Tissue Regeneration of the Cardiovascular System and Stem Cells: Introduction
Despite great advances in the therapy of cardiovascular diseases within the past several decades, ischemic heart disease and congestive heart failure (CHF) continue to be leading causes of mortality and morbidity in the Western hemisphere.1 Myocyte loss as well as structural and functional abnormalities of the myocyte are observed in the syndrome of CHF, regardless of its cause.2 Although much of molecular cardiology over the past 2 decades has been devoted to the understanding of myocyte abnormalities in heart failure, only recently has regenerative therapy of the heart emerged as an exciting therapeutic modality.3 Unlike hearts of lower animals such as newts and zebrafish,4 which regenerate completely after injury, the human heart has been traditionally thought of as a terminally differentiated organ incapable of regeneration.5 This traditional line of thinking has been challenged recently by observations in animals and humans of the existence of cardiac6,7 and extracardiac progenitor cells (CPCs) capable of forming cardiomyocytes, endothelial as well as smooth muscle cells.8-14 These observations in turn have engendered clinical trials of stem cell therapy aimed at regenerating the heart after acute myocardial infarction (MI) and in chronic left ventricular failure.15
This chapter provides a brief overview of the emerging field of cardiac regenerative therapy. The chapter discusses the scientific basis for cardiac regenerative therapy, examines the types of stem cells used and mechanisms of action and mode of delivery, addresses safety concerns, reviews clinical trials of cardiac cell therapy, and finally summarizes the questions that confront the future of this rapidly developing field.
Scientific Basis of Cardiac Regenerative Therapy
The concept of the heart as a terminally differentiated organ incapable of replacing myocytes has been an accepted paradigm over the past 50 years.3 However, several lines of evidence from animal and human studies have emerged over the past few years to challenge this existing paradigm. A number of experimental studies in animals supported the possibility of stem cell–based cardiac regeneration. Orlic et al8 demonstrated that bone marrow cells injected into the infarcted mouse heart regenerated infarcted myocardium and led to improvement in cardiac function. More recently, Beltrami and colleagues6 identified a resident CPC capable of differentiating into cardiomyocyte, endothelial, and smooth muscle lineages. Injection of these cells into the injured heart led to regeneration of functioning myocardium and improvement in cardiac function. Studies in human subjects supported the above-mentioned experimental observations. The presence of Y chromosome–positive cardiomyocytes in female hearts transplanted into male recipients directly pointed to the presence of putative progenitors capable of de novo cardiomyocyte formation (Fig.11–1A,B).11,14 Furthermore, the presence of chimeric cardiomyocytes, albeit at low numbers, in the hearts of patients who had undergone gender mismatched bone marrow transplantation strongly suggested the existence of bone marrow progenitors capable of contributing to cardiomyocyte formation in the adult human heart (Fig. 11–1C).10 Taken together, a large body of experimental evidence thus suggests the existence of progenitors capable of cardiomyocyte formation in postnatal life. Although these observations challenge the concept of the heart as a postmitotic organ, cardiac regeneration with complete restoration of cardiac function after injury does not occur in higher mammals, including humans. Several strategies have thus evolved to assist the postnatal heart in regenerating its damaged cells after injury.2 These have included strategies to (1) force existing cardiomyocytes to override cell cycle check points and reenter cell cycle16 (2) stimulate angiogenesis by delivery of angiogenic cytokines or cells capable of forming new blood vessels,17,18 and (3)deliver exogenous progenitor cells that help in cardiac repair19 or are capable of forming cardiac muscle itself.6 Regenerative therapy of the heart has so far primarily involved the latter two strategies, namely the use cytokines or progenitor cells for cardiac repair. (Table 11–1 and Fig. 11–2)
FIGURE 11–1
Cardiomyocyte chimerism in humans. Y chromosome–positive cardiomyocyte (arrowhead, A) (red dot, B) in a male patient who underwent gender-mismatched cardiac transplantation. Y chromosome–positive cardiomyocyte (arrow, C) present in a female patient who underwent gender-mismatched bone marrow transplantation. With permission from Quaini et al14; Laflamme et al11; Deb et al10.
