Chapter 135
Thoracic and Thoracoabdominal Aneurysms
Open Surgical Treatment
Charles W. Acher, Mimi Wynn
Based on a chapter in the seventh edition by Michael J. Jacobs and Geert Willem Schurink
The surgical repair of descending thoracic aortic aneurysms (DTAAs) and especially thoracoabdominal aortic aneurysms (TAAAs) remains one of the major challenges in vascular surgery. The technical demands of the surgery may require the reattachment of brachiocephalic, visceral, renal, and intercostal arteries to a synthetic graft or aortic homograft, and the physiologic stress of the surgery can result in stroke, renal failure, cardiac or pulmonary failure, and paraplegia leading to significant disability, decline in quality of life, and death. The first successes in treating thoracic aortic disease were by Crafoord, Gross, and Etheridge. The difficulty of thoracic aortic surgery was documented early in aortic surgical history by Adams’ 1956 landmark paper on paraplegia causation and risk in thoracic aortic surgery, in which he described the anatomy of spinal cord blood flow and the high mortality and paralysis risk of 25% in attempts at just thoracic aortic repair. Ten years later, DeBakey reported 50% mortality in his first small series of TAAA repair.1,2
The modern era of surgical treatment of TAAA began with E. Stanley Crawford, who in his first published series in 1974 reported significant reductions in mortality and paraplegia rates accomplished without assisted circulation (cardiopulmonary bypass). With his lifetime experience of more than 1500 TAAAs, achieving an overall mortality of 8% and paralysis risk of 16%, Stanley Crawford set the standard for a generation of surgeons.3
In spite of improved outcomes reported from major centers in the modern era with mortality and paraplegia rates of less than 7% in elective operations,4 high mortality and morbidity continue to occur as demonstrated in two administrative database studies that documented 20% or higher elective mortality and equally high paraplegia rates in smaller, lower volume centers attempting treatment of these challenging complex aneurysms.5,6 This chapter explores how the different pathologic processes encountered affect the surgical approach and treatment indications, the mechanics of repair, and how experienced centers have been able to reduce morbidity and mortality of open surgical repair. To understand paraplegia and mortality risk more comprehensively we use observed/expected (O/E) ratios for paraplegia calculated from a previously published predictive model that uses clinical factors (extent of aortic replacement, clinical acuity, and dissection) to compensate for differing populations in clinical reports.7 We also use data from our experience treating more than 1000 patients and a database of more than 15,000 patients from published reports that we have analyzed and added to for the last 20 years.
Pathology
Aneurysm causation and pathologic classification are discussed in Chapter 134. It is important, however, for the surgeon to understand the pathologic process involved in an individual patient because it can affect technical considerations in thoracoabdominal aneurysm repair. This wide spectrum of pathologic and clinical presentations demands that the anesthesia and surgical teams be well versed in the pathologic process and the variations these patients present in technical repair and operative risk if successful outcomes are to be achieved.
Degenerative
Degenerative aneurysms are the most common TAAAs, usually occurring in older patients who have atherosclerosis and smoked. The pathogenesis is complex and associated with the inflammation of atherosclerosis and the individual’s response to injury through matrix metalloproteinases and collagen remodeling of the aorta.8–13 Degenerative aneurysms are more likely to be calcified to varying degrees and may have a damaged and poorly functioning intimal surface covered with debris from plaque and old thrombus as well as plaque stenosis or occlusion of branch vessels, which make repair and revascularization more difficult. These older atherosclerotic patients may also have significant cardiac, pulmonary, and renal comorbidities as well as the infirmities of advanced age, significantly increasing treatment risk. Although it is not common, the inflammatory response in degenerative aneurysms can be so severe that they fall into a category called inflammatory aneurysms, in which the periaortic structures, like branch vessels, ureters, bowel, or mediastinal structures, are encased in a dense retroperitoneal fibrosis that makes surgery especially difficult.14,15
Inheritable
The known heritable diseases like Marfan, Ehlers-Danlos, and Loeys-Dietz syndromes16–19 are defined by known genetic mutations that create abnormalities in elastin and collagen and transforming growth factor-β receptors that weaken the aortic wall, leading to aortic aneurysms and dissections, which usually occur in younger patients. It is also increasingly apparent that similar mutations account for familial aneurysms and other noncategorized patients predisposed to aneurysms and aortic dissection (Fig. 135-1).20–24 The quality of the aortic tissue with which the surgeon is required to work may vary within a group, depending on the severity of the structural defect and differences between pathologic groups, with Ehlers-Danlos and Loeys-Dietz patients having more fragile aortas than Marfan patients.25,26 These differences may require different surgical techniques with less traumatic vascular clamps, use of hypothermic circulatory arrest to avoid clamping, or use of aortic wall reinforcement with felt pledgets or strips to secure the suture line because of tissue fragility. In some patients, for example, those with severe types of Ehlers-Danlos syndrome, surgical repair may not be a viable option27,28 (see Chapter 143).
