Chapter 136
Thoracic and Thoracoabdominal Aneurysms
Endovascular Treatment
Michael J. Singh, Michel S. Makaroun
Based on a chapter in the seventh edition by Jae Sung Cho and Michel S. Makaroun
Shortly after Parodi et al1 first reported endovascular aneurysm repair (EVAR) of the abdominal aorta, Dake et al2 introduced the same concept of stent-graft exclusion for the thoracic aorta in December 1994. These stent-grafts were custom designed for each patient and constructed of self-expanding stainless steel stents covered with woven Dacron grafts. Almost 20 years later, thoracic endovascular aortic repair (TEVAR) for descending thoracic aortic aneurysms (DTAAs) has become the standard of care. Mainstream technology has advanced at a rapid pace since the release of the first commercially available endograft in 2005 with the TAG device (W. L. Gore and Associates, Flagstaff, AZ). Experimental work continues through a combination of industry-sponsored research and physician-driven back-table modification. The penultimate goal is to preserve in-line branch vessel flow through an endovascular approach for repair of complex thoracoabdominal aortic aneurysms (TAAAs). Chuter and associates used branched grafts in 2001 for the first total endovascular repair of TAAA with preservation of all four visceral arteries.3–7
In contrast to EVAR, which has been compared with open surgical alternatives in randomized controlled trials, most clinical information about TEVAR originates from industry-sponsored, nonrandomized comparisons to open repair of DTAA as well as from single-center experiences or registry data that combine different pathologic processes and indications. Nonetheless, TEVAR has emerged as an attractive option for the treatment of DTAA and has shown very good early and midterm results.
Approved Devices for Thoracic Endovascular Aortic Repair
The pace of development of commercially available stent-grafts for the thoracic aorta has lagged behind that for infrarenal abdominal aortic aneurysms (AAAs) because of a lower incidence of DTAA, the need for a larger device profile, the challenging hemodynamic forces in the thoracic aorta, and the proximity to the great vessels. Despite these hurdles, steady albeit slow progress has been made, with several new thoracic devices released since 2005, and many others are still undergoing testing. Five commercially available devices have completed their regulatory trials and are currently approved for use in the United States, although many more are in use throughout the world.
Gore Conformable TAG Device
The Gore TAG endoprosthesis (W. L. Gore and Associates, Flagstaff, Ariz) was the first thoracic endograft approved by the U.S. Food and Drug Administration (FDA) in March 2005. The Conformable TAG endoprosthesis is a third-generation device that was FDA approved in January 2012. The device was specifically designed to conform to the aortic wall, to improve aortic wall apposition, and to minimize the risk of proximal endograft bird-beaking. It was developed with the concept of maximizing radial fit, which was an advancement from the original TAG device. The current device is made of an expanded polytetrafluoroethylene (ePTFE) tube reinforced with an ePTFE/fluorinated ethylene propylene film and an external nickel-titanium (nitinol) self-expanding stent affixed to the graft with ePTFE/fluorinated ethylene propylene bonding tape (Fig. 136-1). This device deploys from the middle of the graft toward each end, a design characteristic intended to prevent a windsock effect at the proximal fixation zone. A circumferential PTFE sealing cuff is located at the base of each flared, scalloped end. The device is constrained by ePTFE tape that is released during deployment from the middle of the device toward both ends. Devices are currently available in 21- to 45-mm diameters and 10-, 15-, or 20-cm lengths, depending on the diameter. The device also has various tapered graft sizes. The delivery profile requires 18F to 24F introducer sheaths. A minimum of a 2-cm neck is required for sealing on both ends, and broad oversizing (6% to 33%) of the device in relation to the native aorta is recommended. The current TAG device is a modification of the original device that was introduced when longitudinal wire fractures were discovered. It is constructed from three ePTFE layers to increase strength and to reduce porosity.
Medtronic Talent Captivia Device
The Talent device (Medtronic, Santa Rosa, Calif) is composed of a thin woven polyester fabric sewn to a self-expanding nitinol wire frame. The device was approved by the FDA in June 2008 and comes in four different configurations: proximal main, proximal extension, distal main, and distal extension. The proximal stent-grafts and the distal extensions are equipped with a bare spring (FreeFlo) to improve fixation and to allow deployment across the origins of the great vessels proximally and the celiac axis distally. It has a wide assortment of device diameters, ranging from 22 to 46 mm. The lengths are 110, 160, and 200 mm. Oversizing of 10% to 20% in relation to the aortic neck is recommended, and a 2-cm sealing zone is required in the proximal neck. Tapered grafts are also available to accommodate differences in diameter along the length of the aorta. The grafts are loaded in 22F to 25F delivery sheaths.