Type | Type of Trial |
---|---|
Embryonic stem cell | Animal study only |
Skeletal myoblasts | Clinical study |
Whole bone marrow cell | Clinical study |
Bone marrow mononuclear cell | Clinical study |
Mesenchymal stem cell | Clinical study |
CD34+ stem cells | Clinical study |
CD133+ stem cell | Clinical study |
Peripheral blood-derived progenitor cell | Clinical study |
Endothelial progenitor cell | Clinical study |
Resident cardiac progenitor cell | Animal study only |
Bone marrow–derived multipotent adult progenitor cell | Animal study only |
Induced pluripotent stem cell | Animal study only |
FIGURE 11–2
Sources of cells for cardiac stem cell therapy. MSC, mesenchymal stem cell; SP, side population. Adapted with permission from Dimmeler et al.2
Types of Cells Used for Cardiac Regeneration and Repair
Because of their inherent myogenic differentiation potential, autologous skeletal myoblasts were among the first cells to be considered for cardiac stem cell therapy and repair.20,21 Initial studies have shown that myoblasts can expand easily in vitro, show enhanced resistance in ischemic conditions, and hold regenerative capacity.15,22 Moreover injection of these cells into rodent hearts resulted in improved ventricular function and reduced remodeling.21,23 Still, limitations in the therapeutic potential of skeletal myoblasts for stem cell therapy do exist. First, skeletal myoblasts do not differentiate into cardiac myocytes in vivo but rather develop into myotubes.22 Additional limitations include the inability of those cells to synchronize with the surviving myocardium,2 therefore posing the risk of arrhythmia and the risk of inducing embolic damage because of obstruction to the microvasculature after their administration.2 Initial clinical trials have shown that improvements in ejection fraction persist 10 months after injection.5 However, the MAGIC trial,24 which randomized patients to receive either stem cell injection or placebo control, failed to improve echocardiographic heart function. Further preclinical and clinical studies are ongoing and may reveal differing results. Experiments in animals for example have shown that overexpression of connexin 43 gap junction protein on skeletal myoblasts can enhance their electrophysiologic coupling with cardiac myocytes suggesting that the risk of inducing arrhythmias can be circumvented.25
Ferrari26 first reported almost a decade ago the generation of skeletal muscle from bone marrow cells in mice. Since this initial observation, several groups of investigators have studied the ability of bone marrow cells to regenerate cardiomyocytes. It is important to appreciate that the bone marrow contains a varied assortment of progenitor cells with putative cardiac potential such as hematopoietic stem cells (HSCs), mesenchymal stem cells (MSCs), very small embryonic-like stem cells, multipotent adult progenitor cells, and so on.5 Here we focus on progenitors that have drawn the most attention and thus are the best studied thus far.
