of Ebstein anomaly in AV concordant hearts (e.g., cone repair) may not be applicable in ccTGA. Hence, the term “Ebsteinoid” is most often applied to the TV in ccTGA, acknowledging that the morphology is significantly different. In some cases of ccTGA, RV hypertrophy eventually gives way to dilation, so the TV papillary muscles are distracted. The short tethered TV chords and displaced leaflets fail to coapt, and tricuspid insufficiency becomes progressive and self‐perpetuating. Tricuspid insufficiency can also be exacerbated by surgical relief of LV outflow tract obstruction, due to unfavorable septal shift that occurs when the LV pressure drops. PA band placement may have the opposite effect and move the septum to a more favorable midline location, improving TV function. Repair of ccTGA was first attempted during the late 1950s [13]. A summary of past and currently used surgical strategies is presented in Figure 26.8. Palliative or interim procedures may be useful and advisable in selected circumstances. Both physiologic and anatomic repairs have been employed for definitive treatment (Figure 26.9). Because the potentially unfavorable natural history of children with ccTGA and a systemic RV has been well documented, many surgical teams have moved toward “anatomic” repair (i.e., placing the RV in the pulmonary circuit and the LV in the systemic circuit), instead of the “physiologic” approach (leaving the RV in the systemic circuit and the LV in the pulmonary circuit). The latter strategy fails to address AV discordance and its eventual detrimental consequences, despite the correction of associated anatomic aberrations. This of course may have an important influence on the midterm and late outcome despite a good immediate result. Univentricular and 1.5‐ventricular repairs can also be employed selectively [26]. Figure 26.8 Taxonomy of some operative strategies used for congenitally corrected transposition of the great arteries (ccTGA). Bold type implies systemic left ventricle (LV), italic type implies systemic right ventricle (RV) and LV. ASO, atrial septal occluder; BCPS, bidirectional cavopulmonary shunt; CHF, congestive heart failure; LVOTO, left ventricular outflow tract obstruction; PA, pulmonary artery; TV, tricuspid valve; VSD, ventricular septal defect. Figure 26.9 Comparison of physiologic and anatomic definitive repair strategies for congenitally corrected transposition of the great arteries (ccTGA) with ventricular septal defect (VSD). (A) The VSD has been closed, so although septated, the discordant connections remain. (B) A VSD closure and double switch (Senning plus arterial switch operation) have been performed, correcting the atrioventricular to ventriculo‐arterial discordance as well. The latter procedure thus has theoretical advantages, although the operation tends to be more complex. Ao, aorta; LV, left ventricle; PA, pulmonary artery; RA, right atrium; RV, right ventricle. The principal palliative operations used in ccTGA are systemic‐to‐pulmonary artery modified Blalock–Taussig–Thomas (BTT) shunts and PA banding. Isolated repair or replacement of the TV is also appropriate in select circumstances. Pacemaker implantation and cardiac resynchronization may be of benefit in patients with complete heart block and RV dysfunction [25]. The modified BTT shunt may be a useful strategy for ccTGA patients in the following circumstances: We prefer a transsternal approach for all modified BTT shunts, usually employing a 4 mm polytetrafluoroethylene (PTFE) tube graft interposition between the brachiocephalic artery and right PA. With this approach, subsequent takedown is facilitated, and PA distortion can be minimized. The majority of children with ccTGA (especially those with apicocaval ipsilaterality, i.e., cardiac apex and inferior caval vein [IVC] on the same side) do have some natural limitation of pulmonary blood flow, so PA band placement specifically for control of pulmonary arterial blood flow is not frequently required [28]. However, other considerations may apply. A