Thoracic Ultrasonography
INTRODUCTION
Thoracic ultrasonography is a readily available, noninvasive imaging method that has proved particularly useful for pulmonary and critical care specialists. The technique is easy to learn and engenders multiple applications related to lung and pleural disease. The ease of use and portability of ultrasonography allows physicians to perform point-of-care imaging and reduce dependence on traditional thoracic imaging techniques, such as chest computerized tomography (CT) and standard radiography (CXR).
This chapter focuses on use of ultrasonography for diagnosis and management of pleural and lung diseases and emphasizes those areas that are of particular interest to the pulmonary specialist. Many elements of thoracic ultrasonography that have utility for the pulmonary consultant are also germane to critical care medicine. However, this chapter focuses on the broad context of pulmonary medicine, rather than the elements of thoracic ultrasonography that are specific to critical care. Furthermore, the chapter is written with the assumption that the pulmonary consultant will personally perform image acquisition, interpret the image, and apply results at the bedside—a paradigm that is different from the standard one of thoracic imaging requiring reliance on a radiologist’s acquisition and interpretation of the image. While this approach is advantageous with regard to cost and clinical efficiency, it requires technical and cognitive skills. An additional advantage of clinician-performed, point-of-care thoracic ultrasonography is that it couples imaging results with the clinician’s overall knowledge of the case. Indeed, thoracic ultrasonography is not performed in vacuo, rather, it is always combined with other key elements of clinical evaluation, such as the history, physical examination, and laboratory analysis. Ultrasonography is an additional tool in the diagnosis and management of pleuropulmonary disease.
TRAINING IN THORACIC ULTRASONOGRAPHY
The goal of training in thoracic ultrasonography is, of course, to enable clinical competence in performing the procedure. “Competence” must be defined to facilitate development of specific learning objectives as the goals of training. One pragmatic approach is to establish a reasonable minimum standard for competence. The Statement of Critical Care Ultrasonography provides a well-defined description of training goals in pleural and lung ultrasonography.1 The statement is a good starting point for pulmonary specialists who seek training in the field, and the competencies outlined should constitute the initial learning objectives.
No definitive literature is available to guide the optimal duration of training or the minimal number of studies to be performed and interpreted. No widely accepted course of study for the cognitive elements of the field exists, and, at present there is no formal certification process.
Despite these limitations, many clinicians are competent in thoracic ultrasonography. Compared with the training required in the complex fields of cardiac and abdominal ultrasonography, training in thoracic ultrasonography is straightforward. The authors have considerable experience in training many fellows and attending physicians in thoracic ultrasonography and have found that a motivated learner will achieve skill in the basic elements of pleural and lung ultrasonography within several hours of formal teaching. Training should include practicing image acquisition, initially on normal human models and then on patients at the bedside. In addition, training should include a review of a comprehensive image set of normal and abnormal findings, enabling the learner to recognize a wide range of findings during their initial encounter at the bedside. The cognitive elements of the field may be learned through reading chapters or review articles.2–4 For more advanced training, comprehensive textbooks are available.5,6 Formal courses on thoracic ultrasonography are offered. In addition, the learner may choose to develop a local training resource, which requires identification of a local expert who is able to help with bedside training.
EQUIPMENT NEEDS
Thoracic ultrasonography may be performed using a wide variety of ultrasound machines. Virtually any machine that is used for or cardiac or abdominal ultrasonography will generally yield adequate image quality. A probe designed for cardiac ultrasonography, employing a frequency range of 3.5 to 5.0 MHz works well, as the small footprint of the probe allows for easy placement between rib interspaces; frequency selection allows for adequate penetration to enable imaging the deeper structures of the thorax. A larger, curvilinear abdominal probe gives serviceable images as well. A linear vascular probe using a frequency range of 7.5 to 10.0 MHz is required for detailed imaging of the pleural surface; the higher frequency improves the resolution, but at the sacrifice of signal penetration.