Figure 135-1 A and B, A very large thoracoabdominal aneurysm in a 32-year-old woman (5’4”, 110 pounds) with no family history or stigmata of Marfan, Loeys-Dietz, or Ehlers-Danlos syndromes and no elevated inflammatory markers. The patient presented with vague chest pain and fatigue. C, At repair, the proximal neck was wrapped with a Dacron sleeve to prevent future expansion. Patients like this are undefined genetically but obviously have some molecular defect, which more than likely will be defined within the next decade from research efforts like the National Registry of Genetically Triggered Thoracic Aortic Aneurysms and Cardiovascular Conditions (GenTAC).
Trauma, Mycotic Aortitis, and Vasculitis
Clinical presentation also poses significant challenges for the surgeon. In trauma with multiple injuries or mycotic aortitis, treatment requires radical aortic and periaortic débridement with in situ repair (Fig. 135-2) or aortic oversewing with extra-anatomic bypass in desperate situations.29
Figure 135-2 The fundamental principle in treatment of mycotic thoracoabdominal aneurysm is radical débridement of all infected aortic and periaortic tissue as seen here in a patient who developed a mycotic thoracoabdominal aneurysm from an Escherichia coli urinary tract infection and required débridement of the distal third of the thoracic and all of the abdominal aorta with in situ aortic reconstruction by a Teflon graft.
Thoracoabdominal aneurysm associated with the vasculitis of rheumatoid diseases (ankylosing spondylitis)30–32 or the vasculitis of Takayasu’s disease, lupus erythematosus, or giant cell arteritis, although uncommon, may have associated branch vessel occlusive disease and cardiac and pulmonary involvement that can complicate repair.33–35
Anatomic Classification
Aneurysms of the thoracoabdominal aorta are classified anatomically (see Chapter 134) primarily by extent of aortic involvement, which is associated with the surgical complexity of repair. Crawford type II aneurysms have the greatest paralysis risk, followed by Crawford types I, III, and IV and thoracic aortic aneurysms (DTAAs), which are confined to the distal arch and descending thoracic aorta and have the lowest paralysis risk from surgery.36
Aortic dissections have a different classification based on origin and extent of the dissection and the need for immediate surgery (Stanford A) and those historically treated medically with antihypertensives that originate in the distal arch or more commonly in the descending thoracic aorta at or near the ligamentum arteriosum and left subclavian artery (Stanford B) (see Chapter 138). These classifications capture most but not all of the variation seen clinically and in general indicate increased risk of stroke and paralysis with repair.37–39
Therefore, when a TAAA is described as Crawford type II in a patient with a Stanford type A or B dissection, the mental image is an aneurysm extending from distal arch or proximal descending thoracic aorta to at or near the iliac arteries from an aortic dissection that has expanded to become aneurysmal. This is in contrast to a Crawford type I aneurysm with a Stanford type B dissection that has a dissection to the iliac arteries or further, but the aneurysm extends only to the visceral arteries.
Indications for Surgical Treatment
The primary indication for treatment of aortic aneurysms is rupture risk, which is generally considered to correlate with aortic diameter in accordance with Laplace’s law (see Chapter 9). The larger the aneurysm and the thinner the wall, the more likely it is to rupture, which usually results in death. Some TAAAs become very large (>10 cm) before rupture and invariably have thick walls that allow such unusual size before structural failure occurs. However, there is considerable variation in TAAA diameter at the time of rupture or other acute event (dissection, plaque hemorrhage), which in our experience averages 8.2 ± 2.8 cm (median, 7.4 cm), with only 13% of all ruptures occurring below 6 cm and rarely below 5.5 cm. This is analogous to abdominal aortic aneurysms: prospective trials have confirmed the low rupture risk of aneurysms up to 5.5 cm diameter (<1.0%/y).40–42
Griepp, Elefteriades, and others have performed extensive research on the natural history of thoracic aneurysms.43–49 These natural history studies indicate that besides diameter and pain, age, the presence of chronic obstructive pulmonary disease or renal insufficiency, the extent of the aneurysm, and the expansion rate of the aneurysm are independent risk factors for rupture.50–57 The growth rate for DTAA (0.42 cm/y) found by Hirose was the same as the growth rate for abdominal aortic aneurysms found in the Aneurysm Detection and Management (ADAM) trial.41,58
The dilemma in TAAA repair, because of the significantly greater surgical risk than in infrarenal abdominal aortic aneurysm repair, is if and then when to treat. Although the data in anecdotal reviews and single-institution reports support the view that TAAAs have growth and rupture risks similar to those of infrarenal abdominal aortic aneurysms, most aortic surgeons put the threshold for risk/benefit at about 6 cm from natural history information weighed against surgical risk and long-term survival.44,48,59–63 It is known from these observational studies that 40% to 60% of patients die of other causes before rupture, making the decision for aneurysm treatment much more complex than just natural history, physics, and molecular biology. Further, individual patients have differing treatment and rupture risks related to pathologic changes, age, comorbidities, and personal preference.