A number of reports have shown that bone marrow progenitor cells have the capacity to differentiate into cardiac myocytes in vitro, opening the potential for the use of those cells in stem cell therapy27 for the heart. Numerous follow-up in vivo studies indicated that administration of bone marrow hematopoietic progenitors into the diseased myocardium or mobilization of the endogenous population into the area of cardiac injury consistently led to increased cardiac function.28-30 Initial data suggested this improvement was mainly attributable to cardiac myocyte regeneration. However, these results could not be consistently reproduced.31,32 by follow-up studies, and the question of whether bone marrow cells can regenerate cardiomyocytes in the infarcted heart remains unsettled. Despite the controversy, it is widely accepted today that bone marrow hematopoietic progenitors can integrate into the myocardium, albeit at low numbers, and that they can improve cardiac function, regardless of direct regeneration, via indirect proangiogenic or other paracrine effects. These promising experiments in improvement of ventricular function have led to the design and executing of a number of clinical trials in patients with MI. The majority of them have used bone marrow mononuclear cells and have shown, at best, modest improvements in cardiac function and in some cases improved microvascular function.33
MSCs are multipotent cells that reside in the stromal component of the bone marrow and are also known as stromal cells. They lack typical hematopoietic cell surface markers such as CD34 and CD45, and they can differentiate into a number of lineages such bone, cartilage, adipose, and skeletal muscle34 under special culture conditions. Several reports suggest that these cells could also be induced to differentiate into beating cardiomyocytes in vitro, igniting a lot of interest for their use for cardiac regenerative therapy.5 Several additional unique properties of MSCs contribute to enhancing their advantages as cell therapy agents. First, they do not elicit a robust host immune response, a property that might allow them to survive in an allogeneic transplantation setting.35 Moreover, systemically injected MSCs possess an inherent ability to home to the injured heart, but in the absence of injury, they home to the bone marrow.36
A number of preclinical studies have confirmed the salutary effects of injecting MSCs into the injured heart, showing that direct injection of MSCs into infarcted rat, mouse, and pig hearts improved ventricular function.37 Injection of genetically labeled MSCs into infarcted mouse hearts demonstrated a small percentage of MSC-derived cardiomyocytes but improved cardiac performance.38 Moreover, a clinical study of MSCs in 69 postinfarct patients also demonstrated improved left ventricular function.39
MSCs do not appear to integrate electromechanically with the recipient myocardium but do exert beneficial effects on postinfarct ventricular remodeling. Mangi et al19 also demonstrated dramatic reduction of infarct size when MSCs overexpressing the pro-survival gene Akt were injected into rodent hearts at the time of MI. Interestingly, MSCs appeared to be exerting their cytoprotective effects through paracrine mechanisms as injection of MSC conditioned medium into infarcted rodent hearts yielded similar degrees of myocardial protection.40 It appears currently that MSCs likely exert their beneficial effects through panoply of mechanisms pertaining to myocardial protection, ventricular remodeling, angiogenesis, and possibly myocyte regeneration. Several other groups of investigators have isolated other rare progenitors from the stromal component of the bone marrow that have differentiated both in vivo and in vitro to cardiomyocytes.41 The immunotolerant properties of MSCs, ease of handling, and ability to home to injured myocardium after systemic delivery have made them attractive tools for cardiac regenerative therapy.
Embryonic stem cells are derived from the inner cell mass of the blastocyst, can be propagated indefinitely in an undifferentiated state, and are pluripotent (capable of differentiating into tissues belonging to the three germ layers).42 In culture, they spontaneously form cystic structures known as embryoid bodies that contain foci of beating cardiomyocytes. However, when injected into the heart, owing to their pluripotent nature, embryonic stem cells differentiate into undesirable tissue lineages and give rise to teratomas. Thus, much of the research in the use of embryonic stem cells for cardiac regeneration has focused on strategies to direct differentiation into a particular cell type as well as to enhance the yield of isolation of embryonic stem cell–derived cardiomyocyte. Various chemicals and molecules have been used to enhance cardiomyogenic differentiation of embryonic stem cells, including retinoic acid,43 ascorbic acid,44 transforming growth factor β,45 bone morphogentic proteins46 or their antagonist noggin,47 and members of the Wnt48 family of proteins. Although cardiomyogenic differentiation was substantially increased with the use of such a variety of chemicals and proteins, a universal recipe for efficient and large-scale regeneration of cardiomyocytes from embryonic stem cells is still elusive.5 Klug and coworkers49 were the first to pioneer a genetic selection strategy to enhance isolation of cardiomyocytes from embryonic stem cells. Using a selection marker driven by a cardiac promoter, these authors reported a highly purified population of embryonic stem cell–derived cardiomyocytes. Furthermore, injection of cardiomyocytes derived by the above strategy stably engrafted into hearts of immunocompromised animals. Since this observation, other groups have also reported success in improving cardiac function by stable engraftment of embryonic stem cells that seemed to integrate functionally into the recipient myocardium50-52 and not generate teratomas.53
More recently, human embryonic stem cell–derived cardiomyocytes have been shown to successfully engraft and electromechanically integrate when injected into uninjured hearts of immunosuppressed animals.54-56 In these studies, the injected cells behaved as a biological pacemaker and electrically excited the rest of the ventricle. Although these early studies have generated enthusiasm and provided important proof of concept data for generation of biological pacemakers, the field is still in its infancy and is plagued with problems of large-scale generation of embryonic stem cell–derived cardiomyocytes, immunologic rejection, differentiation into undesirable lineages, and finally ethical concerns. Nevertheless, these pluripotent cells still remain an attractive and powerful tool for cardiac regenerative therapy.