PA band may be indicated under the following circumstances: The best surgical strategy for the various forms of ccTGA is debatable, and surgeon‐ and team‐dependent factors are important. Problems in decision‐making are magnified by the rarity of the lesion. All operations are theoretically possible from the neonatal period onward, but not necessarily advisable. Repair has been performed as late as the fifth decade of life [41, 42]. Features favoring the physiologic approach include competence of both AV valves, balanced ventricular size, and excellent RV function. Features favoring the anatomic approach include disparity in ventricular size, poor RV function, tricuspid insufficiency, and a nearly systemic LV pressure (LV outflow tract obstruction, secondary pulmonary hypertension, or following PA banding). In general, anatomic (as opposed to physiologic) correction requires a more complex surgical approach, but may have a better long‐term outcome. Complexity scores and anticipated mortality scores have been determined for various congenital heart operations, including the Aristotle Complexity Score and the Society of Thoracic Surgeons–European Association for Cardio‐Thoracic Surgery Congenital Heart Surgery Mortality Score (STAT) [27, 43, 44]. The double‐switch operation, for example, has a STAT mortality category of 5 (highest expected level of mortality) and an Aristotle Complexity Score of 13.8, rising to 18.8 in a patient with prior transsternal PA banding for LV conditioning. In comparison, STAT mortality categories for a physiologic correction involving isolated VSD closure in ccTGA or VSD closure with LV–PA conduit placement are 3 and 4, respectively, suggesting a significant difference in early mortality risk [42–44]. Although the late results of physiologic repair are well documented, the late results of anatomic repair are still under assessment, and it is fair to say that the long‐term relative benefit for patients who are suitable for either type of repair remains theoretical. Current thought suggests that an anatomic approach is a better prospect for patients who already have problems with the RV and/or TV, and who are therefore poor candidates for physiologic repair. For example, relief of LV outflow tract obstruction in the context of a physiologic repair has the theoretical disadvantage of increasing the tricuspid insufficiency over time by allowing a ventricular septal shift in an unfavorable direction, with the expected compromise in outcome [4, 43, 45]. The cavopulmonary connection (Fontan) strategy is useful for many complex anatomic situations in which the risk of septation outweighs the benefits. BCPS may be selectively applied for anatomic, physiologic, and univentricular approaches (see below). Children with ccTGA who are not candidates for biventricular repair (i.e., those with important straddling of an AV valve, unbalanced ventricular size, and multiple unfavorable VSDs) can be palliated with a BCPS followed by Fontan. We have also employed an interventional strategy for the Fontan operation after appropriate preparation at the BCPS stage [46]. For an extracardiac Fontan operation (all pathologies considered) the STAT score is 2, and biventricular Fontan candidates may be superior candidates (as compared to those with complex univentricular anatomy) [4]. The Fontan operation may, in fact, impart an event‐free survival advantage over physiologic and anatomic biventricular repairs, with similar or better exercise capacity and quality of life, even for patients who, theoretically, could undergo biventricular repair [37, 47, 48
CHAPTER 26
Congenitally Corrected Transposition of the Great Arteries
Important Historical Aspects
Diagnosis
Natural History and Clinical Presentation
Surgical Indications and Options
Interim (Palliative or Nondefinitive) Operations for ccTGA
Definitive Operations for ccTGA
Stay updated, free articles. Join our Telegram channel

Full access? Get Clinical Tree
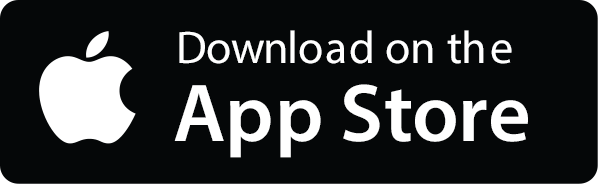
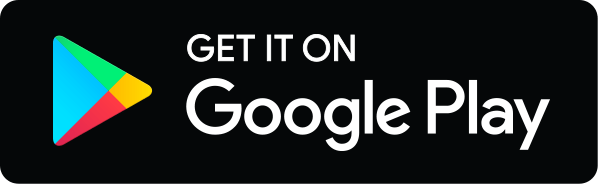