Other indications, such as occlusive or aneurysmal disease of branch vessels (mesenteric or renal ischemia; brachiocephalic, visceral, or iliac aneurysms) may lead to treatment of smaller TAAAs. Similarly, acute events of aortic plaque rupture, mycotic aortitis, trauma, renal failure from obstructive uropathy in inflammatory aneurysms, or malperfusion in acute dissection may require aortic repair or bypass with little aneurysmal dilatation. Some patients have significant aneurysm enlargement in the ascending aorta and arch (see Chapter 138) in addition to the thoracoabdominal aorta that requires staged repair, which presents unique technical challenges. In addition to all these factors, new dilemmas are created because of new and advancing endovascular technologies (see Chapter 136), which may require surgical rescue because of failure or surgery to treat aneurysm progression above or below previously placed endografts. The surgeon may also decide to stage or to combine open and endograft repair for ascending arch and descending aneurysm repair.
Patient Evaluation
The decision to treat begins with and is clarified by evaluation of cardiac, pulmonary, renal, and overall vitality factors to establish the patient’s fitness for elective surgery (see Chapter 134). This evaluation is expensive and time-consuming for patients and families but is important in discussing operative risk.
Imaging
Aortic and branch vessel imaging primarily with magnetic resonance imaging and computed tomographic angiography is important to identify the extent of disease for preoperative planning (see Chapter 134). The improved resolution and quality of imaging that has occurred during the last 15 years allows identification of patent intercostal arteries of interest, detailed understanding of complex dissection anatomy, and evaluation of plaque morphology. A newer and exciting imaging technique is four-dimensional magnetic resonance angiography (Fig. 135-3). With this magnetic resonance imaging technique, detailed anatomy and flow dynamics like velocities, flow rates, wall stress, and pressure gradients can be measured noninvasively, which increases our clinical understanding of the effects of treatment as well as the complex flow and stress dynamics in aneurysms and aortic dissection.64–66
Figure 135-3 Color rendering of velocity and flow patterns in a type B aortic dissection by four-dimensional magnetic resonance imaging. Flows, velocities, wall stress, and pressure gradients can be accurately measured with this imaging technique. Such hemodynamic information, acquired noninvasively, will allow researchers and treating physicians better understanding of flow dynamics and stresses in these complicated aneurysms. Velocity is represented by color (red, yellow, green, blue, from highest to lowest).
Magnetic resonance angiography can be used to localize intercostal or lumbar arteries that may be considered for reimplantation in patients with extensive aneurysms and many open intercostal arteries (higher paraplegia risk).53 This localization is inexact because of false positives for intercostal patency, and the final decision on which arteries, if any, to reimplant is made after opening the aneurysm and confirming the preoperative imaging or selecting intercostal arteries by patency and location between spinal levels T8 and L2, where the greater radicular artery arises 85% of the time. As discussed in the outcomes section later, intercostal reimplantation or preservation is not the most important factor in paraplegia prevention but may reduce the risk of delayed paraplegia in especially vulnerable patients with extensive aneurysms and many open intercostal arteries.
Informed Consent
Informed consent for complex high-risk surgeries for life-threatening diseases is a difficult and nuanced process that should give patients a realistic understanding of what you face as the surgeon and what they face as the patient, which must include the worst case scenario along with the best if the patient is to make a decision consistent with the goals in treatment.67–69 Especially because of the risks of paraplegia, stroke, and renal failure in addition to possible ventilator dependence in marginal patients facing DTAA or TAAA surgery, the consent conversation has to be a realistic discussion of probability and uncertainty surrounding surgery and what life will be like if the surgical effort fails to return the patient to preoperative functional status. The older the patient, the more selective both surgeon and patient should be about treatment.
Surgical Repair
Once the decision for surgery has been made, the surgeon and anesthesiologist have to develop and execute the plan for repair. That plan will depend on anatomy and pathologic conditions that can vary greatly between patients, requiring the surgeon and anesthesiologist to adapt. In addition to patient variation, surgeons may favor different surgical approaches ranging from simple cross-clamping (Crawford technique) with adjunctive measures to protect the kidneys and spinal cord to assisted circulation (atrial-femoral or femoral-femoral bypass) or hypothermic circulatory arrest.