The concept of the heart as a static organ incapable of turnover or regeneration was challenged by several groups of investigators who described a CPC capable of multilineage differentiation. Beltrami and coworkers6 were the first to describe the existence of a resident CPC capable of differentiating into myocytes as well as endothelial and smooth muscle cells. The c-kit + CPC isolated by Beltrami et al6 from the adult rat heart was demonstrated to be self-renewing, clonogenic, and multipotent. In the presence of specialized differentiating medium, c-kit + cells differentiated into cardiomyocyte-like cells bearing myocyte-specific structural proteins. Furthermore, injection of clonogenic c-kit + cells into the infarcted rodent heart resulted in multilineage differentiation of these cells into myocytes, endothelial cells, and smooth muscle cells as well as improved ventricular performance. Intracoronary injection of c-kit + cells after ischemia-reperfusion injury also resulted in improved myocardial performance, diminished scar formation, and new myocyte regeneration independent of cell fusion. C-kit + cells have also been isolated from human myocardial biopsy specimens as well and demonstrated to differentiate into functional cardiomyocytes after injection into immunocompromised mice.57
Since then, other investigators have reported the existence of CPCs identified by other stem cell markers such as Sca-1, Isl-1, and ABC transport protein. Oh et al7 demonstrated that a Sca-1 population of cells isolated from adult mouse hearts when treated with azacytidine expressed cardiac-specific genes and after injection into the infarcted mouse heart improved cardiac function by de novo cardiomyocyte formation as well as fusion with existing cardiomyocytes in roughly equal proportions. Laugwitz et al58 demonstrated that cells possessing the LIM homeo-domain transcription factor Isl-1 isolated from neonatal mouse hearts could rapidly differentiate into beating cardiomyocytes persistently expressing mature cardiac-specific proteins and generating action potentials. However, Isl-1 cells are rare in the adult heart, and the functional significance and the role of these cells in cardiac homeostasis are not entirely clear. Currently, it is not clear whether there are biological differences in CPCs bearing different stem cell markers. Nevertheless, most populations of resident CPCs have improved cardiac function after injection into the postinfarct heart. Importantly, the multilineage differentiation capacity of these cells into endothelial and smooth muscle cells represent an important advantage because regenerating myocytes need blood vessels for survival and effective functioning.
Asahara et al59 first described the existence of circulating endothelial progenitor cells in humans. They isolated CD34+ cells from human peripheral blood and demonstrated that under specific conditions, the proliferating cells displayed endothelial cell–like properties in vitro and incorporated into neovasculature when injected into ischemic hind limbs of mice and rabbits. Since the past decade, the field of endothelial progenitor biology has rapidly expanded. Several groups of investigators have consistently demonstrated that these cells help in formation of de novo blood vessels and lead to improvement in blood flow of ischemic regions. Similar to HSCs, they can be mobilized into the peripheral circulation with the use of cytokines and have been shown to home to ischemic regions party mediated by SDF and CXCR4 signaling.60 The number as well as function of endothelial progenitors is impaired in conditions such as diabetes and coronary artery disease.61 Endothelial progenitors have now been used in several clinical trials of MI and hind limb ischemia. Although very few trials so far have been randomized and placebo controlled, the cells appear to be safe, and therapeutic outcomes are promising. Myocardial blood flow, wall motion abnormalities, ejection fraction, and even chamber dimensions seemed to improve after administration of endothelial progenitors derived from autologous bone marrow or peripheral blood.62 In some reports, an increase in exercise time and decreased number of anginal episodes was noted after cell therapy. Interestingly, these cells also appear to secrete angiogenic cytokines such as vascular endothelial growth factor, hepatocyte growth factor (HGF), and granulocyte colony-stimulating factor (G-CSF).63 Whether endothelial progenitors exert their effects by direct incorporation into new vessels or through paracrine signaling is currently unclear. It is likely that the pleiotropic salutary effects of endothelial progenitors are secondary to a combination of paracrine signaling and direct incorporation into de novo blood vessels.
