Aneurysms
- Ascending aortic diameter > 4 cm on imaging study.
- Descending aortic diameter > 3.5 cm on imaging study.
In the ascending aorta, aneurysms tend to take on three common patterns, as indicated in Figure 37–1. These include the supracoronary aortic aneurysm, annuloaortic ectasia (marfanoid), and tubular diffuse enlargement.
The most common pattern is that of supracoronary dilatation of the ascending aorta. In this pattern of disease, the short segment of aorta between the aortic annulus and the coronary arteries remains normal in size. Sinuses are “preserved,” meaning that the aorta indents normally, forming a “waist,” near the level of the coronary arteries. For this type of aneurysm, a supracoronary tube graft suffices.
In the second type, annuloaortic ectasia, the aortic annulus itself becomes dilated, giving a shape to the aorta like an Erlenmeyer chemistry flask. In this type of disease, the segment of aorta between the annulus and the coronary arteries is diseased, dilated, and thinned. Sinuses are “effaced,” meaning that the normal indentation, or waist, is lost. When surgery is required, the entire aortic root must be replaced.
In the third type of ascending aortic disease, the configuration is midway between the previous two patterns, that is, there is some dilatation of the annulus and root and some effacement of the sinuses, but these elements are not dramatic. The overall appearance is that of a large tube, rather than a flask. For such aortas, either supracoronary tube grafting or aortic root replacement may be appropriate.
The Crawford classification (Figure 37–2) is used to categorize the appearance of an aneurysm in the descending aorta and thoracoabdominal aorta. This classification is based on the longitudinal location and extent of aortic involvement, has implications for surgical strategy, and affects the risk of perioperative complications.
Type I aneurysms involve most of the thoracic aorta and the upper abdominal aorta. Type II aneurysms, the most extensive and most dangerous to repair, involve the entire descending and abdominal aortas. Type III aneurysms involve the lower thoracic and abdominal aortas. Type IV aneurysms are predominantly abdominal but involve thoracoabdominal exposure because of the proximity of the upper border to the diaphragm.
The genetics of Marfan disease, a well-known cause of aneurysms of the thoracic aorta, have been well delineated, with over 600 mutations identified at one locus on the fibrillin gene.
Increasingly, it is being appreciated that patients who do not have Marfan disease also manifest familial clustering of thoracic aortic aneurysms and dissections. Patients with aneurysms often answer one or both of the following questions affirmatively: “Do you have any family members with aneurysms anywhere in their bodies? Did any of your relatives die suddenly or unexpectedly of apparent cardiac causes?” Detailed construction of family trees on over 500 patients with thoracic aneurysm has indicated that 21% of aneurysm probands have a first-degree relative with a known or likely aortic aneurysm. The true number is certainly much higher, as these estimates are based only on family interview and not on head-to-toe imaging of relatives. Figure 37–3 shows the 21 positive family trees of the first 100 families analyzed. The most likely pattern of inheritance appears to be autosomal dominant with incomplete penetrance. A more recent analysis has shown that the location of the proband’s aneurysm largely influences the location of the aneurysms in the family members. If the proband has an ascending aneurysm, the likelihood is that the family members have ascending aneurysms. If, however, the proband has a descending aneurysm, it is likely that the family members have abdominal aortic aneurysms. These proband–family member observations are in keeping with the general concept that aneurysm disease divides at the ligamentum arteriosum: Ascending and arch aneurysms represent one disease, largely nonarteriosclerotic, while descending and abdominal aneurysms represent another disease, largely arteriosclerotic.
Application of modern molecular genetic techniques is successfully making progress toward determining the specific genetic aberrations responsible for these family clusterings and for thoracic aneurysms in general. Original progress was made largely by linkage analysis of large families with multiple members affected. Now, as automated genomic analysis has become feasible and affordable, much scientific discovery has been made by direct exome sequencing. Milewicz, Dietz, Loeys, and others have succeeded in identifying specific familial aneurysm patterns and their underlying mutations (Table 37–1). Note that all of these disorders, except one, are transmitted in an autosomal dominant fashion (with decreased penetrance). Also note that the ACTA2 disorder presents with aortic dissection at small diameters. These mutation-specific categorizations will soon allow personalized medicine based on the specific underlying mutation and its accompanying rupture and dissection patterns.