Extracorporeal Circulation
Assisted circulation is usually atrial-femoral (partial heart bypass) with oxygenated blood coming from a left atrial or left pulmonary vein cannula to a centrifugal pump that feeds a femoral artery cannula, which provides retrograde perfusion to the pelvic and visceral arteries during proximal repair. The bypass flow rate is adjustable so that proximal and distal arterial perfusion pressures can be maintained, and it is an effective technique to offload the heart, reducing cardiac strain with very proximal aortic clamping. This technique is dependent on the heart and lungs for oxygenation and cerebral circulation but does not require full systemic heparinization.
Hypothermic circulatory arrest requires complete cardiopulmonary bypass with arterial perfusion cannulas in the femoral artery or ascending aorta and venous return through a femoral vein cannula, which usually extends to the right atrial level and superior vena cava and is placed with a guide wire and transesophageal echocardiography to confirm location. A left ventricular sump drain is required to prevent cardiac dilatation and pulmonary congestion during cardiopulmonary bypass. Patients are usually cooled to 16° C to 18° C, and then circulation is arrested during the proximal anastomosis, after which cerebral perfusion is resumed through a side arm on the aortic graft while the remaining aorta is reconstructed. Hypothermic circulatory arrest is a complex procedure because of longer pump runs and coagulopathy, with bleeding made worse by the large surface area of a thoracoabdominal incision. Profound hypothermia provides excellent organ protection during aortic reconstruction; however, because of its complexity, this technique is used the least.
Other Perfusion Techniques
Distal aortic perfusion and selective perfusion of the mesenteric arteries can be achieved without pump devices. The Gott shunt and external axillofemoral artery bypass have been used as a passive bypass circuit for repair of DTAA and TAAA.70,71 Cambria described an in-line mesenteric shunt technique that restores pulsatile flow to the mesenteric circulation within 25 minutes, even in the absence of distal aortic perfusion. This technique is used in combination with renal artery perfusion with 4° C renal preservation solution.72 Cambria, however, has abandoned epidural cooling because of poor results. Each technique has advantages and disadvantages, as discussed later in the outcomes section.
Our approach is to simplify the surgery as much as possible but to vary the surgical technique according to the anatomy and pathologic process. Therefore, most of the time, we use Crawford’s technique of simple cross-clamping with adjuncts (hypothermia, spinal fluid drainage, steroids, endorphin receptor antagonists, and optimization of perfusion pressure and cardiac function) that Crawford did not use to protect the spinal cord, kidneys, and other organs.73 When anatomy dictates (no place to proximally clamp the aorta or occasionally in acute dissection), we use hypothermic circulatory arrest (Fig. 135-4). Our surgical approach is based on experimental studies of the effects of aortic occlusion and preservation of spinal cord, renal, and other organ function. Later in this chapter, we will show how experimentally validated protective adjuncts work regardless of surgical technique and that equally good results can be obtained with all techniques if experimentally demonstrated protective principles are followed.
Figure 135-4 This 35-year-old patient required hypothermic circulatory arrest for repair of a thoracoabdominal aneurysm because there was no place to clamp the aorta. His aneurysm was caused by a type A dissection (repaired acutely) from aortic disease associated with ankylosing spondylitis, a rare cause of aortic disease.
Anesthesia
The anesthesia for TAAA repair is complex, requiring multisystem monitoring and control. Arterial lines in the upper (radial artery) and lower (femoral artery) extremities, pulmonary artery catheter for monitoring cardiodynamics (cardiac index, central venous pressure, pulmonary artery pressures), and transesophageal echocardiography to evaluate ventricular volume and function and valve function are used to optimize mean arterial pressure (MAP) and cardiac function. Electroencephalography monitors central nervous system activity when barbiturates are used to reduce oxygen demand74 before and during aortic occlusion. Blood and nasopharyngeal temperature are monitored because these correlate with spinal fluid and brain temperature. Coagulation parameters, blood chemistries, and arterial blood gases are closely monitored throughout surgery. A 16F Medtronic lumbar spinal drain is fluoroscopically placed in most patients to monitor and control spinal fluid pressure by draining spinal fluid to improve spinal cord blood flow during aortic occlusion.75 The anesthesiologist uses vasoactive medications to control arterial pressure and maximize cardiac function. Arterial dilators, such as nitroprusside and hydralazine, are avoided because of their experimentally demonstrated “stealing” effect on spinal cord blood flow.76 Infusion of sodium bicarbonate (0.05 mEq/Kg/min) while the aorta is occluded above the renal arteries prevents systemic acidemia. Our group administers methylprednisolone after induction, and naloxone (1 µg/Kg/h) is infused during and after the procedure.77,78 Mannitol is given before and during aortic occlusion. When one-lung ventilation is required in extensive proximal aortic replacement, we use an Arndt endobronchial blocker, bronchoscopically placed with a guide wire,79 which we think is easier and safer than a double-lumen endotracheal tube. This technique provides excellent lung isolation without the need to change endotracheal tubes at the end of surgery. Moderate systemic hypothermia is used to prolong spinal cord and organ ischemic tolerance. Aggressive blood component replacement is important to prevent coagulopathy.