In a pioneering paper, Yamanaka and colleagues recently demonstrated that human skin fibroblasts can be converted into embryonic stem cells with overexpression of four transcription factors, Oct4, Sox2, Klf4, and C-myc.64 Embryonic stem cells derived from reprogramming fibroblasts are known as iPS (induced pluripotent stem cells).65 This discovery promises to revolutionize the field of cell therapy because it opens up the possibility of deriving patient-specific cell lines for cardiac cell therapy. For instance, a patient’s skin cells can be reprogrammed to generate patient specific embryonic stem cells that could be used for cell therapy purposes. iPS cells can form beating cardiomyocytes in vitro and contribute to de novo cardiomyocyte formation when incorporated into the developing blastocyst.66 Studies determining the efficacy of iPS cells for cardiac diseases are still in their infancy and are technologically limited for human use secondary to the need for viruses for generating iPS cells. However, virus-free generation of human iPS cells has been successfully carried out and appears promising for future iPS cell therapy.67
Mechanisms of Action of Stem Cells in Cardiac Regeneration and Repair
Although multiple experimental animal models and several clinical trials of cell-based cardiac therapy have shown promising results, the mechanisms of such cell-mediated benefits are rather unclear. As illustrated in Fig. 11–3, stem cells could act via several potential mechanisms, which could then dictate specific functional benefits. It is also likely that a type of stem cell could exert more than one mechanism of action. Undoubtedly, interplay between the disease condition, microenvironment, and type of stem cell will ultimately determine the functional benefits. The following section discusses some of the potential mechanisms mediating beneficial effects of stem cell therapy on the heart.