Every machine has its own design characteristics, so that the operator must adapt machine setup to optimize image quality. What works well using one machine may not translate into the same effectiveness when using another. Some machines have presets for thoracic ultrasonography, but they may not necessarily be the optimal setup for thoracic imaging. Consequently, the operator must be prepared to alter machine settings from standard cardiac, abdominal, or thoracic presets. Machines from the 1990s that lack extensive postimage processing often give excellent results. Modern, high-end cardiac echocardiography machines give excellent cardiac image quality, but they often yield poor near-field images. Many modern portable machines have acceptable thoracic imaging capability and provide the additional advantage that they are designed specifically for point-of-care scanning.
SCANNING TECHNIQUE
For the pulmonary specialist, thoracic ultrasonography may be performed with the patient in a seated position. The supine scanning position is more typical for the critically ill patient, but it has the disadvantage that it is difficult to scan the posterior chest. The standard transducer orientation is the transducer indicator oriented in a cephalad position, defining the primary scanning plane in a longitudinal axis. With the screen indicator placed to the left of the screen, images on the left side of the screen represent cephalad structures.
Machine control is integral to good quality image acquisition; hence, total, near, and far field gains must be adjusted for optimization. Depth should be set to place the target structure in the middle of the screen, and the focal point should be adjusted for optimal image quality of the target structure. Using adequate ultrasound coupling gel and application of firm pressure, the transducer is applied perpendicular to the chest wall and adjusted to examine the rib interspace. Adjacent interspaces are examined by sliding the transducer to the next interspace. In this way, the examiner performs a scan line while the transducer is moved over the chest wall. In an orderly fashion, multiple adjacent scan lines are performed so that the entire chest wall can be imaged. Using this approach, the examiner obtains multiple, two-dimensional, tomographic ultrasound planes of the thorax, in effect, developing a three-dimensional model. If a focal abnormality is identified, the examiner may then perform a more detailed ultrasonographic examination of the area.
Aerated lung blocks ultrasound, whereas the liver and spleen do not. Consequently, to visualize the peridiaphragmatic area, the transducer may be angled such that the scanning plane passes through the liver or spleen to obtain an adequate image window. In addition, bone also blocks ultrasound. So, when an abnormality lies deep to a rib, the transducer may need to be angled to look over or under the rib. Conversely, pleural effusion and consolidated lung each transmit ultrasound. Their presence may yield a window for examination of the heart, mediastinum, or, in the case of pleural effusion, the lung. While the standard scanning plane is longitudinal, a transverse plane may be utilized on occasion. This may be required when examining for a “lung point” or to establish an optimal angle for needle insertion for access to a lung mass. Most thoracic ultrasonography is performed using a cardiac or abdominal transducer. A vascular transducer is preferred if detailed examination of pleural anatomy is required.
PLEURAL ULTRASONOGRAPHY
Ultrasonography is particularly effective for identifying pleural fluid, since fluid is either anechoic or hypoechoic relative to adjacent soft tissue. While physical examination is neither specific nor sensitive for detection of pleural effusion,7 a pleural effusion as small as 5 cc may be identified with ultrasonography.8 Pleural ultrasonography is superior to standard chest radiography in identifying pleural effusions and in differentiating a pleural effusion from pleural thickening or atelectasis.7,9 Compared with chest CT, pleural ultrasound demonstrates 93% sensitivity and specificity for identification of pleural effusion.10 When a patient has complete hemithoracic opacification on CXR, ultrasound has 95% sensitivity for identification of pleural effusion.9
IDENTIFICATION OF PLEURAL FLUID
The pulmonary specialist will generally examine the patient in the seated position. Pleural fluid, unless loculated, distributes by gravitational effect to the most dependent part of the thorax. Therefore, the ultrasonography examination focuses on the lower, dependent area of the posterior thorax.