Classification | Chromosome | Gene | Protein | Location | Frequency | Inheritance |
---|---|---|---|---|---|---|
Syndrome | ||||||
Marfan | 15q21.1 | FBN1 | Fibrillin 1 | ECM | 1:5000-10,000 | Dominant |
Loeys-Dietz | 3p24-25 9q33-34 | TGFBR2, TGFBR1 | TGFβ-R2 TGFβ-R1 | Cell surface | Rare | Dominant |
Ehlers-Danlos | 2q24.3-31 | COL3A1 | Type III collagen | ECM | 1:10,000-25,000 | Dominant |
ATS | 20q13.1 | SLC2A10 | GLUT10 | Intracellular | Rare | Recessive |
AOS | 15q22.2-24.3 | Smad3 | SMAD3 | Intracellular | Rare | Dominant |
TGFB2 | 1q41 | TGFB2 | TGFβ2 | Intracellular | Rare | Dominant |
Non-Syndromic | ||||||
TAAD2 | 3p24-25 | TGFBR2 | TGFβ-R2 | Cell surface | ∼3 % of TAA | Dominant |
TAAD4 | 10q23-24 | ACTA2 | Actin | Intracellular | 10-15% of TAA | Dominant |
TAAD5 | 9q33-34 | TGFBR1 | TGFβ-R1 | Cell surface | ∼2 % of TAA | Dominant |
TAAD-PDA | 16p12-13 3q21.1 | MYH11 MYLK | β-MHC MLCK | Intracellular Intracellular | 1-2% of TAA ∼1% of TAA | Dominant |
Examination of single nucleotide polymorphisms (SNPs) in the blood of hundreds of patients with thoracic aortic aneurysms via genome-wide surveys using large (> 30,000) SNP libraries has been accomplished. An “RNA signature” in the blood of patients with thoracic aortic aneurysm was found, which can predict with about 85% accuracy from a blood test alone whether the patient harbors a thoracic aortic aneurysm. This “signature” is composed of specific RNAs that are either markedly upregulated or markedly downregulated in aneurysm patients, compared with healthy controls.
Patients who have a genetic predisposition for aneurysm development, specifically those patients with annuloaortic ectasia or ascending dissection, are significantly protected from arteriosclerosis (Figure 37–4). Remarkably, they have less arterial medial thickness and less calcification than normal controls. It appears likely that the same mutations that promote lysis of the aortic wall also prevent plaque build-up.
Figure 37–4.
Difference in overall calcification scores relative to the control group for all risk factors analyzed. Note that patients with ascending aortic dissection or annuloaortic ectasia are significantly “protected” from arteriosclerosis, manifesting lower calcification scores. AAE, annuloaortic ectasia; Age, age (in 10-year intervals); Dis, ascending aortic dissection; DM, diabetes mellitus; Dyslip, dyslipidemia; G, male gender; HTN, hypertension; Smoke, smoking history.
Accepting that most patients with aneurysms have an underlying genetic predisposition to the condition, how does this genetic programming lead to the development of an aneurysm? Rapid progress is being made in elucidating these mechanisms. Aneurysm formation is currently thought to involve the following processes (Figure 37–5): extracellular matrix proteolysis, chronic inflammation, cytokine activity, and smooth muscle cell loss. The identification of these mechanisms raises the intriguing possibility of interfering pharmacologically with this pathophysiology, so aneurysm formation or progression can be stopped. The importance of the transforming growth factor-β (TGF-β) pathway in aneurysm formation has been demonstrated; the ability of angiotensin receptor–blocking medications (eg, losartan) to interfere with this pathophysiologic mechanism is being tested, and results of randomized, controlled studies will soon be available. At this time, however, it may be said that no specific pharmacologic strategy exists for delaying aneurysm progression. Results of trials of proteolytic antagonists and β-blockers have been underwhelming. The potential roles of statin medications, anti-inflammatory agents (cyclooxygenase [COX]-2 inhibitors), immunosuppressants (sirolimus), and antibiotics (doxycycline) are being investigated.
The proteolytic enzymes called matrix metalloproteinases (MMPs) are receiving extensive attention in aneurysm pathophysiology. These powerful enzymes are found in excess in thoracic aortic aneurysms (Figure 37–6) and are thought to play a major role in destroying the substance of the aortic wall, leading to decreased wall strength and, ultimately, dilatation and rupture.
The biologic changes in the aortic wall discussed earlier are vitally important, but hemodynamic forces need to be considered as well. As the ascending aorta reaches a diameter of 6 cm, the distensibility vanishes, so that the aorta becomes essentially a rigid tube (Figure 37–7). Because of this rigidity, the force of systole can no longer be beneficially dissipated by elastic expansion of the aorta, and this translates into increased wall stress. Especially at high blood pressures, this wall stress becomes excessive, setting the stage for disruption of the aortic wall via rupture or dissection. It is instructive to note how closely this mechanical data dove-tail with the clinical behavior of the aorta: The mechanical properties deteriorate at 6 cm diameter, and that is precisely the hinge point for clinically manifest rupture and dissection.
Figure 37–7.