During the surgical exposure, the anesthesiologist cools the patient (34° C or lower) with a cold operating room and intravenous fluids. As the time for aortic clamping approaches, our anesthesiologists drain enough spinal fluid to achieve a spinal fluid pressure less than 6 mm Hg during aortic occlusion. Timing this requires close communication between the surgeon and anesthesiologist. If assisted circulation (cardiopulmonary bypass, circulatory arrest, or atrial-femoral bypass) is used, we complete the exposure dissection before cannulation and heparinization to minimize bleeding. These assisted circulation patients require additional exposure, depending on surgeon preference for cannulation of the femoral artery and vein, left ventricle apex, ascending aorta, left atrium, or pulmonary veins.
During surgical reconstruction, the anesthesiologist is equally busy keeping the patient volume resuscitated with blood reclaimed from the high-speed Cell Saver, red blood cells, and fresh frozen plasma to replace coagulation factors from blood loss. The anesthesiologist’s goal is to optimize tissue oxygen delivery, intravascular volume, cardiac function, and arterial perfusion pressure (MAP > 90 mm Hg) in addition to keeping the spinal fluid pressure low without draining excessive fluid. Unless the anesthesiologist is aggressive and proactive in managing cardiac function and fluid resuscitation and in preventing coagulopathy, hypovolemia, and hypotension, the patient will have increased risk of coagulopathy, paraplegia, renal failure, cardiac ischemia, and death. The anesthesiologist is as important as the surgeon in the success of these complicated aortic reconstructions.
When surgery is done with assisted circulation, the anesthetic management has to adjust primarily to prevent the proximal relative hypotension associated with left heart bypass that puts the spinal cord at increased risk because of reduced perfusion pressure to the collateral circulation. This negative effect is exacerbated if hypothermia is not used. To maintain proximal and distal MAP higher than 90 mm Hg during partial bypass requires aggressive volume replacement, inotropes, and vasopressors. This is even true in hypothermic arrest, in which the very potent spinal cord protective effects of profound hypothermia can be undone during rewarming if a low MAP is allowed and the patient is made normothermic.
Patient Positioning
For a thoracoabdominal incision, the patient is placed in a lateral decubitus position with the left side up. The shoulders are usually vertical, but the pelvis is tilted so the left femoral artery and vein can be easily accessed for aortofemoral bypass, arterial line, or cannulation if left heart bypass is planned. Extreme pelvic tilting can expose the right groin for access to those vessels (Fig. 135-5). We use an Olympic Vac-Pak patient positioner (beanbag) with inner gel pad to secure the patient in this position.
Figure 135-5 A-D, The lower the interspace of the incision, the lower the risk of pulmonary complications, so the lowest incision is chosen that allows aortic repair. Aneurysms at the arch level usually require a fifth interspace incision, whereas type III and type IV aneurysms can be repaired with an incision in the eighth or ninth interspace, with extraperitoneal exposure. As much of the latissimus dorsi and serratus anterior is preserved as possible by incising them lower than the interspace to be opened.
Surgical Exposure
The level of the incision is determined by what portion of the aorta is to be replaced. An aneurysm confined to the descending thoracic aorta may require a thoracotomy in the fifth, sixth, or seventh intercostal space, depending on the extent of aortic involvement, or occasionally in the fourth for a distal arch–proximal descending aneurysm. A Crawford type III or type IV replacement allows extraperitoneal exposure through the eighth or ninth intercostal space; but a Crawford type I or type II requires incision at the fifth or sixth intercostal space with intra-abdominal exposure, with the spleen, colon, and left kidney mobilized medially off the retroperitoneal space (Fig. 135-6). In such cases, the diaphragm is incised through the aortic hiatus, which allows compete exposure of the descending thoracic and abdominal aorta with its visceral branches and the iliac arteries if necessary (Fig. 135-7A, C). Some surgeons spare the central diaphragm and some use two incisions, a higher thoracotomy and a lower thoracoabdominal extraperitoneal incision, believing this reduces pulmonary morbidity; but this approach obscures the anatomy, making surgical repair more difficult, with only a modest decrease in prolonged ventilation (36% vs 48%) without any demonstrable decrease in mortality.80
Figure 135-6 A, In older patients and in patients with elevated body mass index, in addition to cutting the costochondral junction, the ribs are cut posteriorly to create a controlled cut instead of a fracture. After the diaphragm is incised down to the peritoneal reflection (B), the spleen, pancreas, colon, and left kidney are mobilized off the retroperitoneum (C). It is important to not dissect into the psoas fascia but open Gerota’s fascia posteriorly closer to the midline so the retroperitoneal periaortic space can be easily seen and exposed. On occasion, dissection is also anterior to the left kidney to gain access to more of the superior mesenteric artery.