FIGURE 11–3
Mechanisms of action. Progenitor cells may improve functional recovery of infarcted or failing myocardium by various potential mechanisms, including direct or indirect improvement of neovascularization. Paracrine factors released by progenitor cells may inhibit cardiac apoptosis, affect remodeling, or enhance endogenous repair (eg, by tissue-resident progenitor cells). Differentiation into cardiomyocytes may contribute to cardiac regeneration. The extent to which these different mechanisms are active may critically depend on the cell type and setting, such as acute or chronic injury. Reproduced with permission from Dimmeler et al.2
Stem cells depending on their lineage commitment possess the ability to differentiate into cells of various tissues. Embryonic cells are pluripotent, can generate tissues belonging to all three germ layers, and can differentiate and electromechanically integrate with existing cardiomyocytes in animals. Adult stem cells are thought to be more committed and possess a limited ability to differentiate along a specific lineage. In contrast to embryonic stem cells, differentiation of adult bone marrow stem cells into functional cardiomyocytes has been more difficult to demonstrate and fraught with controversy.68 However, several groups of investigators have demonstrated that resident cardiac progenitors are capable of adopting a cardiomyogenic fate both in vitro and in vivo.57
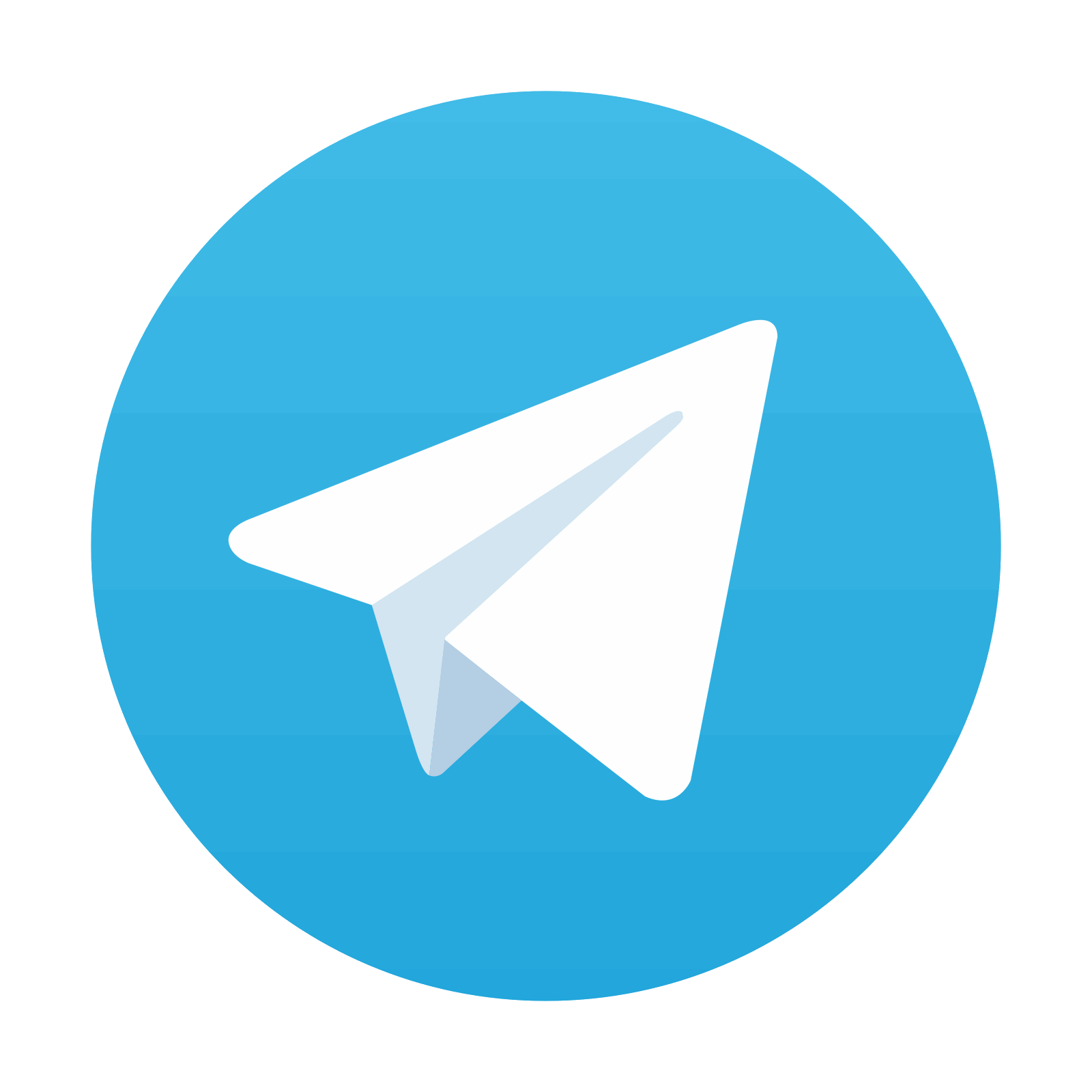
Stay updated, free articles. Join our Telegram channel

Full access? Get Clinical Tree
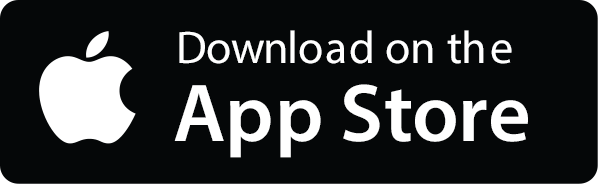
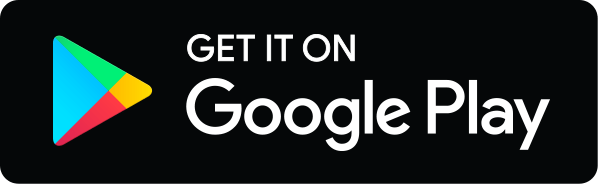