A: Distensibility values in normal aortas and aortic aneurysms of different diameters. Distensibility of ascending aortic aneurysms decreases rapidly as diameter increases, to very low values at dimensions > 6 cm. At 6 cm, the aorta is essentially a rigid tube, unable to dissipate the force of systole by expanding phasically during the cardiac cycle. B: Exponential relationship between wall stress and aneurysm size in ascending aortic aneurysms. The dark columns represent a blood pressure of 100 mm Hg, and the light columns represent a blood pressure of 200 mm Hg. The lines at 800–1000 kPa represent the range of maximum tensile strength of the human aorta. Note that a patient with a 6-cm aneurysm and a blood pressure of 200 mm Hg (as during stress or extreme exertion) “flirts” with the limits of the ultimate strength of his or her aorta wall.
The Yale computerized database now contains information on nearly 3000 patients with thoracic aortic aneurysm, including some 9000 tabulated serial imaging studies and 9000 patient-years of follow-up. This database and these methods of analysis have permitted assessment of multiple fundamental topics and questions regarding the natural behavior of the thoracic aorta and have shed light on appropriate criteria for surgical intervention.
Via specifically developed statistical methods designed to account for important potential sources of error, the annual growth rate of an aneurysmal thoracic aorta has been determined to be 0.12 cm on average. The descending aorta grows faster than the ascending aorta, at 0.19 cm/year compared with 0.07 cm/year. Also, the larger the aorta becomes, the faster it grows.
Critical to decision making in aortic surgery is an understanding of when complications occur in the natural history of unrepaired thoracic aortic aneurysms. In the case of the thoracic aorta, the two complications that are vitally important are rupture and dissection. Knowing when these complications are likely to occur would permit rational decision making regarding elective, preemptive surgical intervention to prevent their occurrence.
Size criteria apply only to asymptomatic aneurysms. We are learning increasingly that the aorta can indeed “communicate” with us—via pain. Symptomatic (painful) aneurysms should be resected regardless of size. For ascending aneurysms, this pain is usually felt anteriorly, under the breastbone. For descending thoracic aneurysms, the pain is usually felt in the interscapular region of the upper back. For thoracoabdominal aneurysms, the pain is usually felt lower in the back and in the left flank. Other symptoms may occasionally be produced by thoracic aortic aneurysms, including bronchial obstruction, esophageal obstruction, and phrenic nerve dysfunction; these symptoms also constitute indications for surgical intervention.
Initial statistical analysis revealed sharp “hinge points” (Figure 37–8) in aortic size at which rupture or dissection occurred. For the ascending thoracic aorta, the hinge point occurs at 6.0 cm. By the time aortas reach this size, 31% have ruptured or dissected. For the descending aorta, the hinge point is located at 7.0 cm. By the time descending aortas reach this size, 43% have ruptured or dissected.
Figure 37–8.
The “hinge points” (arrows) in the cumulative, life-time incidence of complications (rupture or dissection) of thoracic aortic aneurysms, based on size. By the time the aorta reaches the dimensions on the x-axis, the percentage of patients shown on the y-axis has already incurred rupture or dissection. A: Curve for the ascending aorta. B: Curve for the descending aorta.
If a surgeon were to wait for the aorta to achieve the median size at time of complications in order to intervene, by definition rupture or dissection would have occurred in half of the patients (Figure 37–9). Accordingly, it is important to intervene before the median value is attained. The following recommendations take this factor into account, permitting preemptive surgical extirpation before rupture or dissection in most patients.
Figure 37–9.
A schematic representation of the importance of selecting a criterion for intervention before complications (rupture or dissection) commonly occur. Utilization of the median as the criterion level would allow half the population to realize a devastating complication before preemptive intervention. Accordingly, a criterion below the median is selected (arrow) to allow preemptive intervention before a large proportion of patients have suffered a complication.
Current recommendations are listed in Table 37–2 and are based on the hinge points noted in Figure 37–8. Specifically, prophylactic extirpation of the aneurysmal ascending aorta is recommended when the aneurysm measures 5.5 cm; for the descending aorta, which does not rupture until a larger size, surgical intervention is recommended when the aneurysm measures 6.5 cm. Application of these criteria will prevent most ruptures and dissections, without prematurely exposing the patient to the risks and inconveniences of surgery. The efficacy of this management algorithm has recently been documented in a large cohort of prospectively followed patients.
It is well-known that patients with Marfan disease are prone to unpredictable dissection at an early size. For this reason, earlier intervention is recommended for patients with Marfan disease as indicated in Table 37–2.
For patients with a positive family history, but without Marfan disease, the same criteria are applied as for Marfan disease, because malignant early behavior of the aneurysm in these patients may be seen as well.