Figure 135-7 A, After mobilizing renal and visceral structures off the retroperitoneal space, the diaphragm is incised through the aortic hiatus, the inferior pulmonary ligament is divided with cautery, and the periaortic lymph tissue is divided, exposing the thoracoabdominal aorta. C, We usually dissect the first few centimeters of the left renal and visceral arteries. On occasion, a retroperitoneal left renal vein is encountered (B), which we preserve by dividing it between two straight DeBakey vascular clamps and then sewing it back together after the arterial reconstruction is done.
If the patient has had previous aortic or thoracic surgery, the exposure can be more difficult because of periaortic scaring, inflammation, and adhesions, especially lung adhesions to the parietal pleura, which can be tedious to take down and can result in lung injury with bleeding or air leaks. We usually dissect and expose the celiac, superior mesenteric, and left renal arteries; but if the aneurysm is very large or is inflammatory, the mesenteric exposure may be too difficult and is avoided. In the case of associated mesenteric ischemia, the superior mesenteric artery can be exposed more completely by dissecting anterior to the kidney between the peritoneal sac and Gerota’s fascia. On occasion, the left renal vein is retroaortic, requiring the surgeon to work around it or divide it for reanastomosis after aneurysm repair (Fig. 135-7B).
Patients with aneurysms from chronic dissections or with anomalous arch anatomy present special challenges and operative planning. The patient in Figure 135-8A is an example of difficult arch anatomy because of an aneurysmal aberrant right subclavian artery in a dissected arch. Only after a right carotid subclavian transposition that was staged a month before TAAA repair could the arch be safely cross-clamped and the aneurysm repaired (Fig. 135-8B).
Figure 135-8 Marfan patient with an 8-cm Crawford type II aneurysm from a chronic dissection of an acute type A dissection presents additional challenges because of an aneurysmal aberrant right subclavian artery shown from the posterior view (arrow) (A). Right carotid subclavian transposition (arrow) was done before TAAA repair to fix the subclavian aneurysm and secure the arch for cross-clamping and TAAA repair (B). Even though the dissection runs through the arch, it is not aneurysmal and is suitable for clamping and suturing a graft with felt reinforcement.
The extent of exposure of the arch vessels depends on where the proximal aortic clamp is placed and requires avoidance of injury to the phrenic nerve and the vagus and its recurrent branch because injury to these nerves increases the risk of pulmonary complications. Patients should be educated preoperatively about recurrent nerve injury and the resulting vocal cord dysfunction, which cannot always be avoided and occurs in about 10% of patients when the proximal anastomosis is at or proximal to the left subclavian artery. Also at this level, the ligamentum arteriosum is usually divided for more complete arch exposure. On occasion, in emergencies with a large mediastinal or arch hematoma or in the case of a very large proximal aorta, the pericardium is opened to obtain easier access to the proximal arch for aortic control. The left iliac artery is easily seen and exposed, but the right may require division of the inferior mesenteric artery at its origin to mobilize the distal aorta for better access to the right iliac artery. If the inferior mesenteric artery is patent and large, it may be important for colonic perfusion and require reattachment, although this is uncommon. The ureters, especially the left, are vulnerable to injury in inflammatory aortas or “redo” surgery; in such cases, ureteral stents can be placed before surgery so that the ureters can be identified more easily. It is important to take the time to obtain complete enough anatomic exposure in anticipation of problems that might develop (such as a need for a more proximal clamp or more distal exposure) so that if the need arises, further dissection is not necessary after aortic occlusion.
Aneurysm Repair
Initial Clamping
With the simple cross-clamp surgical technique, heparin flush (5000 units/L) is used on the field. Systemic heparin is used only if patients have previous aortic grafts, and then the dose is small (3000-5000 units). When assisted circulation (AC) is used, heparin requirements are increased and activated clotting time is monitored. With complete heart bypass (hypothermic arrest), total systemic heparinization is required (400 units/Kg).