Medtronic Valiant Captivia Device
In April 2011, the Valiant device (Medtronic, Minneapolis, Minn) was approved by the FDA. It is a modified Talent endograft without the longitudinal connecting bar and with increased stent peaks and closed web options. It is a monofilament polyester graft attached to sinusoidal nitinol springs that are placed on the outside of the graft. The modular design consists of an eight-peaked proximal FreeFlo configuration that evenly distributes radial force across the proximal aortic neck (Fig. 136-2). Similar to the Talent device, it has a wide assortment of diameters, ranging from 22 to 46 mm. It is available in various lengths ranging from 107 to 224 mm and has both closed web and open FreeFlo stents available proximally and distally. Oversizing of the graft diameter (3-5 mm) in relation to the native aorta is recommended. It has completed clinical testing in the United States. Multiple publications have reported the Valiant results (TRAVIATA registry, VIRTUE registry, Valiant Captivia registry, and VALOR II pivotal trial), which demonstrate that the Valiant device is safe and effective in the treatment of DTAAs.
Cook TX2 Device with Pro-Form
The Cook TX2 (Cook Medical, Bloomington, Ind) is a one or two-piece modular graft made of woven Dacron fabric sewn to self-expanding stainless steel Z-stents. The Pro-Form modification uses a trigger-wire release mechanism that provides improved proximal conformity and wall apposition. During deployment, the proximal stent remains in a trifold configuration, which allows pinpoint placement and avoids a windsock effect. Through deployment, the trigger wires hold the entire proximal seal stent in place parallel to the aortic inner curvature. Unique to this device, the metal stents are on the inside of the graft in the sealing zone but on the outside in the rest of the endoprosthesis (Fig. 136-3). The device is available in straight and tapered designs. The graft diameters range from 28 to 42 mm, and lengths are 120 to 216 mm. Active fixation is provided by barbs on both ends that are oriented caudad in the proximal sealing stent and directed cranial on a bare distal stent; these barbs are specifically intended to prevent graft migration and component separation during conformational changes in the aneurysm after TEVAR. The device is delivered in a 20F or 22F hydrophilic introducer sheath, which facilitates insertion and allows a controlled deployment. A minimum of a 3-cm landing zone is recommended.
Bolton Relay plus Device
The Bolton Relay Thoracic Stent-Graft with Plus Delivery System (Bolton Medical, Sunrise, Fla) is a one-piece endograft made of woven polyester fabric sewn to self-expanding polished Duralloy stents. Longitudinal support is provided by a curved nitinol spiral support strut that aids aortic conformability. The device was FDA approved in September 2012. Distinct stent performance zones provide varying degrees of radial support and apposition. The unique transport delivery system consists of a series of coaxial sheaths and catheters that facilitate precise endograft deployment. The device is available in straight and tapered designs. The diameters range from 28 to 46 mm, with lengths of 100, 150, 200, and 250 mm (Fig. 136-4). Active proximal fixation is provided by bare stents on the Relay plus device. A non–bare stent configuration (NBS) is also available. The device is constrained in a 22F to 26F delivery sheath. Depending on the endograft diameter, a minimum landing zone of 15 to 25 mm is recommended.
Thoracic Endovascular Aortic Repair for Descending Thoracic Aneurysms
Indications and Contraindications
The indications to treat a thoracic aneurysm depend on various factors, including size of the aneurysm and its rate of growth, anatomic location, presence of symptoms, and general medical condition of the patient (see Chapter 134). Similar criteria should be applied for both open and endovascular repair and commonly include diameter of 6 cm or larger, saccular configuration, and symptomatic aneurysms, including rupture. The size recommendations are somewhat variable because no randomized trials exist to guide the decision-making process. The main considerations in the preferential choice of TEVAR over open repair are based on anatomy and comorbidities. There should be an appropriate landing zone (typically >20 mm) both proximally and distally to allow adequate sealing and exclusion of the aneurysm from the circulation as well as appropriately sized arterial access to deliver the stent-graft to its desired location. Anatomy, however, is not the only parameter in treatment planning; age and risk assessment also play a significant role in selecting the appropriate treatment modality.
In the presence of suitable anatomy, TEVAR seems to be the logical choice in most patients because of its lower morbidity and mortality. However, even in these patients, the decision should be tempered by the paucity of extended follow-up data for TEVAR and the requirement for lifelong clinical and imaging follow-up. Most endograft fatigue testing is carried out to 10 years on the basis of International Organization for Standardization recommendations. Therefore, consideration should be given to open repair in younger patients with a longer life expectancy and apparent low risk for open repair. For high-risk individuals, the decision is simple, given that clinical results clearly favor an endovascular approach. As more long-term data become available, more liberal use of TEVAR in lower risk individuals may become easier to justify, particularly in light of the demonstrated significant perioperative reduction in risk with TEVAR (see later).8 In the absence of level A or B evidence comparing the results of open repair of DTAA and TEVAR, lack of long-term durability data, and potential deleterious effects of repeated radiation exposure necessary for graft surveillance, some investigators advocate TEVAR only when the predicted operative risk is clearly lower than the risk associated with conventional open repair.9
The majority of patients, however, do not have “ideal” anatomy for TEVAR, and the risk/benefit assessment becomes more complex. Many aneurysms impinge on a major arterial branch that must be covered for adequate sealing. In these situations, the safety of such branch sacrifice must be balanced against further observation of the aneurysm or open repair. The alternative would be surgical bypass or “debranching” to maintain perfusion while extending the sealing zone, as reviewed later. The incremental risks of branch occlusion or the particular surgical bypass involved should be carefully evaluated in considering the TEVAR option, which would be appropriate only in the case of poor-risk individuals with large aneurysms. This category includes those with cardiac or pulmonary comorbid conditions that would preclude safe open repair and those with acute manifestations or “high-risk” anatomic conditions, such as previous thoracotomy.