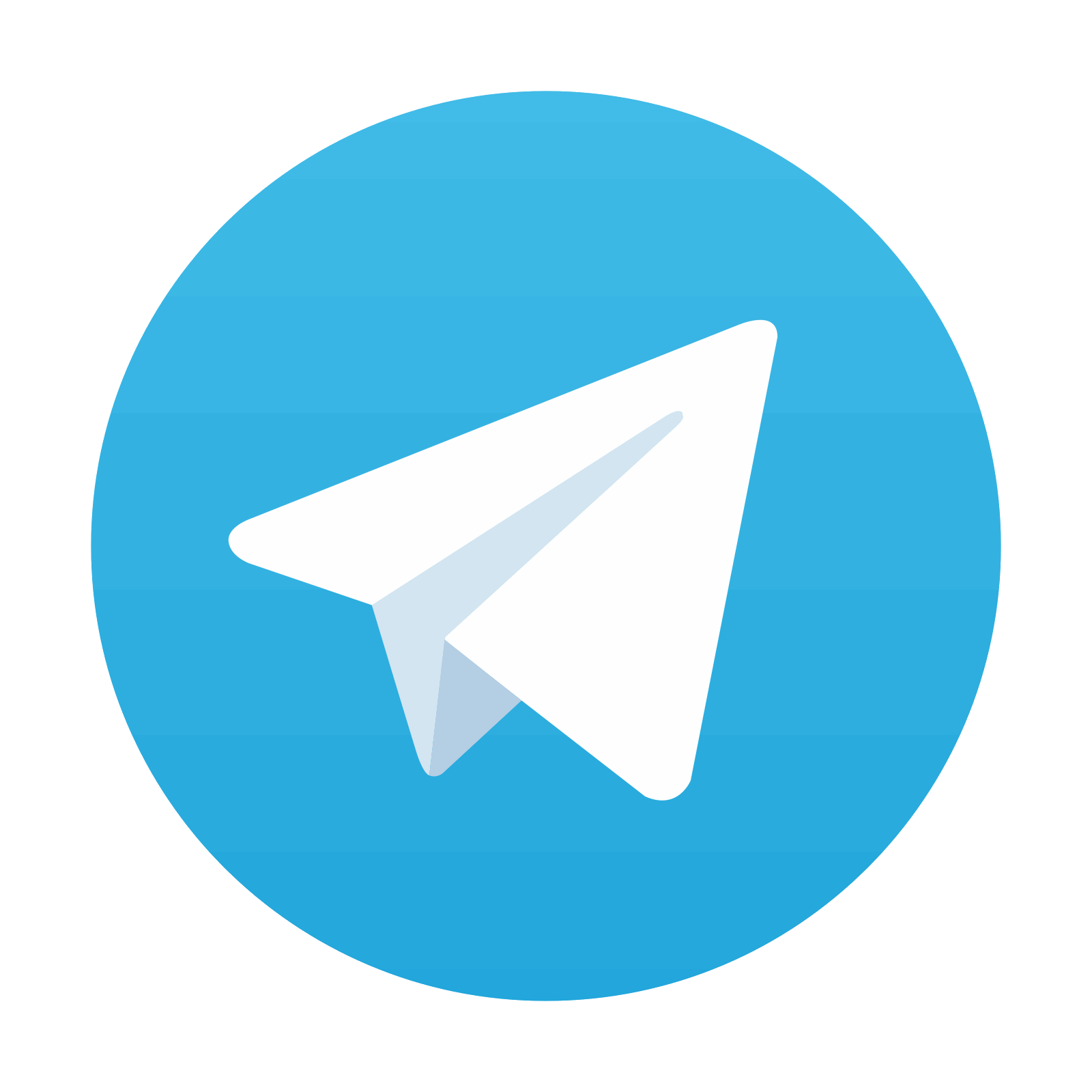
Stay updated, free articles. Join our Telegram channel

Full access? Get Clinical Tree
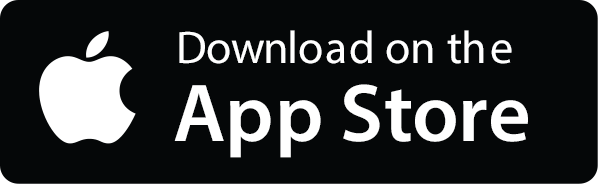
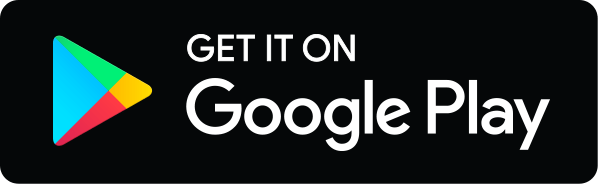