The examiner seeks to identify three characteristic findings indicating the presence of a pleural effusion: (1) An anechoic or hypoechoic space that is surrounded by typical anatomic boundaries. This space represents the pleural effusion. Diagnosis requires definitive identification of a relatively echo-free space surrounded by the typical anatomic boundaries (Fig. 31-1; Video 31-1). (2) Typical anatomic boundaries. This element requires definitive identification of the chest wall, lung surface, and diaphragm. The heart may form an anatomic boundary on the left side. Recognition of the diaphragm requires definitive identification of the subdiaphragmatic organs (including liver, spleen, and kidneys) (Fig. 31-2; Video 31-2). (3) Dynamic changes typical of a pleural effusion, as described later) (Video 31-3).
Figure 31-1 Typical anatomic boundaries that surround a hypoechoic pleural effusion. The image was obtained using a 3.5-MHz transducer. The transducer was in longitudinal orientation and placed perpendicular to the chest wall to scan through the seventh intercostal space in the right midaxillary line.
Figure 31-2 Pleural effusion above the diaphragm, liver, hepatorenal space, and kidney. These structures must be positively identified before thoracentesis to avoid inadvertent subdiaphragmatic device insertion. The image was obtained using a 3.5-MHz transducer. The transducer was in longitudinal orientation and placed perpendicular to the chest wall to scan through the eighth intercostal space in the right midaxillary line.
Video 31-1 Typical anatomic boundaries that surround a hypoechoic pleural effusion: chest wall, surface of lung, and diaphragm. The image was obtained using a 3.5-MHz transducer. The transducer was in longitudinal orientation and placed perpendicular to the chest wall to scan through the seventh intercostal space in the right midaxillary line. Access at www.fishmansonline.com
Video 31-2 Pleural effusion above the diaphragm, liver, hepatorenal space, and kidney. These structures must be positively identified before thoracentesis to avoid inadvertent subdiaphragmatic device insertion. The image was obtained using a 3.5-MHz transducer. The transducer was in longitudinal orientation and placed perpendicular to the chest wall to scan through the eighth intercostal space in the right midaxillary line. Access at www.fishmansonline.com
Video 31-3 Dynamic findings typical of a pleural effusion, including movement of atelectatic lung, movement of the diaphragm, and movement of echogenic elements within the effusion (plankton sign). The image was obtained using a 3.5-MHz transducer. The transducer was in longitudinal orientation and placed perpendicular to the chest wall to scan through the sixth intercostal space in the right midaxillary line. Access at www.fishmansonline.com
Usually, pleural fluid is hypoechoic relative to the liver or spleen. However, complex pleural effusions may demonstrate echogenicity similar to these organs. The chest wall, as a “stationary” structure, does not show dynamic changes with respiration. Lung that is adjacent to, or surrounded by, pleural fluid is compressed by the pleural effusion and, consequently, is airless. The atelectatic lung, which is of tissue density and presents a pattern of alveolar consolidation on ultrasonography examination, is visualized as “floating” in the pleural fluid. It moves in both cardiac- and respiratory-phasic fashion—one of the typical dynamic changes required for identification of pleural effusion. Other typical dynamic changes include those of swirling debris or fibrin strands agitated by respiratory or cardiac motion. On occasion, a highly cellular pleural effusion forms a layer because of gravitational effects if the patient is immobile for a period of time; patient movement disrupts the fluid-to-cell interface.
Identification of the diaphragm is imperative for localizing a pleural effusion and enabling safe performance of thoracentesis. Subdiaphragmatic device insertion is a serious complication of thoracentesis.
The diaphragm is a curvilinear structure lying above the spleen and liver, which demonstrates respirophasic movement. The inexperienced operator may mistake the hepatorenal or splenorenal space for the diaphragm, as they appear as curvilinear structures that resemble the diaphragm. The overlying spleen or liver may then be mistaken for an echo-dense pleural effusion. This may result in inadvertent subdiaphragmatic device insertion while attempting a thoracentesis with potentially catastrophic consequence to the patient (Fig. 31-2; Video 31-2). It is helpful to identify the kidney when scanning below the diaphragm to avoid this dangerous pitfall. Identification of the chest wall allows measurement of the depth required for needle penetration when planning thoracentesis. Identification of the underlying lung allows the operator to limit the depth of needle insertion to avoid visceral pleural laceration.