Many relative contraindications to stent-graft treatment of DTAA exist. TEVAR should be contraindicated when the anatomy of the aneurysm prevents safe and effective performance of stent-graft exclusion of the DTAA because short- and long-term complication rates are increased in such cases. Aortic diameters outside the instructions for use treatment range of available devices should be avoided. Severe tortuosity of the aneurysm or of the access vessels can increase morbidity. Severe aortoiliac occlusive disease, rapid taper of the aorta, and circumferential thrombus at the attachment sites preclude the safe performance of TEVAR, so TEVAR should be cautiously used in the presence of these conditions. Severe angulation of the arch of greater than 60 degrees adjacent to the proximal portion of the aneurysm increases the difficulty of device tracking and sealing at that level. Endovascular repairs should be avoided in patients with life-threatening allergic reactions to intravenous contrast material or to nickel or stainless steel, in patients with advanced chronic renal insufficiency (because of precipitation of end-stage renal failure), and in those who cannot be monitored reliably.
Relevant Anatomy
Careful assessment of anatomy and meticulous preoperative planning are essential for successful TEVAR. Although anatomic requirements can be device specific, adequate arterial access and sealing zones are essential for all. Several anatomic features, when present, may facilitate TEVAR or render it very difficult.
Iliac Anatomy
Most procedures use the common femoral artery as access to the aorta. Vessel calcification, small lumen size, and degree of tortuosity can have a profound impact on the procedure. The right iliac artery usually has less of an acute angle at the aortic bifurcation and thus is favored for device access, whereas the contralateral side is usually reserved for diagnostic angiography catheters to guide deployment. This, however, is not universally true, and individual assessment is required.
An ideal access channel should have a lumen of at least 8 mm in diameter to allow larger devices to be introduced when needed. The smallest diameter is typically found in the proximal external iliac artery, an area that is also more prone to calcified plaque, which can restrict delivery of the device. This is a particular problem in women, whose iliofemoral arteries tend to be smaller.10 When the narrowest segment of the iliac artery falls below the expected minimum diameter required for the planned device insertion, access is generally shifted proximally to the common iliac artery by use of an iliac conduit. Rarely, the entire iliac system is hostile to delivery of the device on both sides, and consideration should then be given to access through the distal aorta if the risks associated with thoracotomy are too significant.
The Aortic Channel
The abdominal aorta rarely presents an anatomic hindrance to delivery of the device, but tortuosity of the thoracic aorta can be challenging in the presence of aneurysmal disease because it is frequently associated with elongation and exaggeration of the normal curvatures of the aorta in the chest. As illustrated in Figure 136-5, this is particularly relevant in the case of a large descending thoracic aorta when cephalocaudad elongation causes exaggeration of the posteroanterior course of the aorta as it enters the aortic hiatus. This tortuosity, when it is combined with increased angulation at the transition from the arch to the proximal descending aorta, may result in difficulty in tracking because of bending of the delivery sheath at flexion points as it negotiates severely angulated regions.
Aortic Sealing Zones
Adequate fixation of a stent-graft to both proximal and distal landing zones is essential for successful performance of TEVAR. To standardize comparisons, reporting standards for TEVAR were published by the Society for Vascular Surgery, and 11 landing zones have been defined for the management of thoracic aortic disease (Fig. 136-6).11 This standardization aids in determining the risk and complexity of the procedure in relation to the site of fixation and length of aortic coverage. Several publications have shown that the frequency of neurologic events correlates with location of the proximal landing zone.12,13 The original proposal had four attachment zones for the ascending aorta, arch, and proximal descending aorta. The updated and extended reporting system precisely defines the proximal and distal attachment zones, which in turn aids in the determination of device selection, operative risk, and complexity of the procedure. The proximal attachment zones are based on the location of the proximal edge of the TEVAR device in relation to branch vessels:
zone 0: proximal to the innominate artery;
zone 1: proximal to the left common carotid artery (CCA);
zone 2: proximal to the origin of the left subclavian artery (SCA);
zone 3: the proximal descending thoracic aorta (<2 cm from the left SCA);
zone 5: distal half of the thoracic aorta to the celiac artery;
zone 6: celiac artery to the top of the superior mesenteric artery (SMA); and
zone 7: SMA to the suprarenal aorta.
zone 8: involves the perirenal aorta;
zone 10: common iliac arteries; and
Zones 4 to 7 may also represent distal attachment zones for TEVAR.