Once the exposure is complete and the systolic pressure is less than 100 mm Hg, temperature is below 34° C, spinal fluid pressure is less than 6 mm Hg, and barbiturate burst suppression is initiated, the aorta is cross-clamped and the aneurysm opened for repair. MAP is kept at 100 mm Hg while the aorta is clamped. If there are many open intercostal arteries or assisted circulation is used, a distal or distal sequential clamp technique is used to avoid what can be exsanguinating backbleeding from open intercostal and lumbar arteries. In aneurysms of just the descending thoracic aorta, we usually do not reattach intercostal arteries; but in TAAA patients before or after opening the aneurysm the intercostal arteries chosen for reimplantation by preoperative magnetic resonance angiography or patency and location at surgery are temporarily occluded with micro bulldog clamps (usually two to four arteries between T8 and L2), and the remaining intercostal and lumbar vessels are quickly oversewn to minimize blood loss and maximize collateral perfusion pressure. Similarly, Fogarty balloon catheters are used to occlude the visceral and renal arteries. At this point, the renal artery orifices are exposed, and each kidney is cooled with 300 to 400 mL of cold (4° C) renal perfusion solution (12.5 G of mannitol and 1000 units of heparin per L of lactated Ringer’s solution). This gives hypothermic renal protection and also further lowers the body temperature to 31° C to 32° C to enhance spinal cord and end-organ ischemic tolerance. This sudden volume infusion increases spinal fluid pressure, which may require more drainage to keep the spinal fluid pressure low.
Proximal Anastomosis
After completion of this portion of the operation, the proximal graft aortic anastomosis is performed using 2-0 Prolene on a large CT-1 needle most of the time. Depending on the tissue integrity (dissection, calcium requiring aortic endarterectomy, or just a fragile thin aortic wall), a finer suture or needle (3-0 suture or SH needle) or felt strips or pledgets may be used to reinforce the anastomosis. An important technical point is that all anastomosis should be done so the aorta is not repeatedly cross-clamped to repair leaks. This requires a little more time but makes for a faster, less traumatic anastomosis. After completion of the proximal anastomosis, the patient is put in Trendelenburg position, and the anastomotic integrity is tested by placing a vascular clamp on the graft after flushing (to remove air and thrombus that can cause a stroke through the arch vessels) and removing the proximal clamp. If there is a leak requiring attention, we usually repair this with a pledgeted stitch without reclamping the aorta. In thoracic aortic aneurysm patients, the distal anastomosis is completed, and flushing of air and atheromatous debris is done in a similar manner.
Visceral-Renal Reattachment
The next stage of the repair is visceral and renal artery reattachment, which is usually done with a Carrel patch directly to the aortic graft (Fig. 135-9). The patch configuration is determined by the spacing of the vessels; the most common configuration is the celiac, superior mesenteric, and right renal arteries in one patch and the left renal artery attached separately. Another common grouping is the celiac and superior mesenteric arteries in one patch and both renal arteries attached separately. The Carrel patch is kept as narrow as possible to avoid patch aneurysms; but if the vessels are spaced too far apart, they are attached individually to avoid a large visceral patch that is prone to aneurysmal dilatation over time (Fig. 135-10). There is a tendency by inexperienced surgeons to sew all the visceral vessels on one patch or to create a too large proximal bevel, and this more often than not leads to problems later.81 In our experience, by using these principles for visceral patch reconstruction, we have avoided patch aneurysms in our patients. However, another strategy that has been advocated is individual bypass grafts to the visceral arteries,82 especially in patients with connective tissue disorders like Marfan syndrome, because patch aneurysms are more prevalent in patients with connective tissue disease. In about a third of patients, an endarterectomy of some or all of the renal and visceral vessels is required (Fig. 135-11) because of calcified plaque with or without stenosis. If the renal or visceral artery has been previously stented, the stent is usually removed with the endarterectomy plaque. Some surgeons prefer bypass or direct stenting rather than endarterectomy because of possible flap dissection beyond the endarterectomy endpoint, which can require additional exposure and artery repair to avoid occlusion. However, the superior long-term patency of a properly endarterectomized artery is why we prefer endarterectomy. A bypass to a renal or visceral vessel may be necessary, however, if the endarterectomy fails or the artery is aneurysmal or too distant from the graft surface for easy no-tension reattachment. In patients with dissections, the dissection flap may extend some distance into a visceral or renal artery, which can create a possible flap occlusion with reattachment. In most cases, the flap can be excised to create a common channel for reattachment, but stenting the true lumen directly has also been proposed as an effective technique as well.83
Figure 135-9 A, When visceral and renal arteries are attached to the graft, it is important to keep the Carrel patch as narrow (small) as possible so patch aneurysms do not form. Spacing between the vessels determines patch configuration, and if the vessels are too far apart, they are attached individually. B, Sewing a visceral patch comprising the celiac and superior mesenteric artery with their balloon catheters in place. These are removed after blood flow to that portion of the aorta is established. C, The completed graft within the aneurysm sac with arrows to the visceral patch and left renal artery. LRA, Left renal artery; RRA, right renal artery; SMA, superior mesenteric artery.