CHARACTERIZATION OF THE PLEURAL EFFUSION
Ultrasound examination can be helpful in distinguishing transudative from exudative effusions. Transudates lack constituents that are ultrasound reflectors and are, therefore, echo-free (anechoic) (Fig. 31-3; Video 31-4). An anechoic pleural effusion is very likely to be transudative, although some may have a complex, nonseptated pattern.11 Slow flowing movement of ill-defined heterogeneous elements within a hypoechoic effusion, referred to as swirling, may be observed with a transudate, but its presence suggests an exudative cellular effusion, such as that associated with malignancy.12
Figure 31-3 Anechoic pleural effusion, likely a transudate. The image was obtained using a 3.5-MHz transducer. The transducer was in longitudinal orientation and placed perpendicular to the chest wall to scan through the sixth intercostal space in the right midaxillary line.
Video 31-4 Anechoic pleural effusion, likely a transudate. The image was obtained using a 3.5-MHz transducer. The transducer was in longitudinal orientation and placed perpendicular to the chest wall to scan through the sixth intercostal space in the right midaxillary line. Access at www.fishmansonline.com
A heterogeneously echogenic pattern, with swirling echoes, septations, fronds, or strands is typically observed with exudates. However, very cellular exudates, such as empyema or hemothorax, may demonstrate homogeneous echogenicity (Fig. 31-4; Video 31-5).13 Exudates are usually echogenic, but are occasionally anechoic. The presence of strands, debris, or septations is characteristic of a parapneumonic effusion or empyema.14
Figure 31-4 Pleural effusion with a homogeneously echogenic pattern and mobile strands suggestive of an exudate. Thoracentesis showed a hemothorax. The image was obtained using a 3.5-MHz transducer. The transducer was in longitudinal orientation and placed perpendicular to the chest wall to scan through the sixth intercostal space in the right midaxillary line.
Video 31-5 Pleural effusion with homogeneous echogenic pattern and mobile strands, suggestive of an exudate. Thoracentesis showed a hemothorax. The image was obtained using a 3.5-MHz transducer. The transducer was in longitudinal orientation and placed perpendicular to the chest wall to scan through the sixth intercostal space in the right midaxillary line. Access at www.fishmansonline.com
The presence of a septated effusion on ultrasonography suggests the need for fibrinolytic therapy or surgical intervention, prolonged chest tube drainage, and longer hospital stay when compared with effusions lacking ultrasound complexity (Fig. 31-5; Video 31-6).15 Pleural ultrasound is superior to CT scanning in detecting pleural fluid complexity.16
Figure 31-5 Multiseptated pleural effusion. The pattern is consistent with a complex parapneumonic effusion or empyema that will likely require fibrinolytic treatment or surgical drainage. The image was obtained using a 3.5-MHz transducer. The transducer was in longitudinal orientation and placed perpendicular to the chest wall to scan through the fourth intercostal space in the left midaxillary line.