Atherosclerotic aneurysms typically are not limited to one focal area, and the degenerative process usually involves the apparently normal adjacent aorta. This is important in selection of landing zone length. Although 2 to 3 cm is considered adequate for various devices, a longer seal zone may be preferable, especially at angulated areas to decrease the occurrence of late endoleaks and to protect from late aneurysmal degeneration. This urge to cover more aorta, however, should be tempered by the increased risk for spinal cord ischemia with excessive coverage. If it is anatomically possible, the landing zone should ideally be parallel in configuration and distal to the left SCA (zones 3 and 4) and proximal to the celiac artery (Fig. 136-7). This results in the best outcomes. A sealing zone in a curved portion of the aorta, generally near the left SCA (zones 2 and 3), presents added complexity. The longitudinal stiffness of the devices often limits complete apposition to the inner curve. The minimum sealing length in those regions should apply to the shorter inner curve rather than to the longer outer curve. The proximity of the sealing zones to major branches should be carefully evaluated to avoid occlusion of a necessary artery. Alternatively, a bypass could be planned to maintain patency of the branch vessel or a chimney/snorkel technique used to preserve in-line branch vessel flow. The decision whether to revascularize the left SCA is discussed under Management of the Left Subclavian Artery.
Figure 136-7 A, Operative thoracic aortography demonstrating a large thoracic aortic aneurysm. Note the parallel proximal and distal landing zones. B, Completion aortography showing the thoracic endograft deployed proximal to the left subclavian artery with complete exclusion of the aneurysm. Note the retrograde filling of the left subclavian artery without endoleak (arrow).
Operative Planning and Options
Imaging
Preoperative planning begins with imaging studies to delineate the morphologic features of the aneurysm, its relationship to adjacent arch and mesenteric vessels, and the quality of access vessels. The primary modality for such planning is computed tomographic angiography (CTA), which has mostly supplanted the use of catheter angiography in preoperative planning and is capable of providing all preoperative anatomic information. Although fine reconstructions (<2.5 mm) from older-generation scanners are acceptable, the 64-slice computed tomography (CT) scanner is the latest technology and provides high definition and very fast scan times. It also offers three-dimensional volume rendering as well as maximum intensity projections and sagittal and coronal reconstructions, which aid in the analysis of angles and relationships to adjacent vessels. Software such as M2S (M2S, West Lebanon, NH), Vitrea (Vital Images, Minnetonka, Minn), and Aquarius (TeraRecon, San Mateo, Calif) offers additional tools, such as reconstructions orthogonal to the line of flow and virtual grafts to aid in measurements and procedure planning. When patients are unable to undergo CTA because of life-threatening allergies to contrast material or renal insufficiency, gated magnetic resonance angiography (MRA) can be substituted for additional information and is usually added to a CT scan without contrast enhancement.
The preoperative CTA should include the aortic arch, chest, abdomen, and pelvis with and without contrast enhancement to fully evaluate the native vessels, focusing on the extent of calcification and angulation throughout. Tortuosity is best delineated by a three-dimensional reconstruction. Qualitative evaluation of arch disease for atheromatous burden as well as the location of the vertebral arteries is critical to minimize the risk of cerebral ischemic complications when proximal extension into zones 1 and 2 is planned. This can be achieved by extracranial imaging with CTA or MRA. Determination of pelvic perfusion is essential in assessing perfusion to the spinal cord, especially in patients who have undergone a prior aortic repair, or in anticipating coverage of a long segment of aorta or the left SCA without revascularization.
Sizing
Accurate measurements of the diameter of the access vessels and the sealing zones are important to determine the feasibility of the procedure and appropriate graft sizes, which are typically oversized by 6% to 36%, depending on manufacturers’ recommendations. These recommendations should be followed closely because they form the range in which fatigue testing of the stent-grafts was performed in the preclinical assessment phase. Too small a stent-graft can result in an endoleak or migration, whereas excessive oversizing may cause infolding, poor sealing because of gutter formation and excessive radial force with accelerated degeneration of the neck, and possibly earlier material failure. Diameters are best measured on reconstructions orthogonal to the line of flow, especially in areas of tortuosity and angulation, to avoid erroneous measurements. If reconstructions are not available, the short axis is a reasonable estimate of the true diameter.14 Neck diameters between the proximal and distal sealing zones can be different, which may lead to inappropriate oversizing on the smaller end. Strategies for overcoming this problem include tapered grafts and shorter devices deploying a larger device inside the smaller one to prevent a type III endoleak.
Appropriate length measurements are also necessary to estimate the required device lengths. This is difficult in the thoracic aorta because the aorta moves posterolaterally in its proximal portion and anteromedially near the diaphragm. The presence of a DTAA exacerbates this native tortuosity and adds complexity in that the aorta and aneurysm have both inside and outside measurements that can be different from each other. The best estimate of length is a centerline flow measurement from a three-dimensional reconstruction, although this will commonly underestimate the length of endografts needed because the graft will tend to gravitate toward the greater curvature, especially when a large cavity exists in the aneurysm sac.