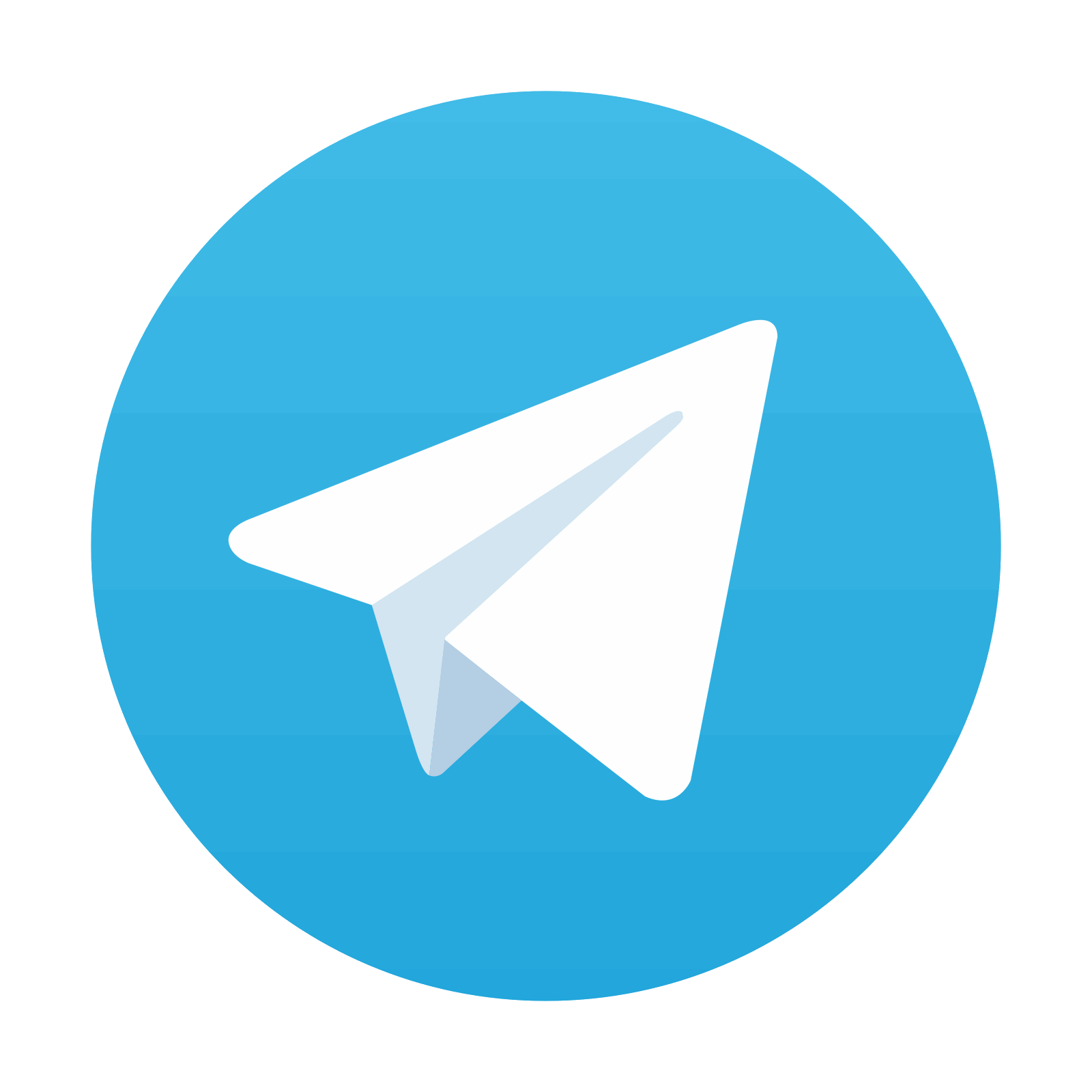
Stay updated, free articles. Join our Telegram channel

Full access? Get Clinical Tree
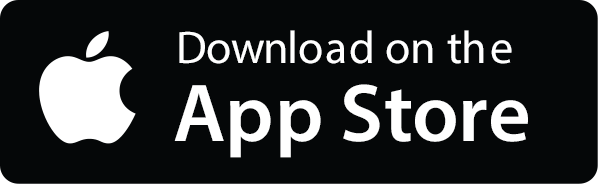
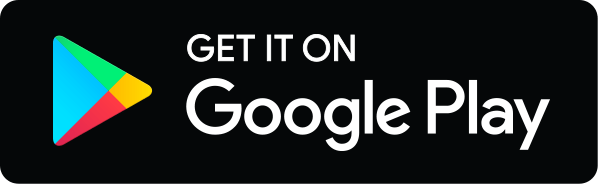