Video 31-6
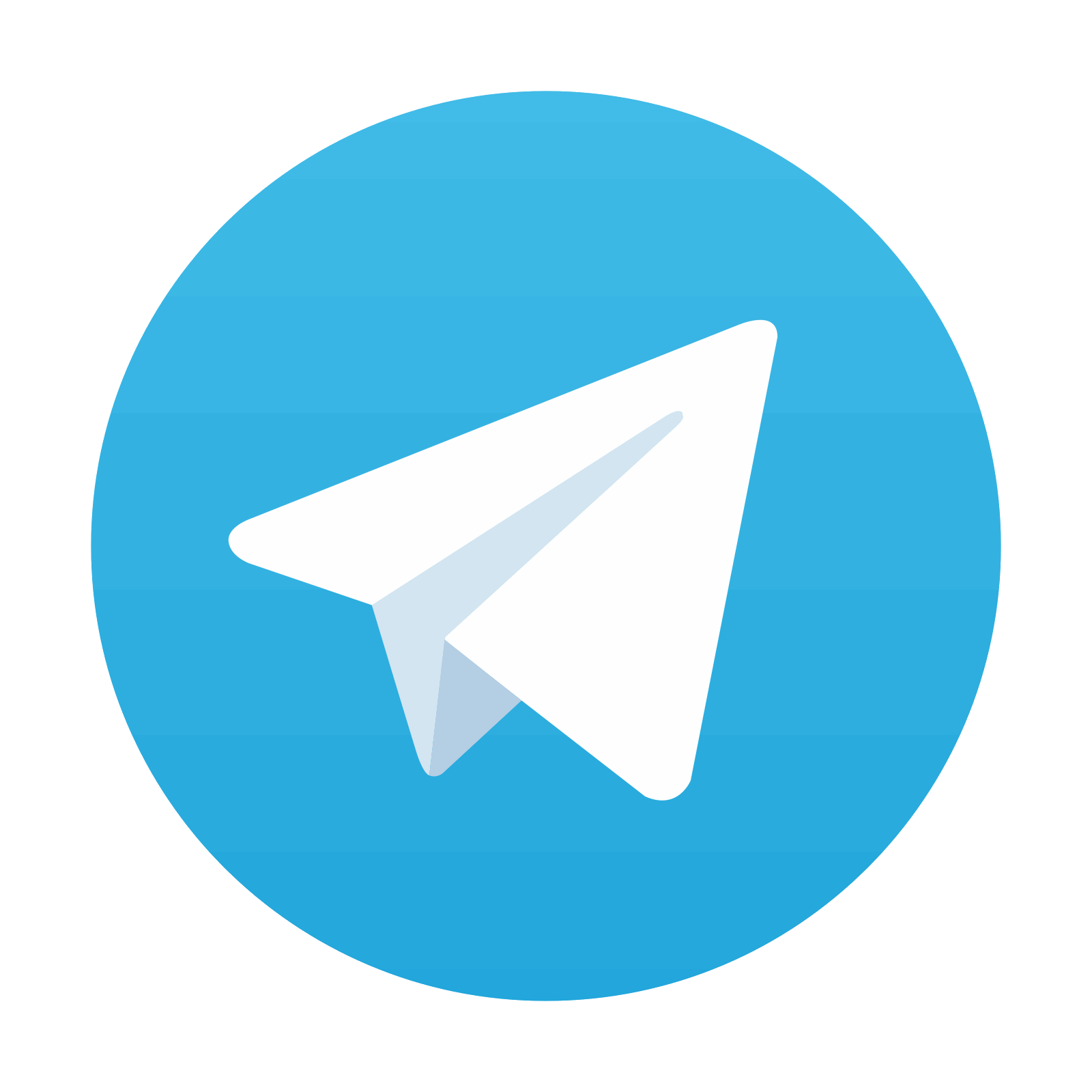
Stay updated, free articles. Join our Telegram channel

Full access? Get Clinical Tree
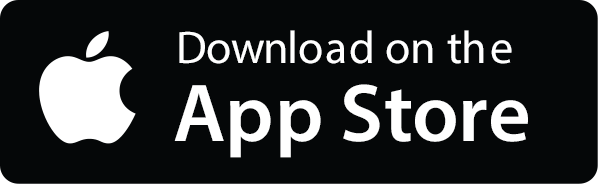
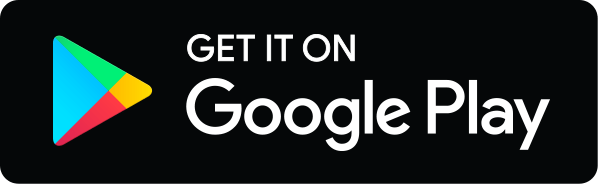