Selection of Access
Planning for access clearly depends on device size and access arteries. With TEVAR requiring larger device sizes and with DTAA being more prevalent than infrarenal AAA in women,15 an iliac conduit is often needed, in up to 15% of patients, and is better done prophylactically than after an iliac artery injury.16 Creation of an internal endoconduit has been described to address unfavorable iliac artery anatomy during TEVAR.17 This technique uses the controlled deployment of a stent-graft across a stenotic iliac artery, followed by balloon angioplasty and controlled rupture of the diseased segment. In turn, this facilitates safe passage of the TEVAR device. Technical advancements of introducer sheaths have also aided in the management of challenging iliac access. The Terumo SoloPath Balloon Expandable Transfemoral Access Sheath (Terumo Medical Corporation, Somerset, NJ) is a larger size hydrophilic introducer sheath (14F-21F) with an external noncompliant radially expanding balloon.18 This device eliminates the need for tapered dilators and facilitates delivery though difficult anatomy, and the balloon passively collapses before the removal of the introducer sheath, which may reduce the risk of iliac artery avulsion.
Identification of Landing Zones
The lack of adequate landing zones both proximally and distally is encountered frequently. Extension into zone 2 to achieve adequate sealing proximally was required at least 20% of the time in some regulatory trials.16 The decision to cover the left SCA or to bypass it has undergone a substantial paradigm shift over the years. Although all early cases underwent a left carotid–subclavian bypass or transposition, later reports of safe coverage resulted in indiscriminate coverage of the left SCA.17–21 Recently, however, renewed interest in preserving flow in the left SCA developed after reports of brainstem stroke, increased risk for spinal cord ischemia, and severe arm ischemia.22,23 It has been shown that a dominant left vertebral artery is found in more than 60% of patients, which coincides with an absent or atretic right vertebral artery. Intuitively, this finding places the patient at a higher risk for a posterior circulation infarct. Intentional covering of the left SCA without revascularization has been shown in two studies to significantly increase the overall risk of stroke and specifically the risk of posterior circulation stroke (5.5% vs. 1.2%).24,25
Management of the Left Subclavian Artery
The left SCA is an important vessel for perfusion of both the spinal cord and the brain through the left vertebral artery.26 It also provides blood flow to the spinal cord through the internal mammary and its anterior intercostal branches.27 When it is feasible, it should be preserved during TEVAR when a stent-graft is deployed at or near the left SCA. Placement of a transbrachial left SCA access wire in these situations can be helpful. It can serve as a guide for safe and precise deployment of the device at the level of the left SCA and as a rescue procedure to restore flow should inadvertent coverage of the vessel occur.28 It can also be used for proximal SCA embolization at completion of the procedure to prevent a type II endoleak after intentional coverage of the vessel.
Coverage without Revascularization.
Although coverage of the left SCA is generally well tolerated because of a rich collateral network,17–21,29 routine exclusion should be discouraged because both experimental and clinical evidence suggests that not all patients can tolerate the occlusion safely.29–31 When reconstruction is undesirable, careful preoperative imaging should be performed to assess the patency of the right vertebral artery into the basilar artery, as well as the circle of Willis, to identify patients who cannot tolerate left SCA occlusion. Others can safely undergo coverage as an initial intervention with later revascularization if necessary. Mandatory left SCA revascularization is indicated in the presence of a dominant left vertebral artery, patent left internal mammary bypass to a coronary artery, left arm arteriovenous access for hemodialysis, aberrant right SCA, hypoplastic or absent right vertebral artery, termination of the left vertebral artery into the posterior inferior cerebellar artery, occluded internal iliac arteries, or anomalous origin of the left vertebral artery from the arch.22,29,32,33 Revascularization should also be considered when an elevated risk for paraplegia exists with extensive coverage of the thoracic aorta combined with previous infrarenal aortic replacement.
Coverage without revascularization has been linked to an increased risk for spinal cord ischemia in a review of EUROSTAR (European Collaborators on Stent/Graft Techniques for Aortic Aneurysm Repair) registry data.23 The incidence of left SCA occlusion without revascularization was significantly higher in those with spinal cord ischemia (40%) than in those without (19%). On multivariate analysis, left SCA coverage without revascularization was an independent predictor of spinal cord ischemia with an odds ratio of 3.49. A Northwestern series also indicates the importance of selective left SCA revascularization when its coverage is necessary.22 In a series of 30 patients with left SCA coverage, 22 with and 8 without periprocedural left SCA revascularization, a 63% incidence of acute complications (stroke and subclavian steal syndrome) was noted in patients without left SCA reconstruction, whereas only one delayed paraparesis and two vocal cord palsies occurred in those with left SCA transposition/bypass grafting. Delayed revascularization can usually be performed in cases of arm ischemia without untoward effects.22,33 Revascularization of the left SCA may be performed by either left CCA–to–left SCA bypass grafting or left SCA–to–left CCA transposition. Although transposition eliminates any source of type II endoleak, it is more difficult and less desirable in these situations because the left SCA can be aneurysmal or distorted by the proximity of the descending thoracic aorta; furthermore, preservation of the posteriorly located vertebral artery can be difficult, and for this reason most surgeons favor carotid-to-subclavian bypass grafting (see Chapter 120) with ligation of the left SCA proximal to the left vertebral artery. This eliminates any potential for retrograde type II endoleak.
Revascularization.
Current Society for Vascular Surgery practice guidelines recommend that in patients who require an elective TEVAR in which the left SCA is expected to be covered, preoperative revascularization should be performed in situations in which collateral perfusion is compromised.32 Frequently, however, deployment of a stent-graft into zone 2 is necessary to achieve a proximal seal in the absence of an acceptable landing zone distal to the left SCA. Prophylactic left SCA revascularization was performed routinely during the early regulatory trials to avoid potential neurologic or upper extremity ischemic complications (Fig. 136-8).32 The procedure, however, adds an operative intervention with its attendant risks. In addition to death and stroke, recurrent laryngeal and phrenic nerve injuries as well as thoracic duct injuries have been reported.22,34 McWilliams was the first to describe an in situ stent graft fenestration of a modified Cook TX1 thoracic endograft in 2004. The original transluminal technique was performed with use of the back end of a stiff 0.018-inch guide wire inserted through a left supraclavicular incision. After the TEVAR fenestration was created, it was serially enlarged with cutting balloons.35 Ahanchi recently reported his midterm results in a series of six patients who underwent in situ laser fenestration for revascularization of the left SCA during emergent TEVAR. This technique was performed through left brachial artery access and retrograde insertion of a 2.0- to 2.5-mm Turbo Elite laser catheter (Spectranetics, Colorado Springs, Colo). The laser was juxtaposed to the TEVAR device, and 3 to 5 seconds of laser energy was applied. The fenestration was cannulated with a 0.018-inch guide wire, which was then upsized to a 0.035-inch wire for better stability. Serial dilation with 6-, 8-, and 10-mm angioplasty balloons was followed by placement of a covered balloon-expandable stent that was flared proximally. Five of six cases were technically successful; a sharp angulation of the proximal left SCA prevented use of the laser. Mean follow-up at 8 months (range, 1-17 months) confirmed that all five in situ fenestrations remained patent.36
Debranching of the Arch Vessels
Several alternative treatment options have emerged to maintain branch vessel perfusion during TEVAR in zones 0 to 3; these include hybrid procedures with extra-anatomic bypasses, endovascular debranching with a chimney technique, and open aortic debranching. The goals with each of these techniques are to extend the proximal landing zone, to lower the risk of a type Ia endoleak, and to decrease operative morbidity and mortality. Despite the theoretical benefits of a hybrid repair, outcomes after arch debranching are poorly defined. The management of thoracic aneurysms juxtaposed to the left SCA has been previously discussed.
Thoracic aneurysms encroaching on the left CCA are not uncommon and can be technically challenging to manage. If coverage of the left carotid artery is needed, several options are available to maintain carotid perfusion. The most commonly performed procedure is an extra-anatomic carotid-carotid bypass. Right common carotid exposure is obtained through an oblique right-sided neck incision. A similar exposure is obtained on the contralateral side. A ringed 6-mm PTFE graft is tunneled retroesophageally to connect the two carotid arteries. If it is too large, the retroesophageal graft may compress the esophagus and cause dysphagia. These bypasses have been found to be a safe and durable (3-year primary patency rate of 88%) procedure.37 In select situations when inflow from the right side (CCA or SCA) is not adequate and the patient is found to be at too high a risk for open arch reconstruction, sequential extra-anatomic bypasses are an option. This would involve performing a series of bypasses from the left common femoral to the left axillary artery and from the left SCA to the left CCA with prosthetic conduits. The length of the multiple bypasses does create concern in trying to define patency rates.38 Endovascular debranching techniques for the left CCA have been described.39 Exposure of the left CCA allows retrograde access into the aortic arch and the creation of a left carotid–SCA bypass if needed. TEVAR deployment from the femoral access and retrograde covered stent placement into the arch are then performed; this has been described as the chimney technique.40 The covered stent is then deployed at the same level as the TEVAR stent graft. Early results have shown this technique to be an acceptable option for high-risk patients.
Thoracic aneurysms encroaching on the innominate artery are the most challenging to manage. Hybrid endovascular repair options are well described. Involvement of zones 0 and 1 are often best managed with an open arch debranching originating from the ascending aorta (Fig. 136-9A). Through a median sternotomy, a bifurcated prosthetic graft is sewn to the ascending aorta, the innominate artery, and the left CCA. After the debranching, a TEVAR stent graft can be advanced to the origin of the conduit and deployed.41 Hybrid options for Zone 0 and 1 aortic arch debranching are shown in Fig. 136-9 B-D. Andersen proposed an algorithmic approach to the management of aortic arch disease. This technique was found to be successful but did carry a higher than expected rate of type A dissection and 30-day mortality (14.9%).42
Figure 136-9 A, Zone 0 open debranching of the supra aortic arch vessels with prosthetic graft, followed by a left common carotid to left subclavian artery bypass, delivery of aortic stent graft into ascending aorta. B, Zone 1 right common carotid artery to left subclavian artery retropharyngeal bypass with reimplantation of left common carotid artery, followed by delivery of aortic stent graft into the aortic arch. C, Zone 0 endovascular debranching of the supra aortic arch vessels, right common carotid artery to left subclavian artery retropharyngeal bypass with reimplantation of left common carotid artery, snorkel technique with simultaneous delivery of innominate artery covered stent and aortic stent graft into ascending aorta. D, Zone 1 endovascular debranching of the supra aortic arch vessels, left common carotid artery to left subclavian artery bypass, snorkel technique with simultaneous delivery of left common carotid artery covered stent and aortic stent graft into aortic arch.
Management of the Celiac Artery
If the distal landing zone is inadequate, the operator faces options similar to those with the left SCA. When adequate collateral circulation between the celiac axis and SMA, especially through the gastroduodenal artery, is ascertained by preoperative angiography, the celiac axis may be covered safely to extend the distal landing zone during TEVAR.43 Criado et al10 observed no mesenteric ischemic complications with three cases of celiac axis coverage. Vaddineni et al44 reported seven such cases without any mesenteric or spinal ischemic complications. If the collateral network is not adequate, a bypass to the celiac circulation must be provided before occlusion. At the 2008 annual meeting of the Society for Vascular Surgery, Darling et al45 presented their series of 21 patients with celiac axis coverage during TEVAR. They observed one fatal hepatic ischemic complication in a patient who had no demonstrable SMA-celiac collaterals, but no late complications were noted. They concluded that celiac coverage can be performed safely in the presence of SMA-celiac collaterals. The periscope graft is an acceptable option in situations in which celiac coverage is necessary and open revascularization is not (Fig. 136-10). This technique has proved useful as a means of preserving or rescuing aortic branch vessels in stent-graft seal zones. A self-expanding covered stent is inserted into the celiac artery from a femoral access. The TEVAR stent graft is deployed in the standard manner. Reinforcement of the self-expanding stent may be necessary because of the steep downward angle created by the covered stent and TEVAR.40
Adjunctive Measures for Neuroprotection
As reviewed later, TEVAR is not without risk for spinal ischemic complications, and there is consensus that patients with long-segment descending thoracic aortic coverage and antecedent or concomitant abdominal aortic grafting are at particular risk. In such circumstances, adjuncts are often used, albeit without a firm evidence base. Such techniques have been evaluated mostly during open repair of TAAA. For a more detailed discussion, the reader is referred to Chapter 135.
One of the most commonly used adjuncts with TEVAR is drainage of cerebrospinal fluid (CSF). Although some use it routinely, most centers use it selectively in patients with an elevated risk for paraplegia, such as extensive coverage of the thoracic aorta in association with prior aortoiliac intervention. The spinal drain is typically inserted preoperatively and maintained for 48 to 72 hours while CSF pressure is kept below 10 cm H2O. The advantage of selective drain use with TEVAR relates to an obligatory intensive care unit admission, but it is also desirable for arterial line monitoring and maintenance of relative hypertension. The incidence of hemorrhagic complications from catheter placement is also not negligible, and such complications are reported in 0% to 3% of patients.46 Regardless of whether it is used preoperatively, CSF drainage has been shown to be effective in reversal of delayed-onset paraplegia after TEVAR and should be used whenever spinal cord ischemia is suspected.47
Besides the anatomic features noted earlier, hemodynamic stability seems to play a critical role in spinal cord protection because the underlying pathologic process appears to be poor collateral flow and decreased perfusion of the spinal cord. A low intraoperative mean arterial blood pressure (≤70 mm Hg) has been linked to spinal cord ischemia in one review.47 Except for the short time required for endograft deployment, when relatively low blood pressure may be beneficial, an elevated pressure is preferred intraoperatively. Similar to the open DTAA repair paradigm, postoperative hypotension has been noted to precipitate spinal cord ischemia events in patients after TEVAR.
Of pharmacologic adjuncts, naloxone has been studied the most. Intravenous naloxone has been shown to be effective in decreasing circulating levels of excitotoxic amino acids and antagonizing opiate-mediated spinal cord vasoconstriction after open TAAA repair. Its beneficial effects have been observed in open procedures,48,49 and it may be used in selected cases of TEVAR.
The importance of the artery of Adamkiewicz, which is usually located between T8 and L2, has been debated extensively but has not clearly been linked to spinal cord ischemia. The EUROSTAR review found an association between T10 coverage and spinal cord ischemia; 40% of patients with spinal cord ischemia exhibited T10 occlusion, and only 18% of this 40% did not suffer periprocedural paraplegia or paraparesis.23 The association, however, was no longer present on multivariate regression analysis. Accordingly, no recommendation can yet be made to avoid covering the T10 region with a stent-graft; the variable origin of this vessel along the length of the thoracic aorta and its multiple contributing collateral vessels form the essence of this position.
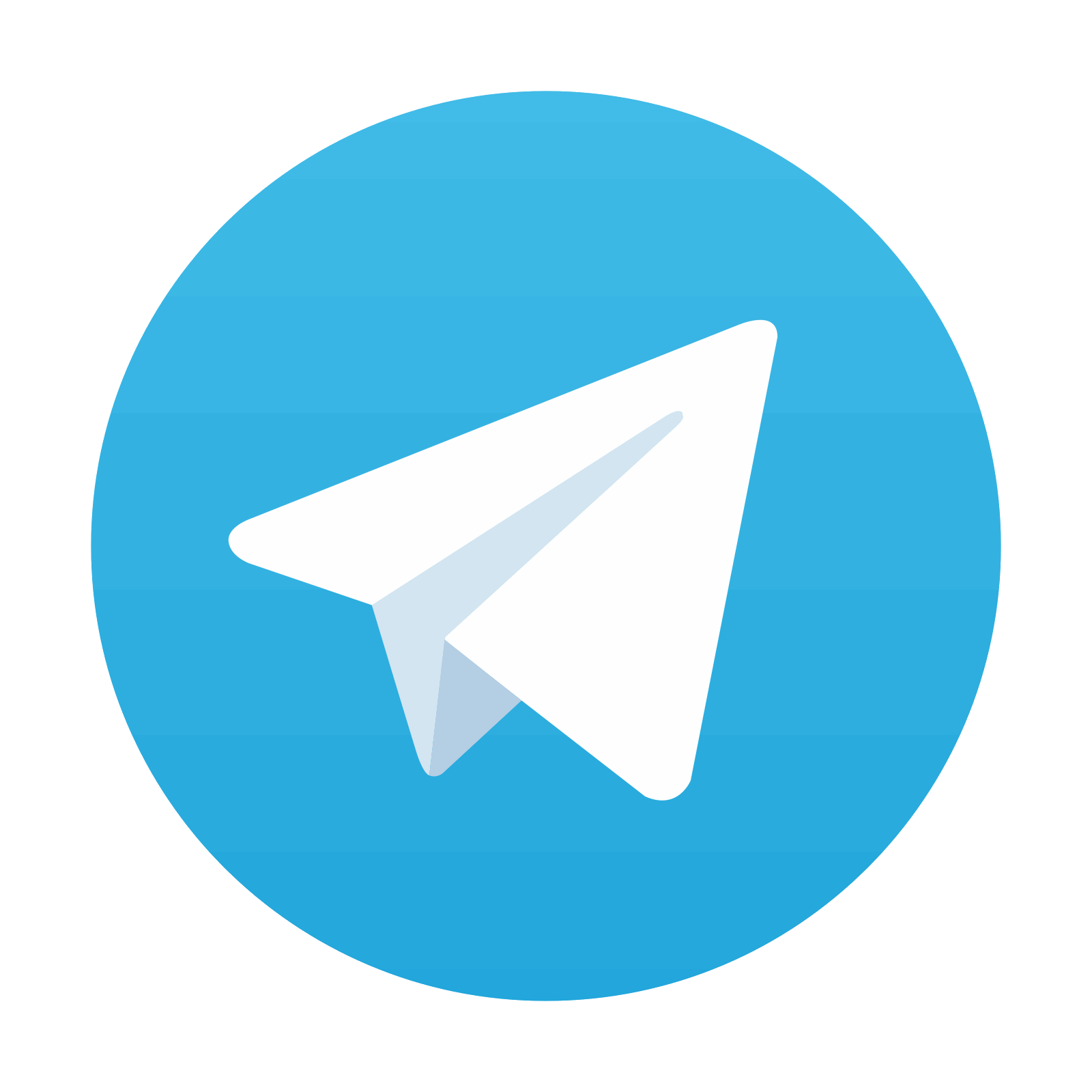
Stay updated, free articles. Join our Telegram channel

Full access? Get Clinical Tree
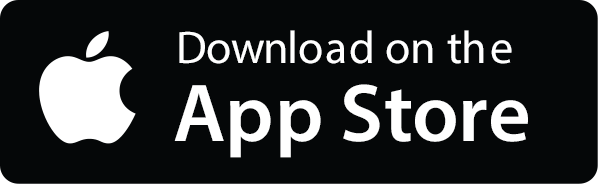
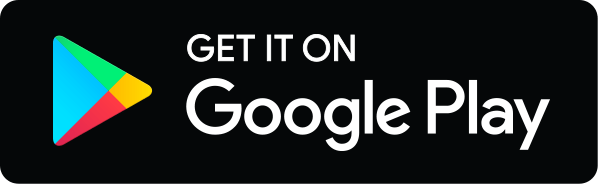