Chapter 60 The Wound Care Center and Limb Salvage
Normal Wound Healing
Wound healing is a complex process, the details of which we are only beginning to understand. Ideally, wound healing involves a well-organized, multifaceted series of events beginning after the actual event of wounding and ends with the formation of mature scar tissue (Figure 60-1). The acute wound is caused by external trauma, and acute wounds heal within a predictable time frame by progressing through orderly phases. Chronic wounds, however, do not follow this predictable course of events and may be the result of or impeded by internal events. However, correction of the internal problem does not always guarantee that the wound will heal in a timely fashion. Health care providers are just now beginning to comprehend the complex cellular and biological abnormalities that are inherent in the wound that has failed to heal.
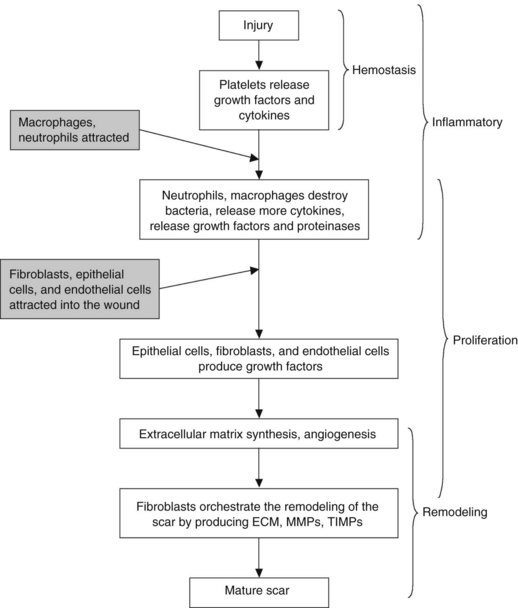
FIGURE 60-1 The normal process of wound healing is complex and involves a series of phases that overlap.
Much of the latest research on wound healing involves investigation of the effects of various cytokines, growth factors, proteinases and their regulators and how they control the process of wound healing (Figure 60-2). To understand the effect of these substances on the chronic wound, the normal healing process of the acute wound must be understood. In acute wounds, the healing process begins with tissue injury and progresses predictably through the four phases of wound healing: hemostasis, inflammation, proliferation, and remodeling. These stages of healing, while occurring in a predictable manner, can overlap and can last for months.
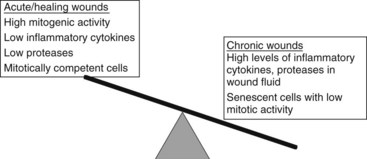
FIGURE 60-2 Balance of healing and nonhealing factors in wounds.
(Adapted from Schulz GS, Sibbald G, et al: Wound bed preparation: a systemic approach to wound management, Wound Rep Reg 11:1-28, 2003.)
Acute Wounds
Hemostasis
At the time of the initial injury, platelet activation and vasoconstriction occur after injury to the endothelium. Platelet aggregation, vasoconstriction, and clot formation begin the process of hemostasis. A number of soluble mediators are released by the platelets, including platelet-derived growth factor (PDGF), insulin-derived growth factor 1 (IGF-1), epidermal growth factor (EGF), fibroblast growth factor (FGF), and transforming growth factor-β (TGF-β). These mediators are responsible for initiating the healing process. Growth factors stimulate proliferation of wound cells, act as chemotactant agents, and regulate the differentiated functions of wound cells.1 Neutrophils and macrophages are recruited to the injured site, attracted by the chemotactant release by the platelets.
Chronic Wounds
In contrast to the acute wound, the chronic wound does not heal in a predictable fashion. Lazarus and colleagues2 defines the chronic wound as one in which the normal process of healing has been disrupted at one or more points in the phases of hemostasis, inflammation, proliferation, and remodeling. Chronic ulcers are characterized by defective remodeling of the ECM, a failure to epithelialize, and prolonged inflammation.3–5 In the chronic wound, fibroblasts do not readily respond to growth factors such as PDGF-β and TGF-β; this failure to respond is hypothesized to be due to a form of cellular senescence. Hyperproliferation at the wound margin interferes with normal cellular migration across the wound bed, probably because of inhibition of apoptosis within the fibroblasts and keratinocyte cells.6,7
The phases of wound healing are replete with factors that have played important roles in the progression of wound healing; therefore any alteration in one or more of these components may interfere with the healing progression. Growth factors such as PDGF, EGF, bFGF, and TGF-β are present in the chronic wound, but the levels remain constant, rather than displaying the variation seen in normal acute wound healing. The proinflammatory cytokines such as IL-1, IL-6, and TNF-α remain elevated in chronic wounds, when compared with the normal, acute wound, where a dramatic decrease is seen during healing, because there is a reduction in the inflammatory state.8
Several studies have shown that chronic wounds stall in the inflammatory phase of wound healing process.9–11 The inflammatory phase in wound healing is most often associated with an increased amount of exudate, postulated to be from infection, heavy colonization, or from reaction to increased necrotic tissue in the wound bed. The amount and character of fluid produced by the chronic wound can severely impede and even reverse the healing process.12–15
Studies investigating chronic wounds effluent have provided increasing evidence that the cellular and molecular environments are substantially altered in chronic wounds. Bucalo and colleagues16 collected exudate from venous ulcers and examined the effects of chronic wound fluid on the proliferation of dermal fibroblast, microvascular endothelial cells, and keratinocytes in culture. This study found that not only is wound fluid cytotoxic, it inhibited or failed to stimulate the proliferation of dermal fibroblasts, endothelial cells, and keratinocytes. Fluid from acute wounds, in contrast, has been found to stimulate fibroblast proliferation.17 Proteases in chronic wound fluid have also been shown to degrade growth factors such as PDGF and TGF-1.18,19
MMPs are a necessary component of the wound healing process and have an important role in cell migration and modification of the ECM. If the regulation of these protease molecules is disrupted, excessive MMP production may lead to degradation of the ECM, preventing cellular migration and attachment, and ultimately causing tissue destruction.20 It has been shown that levels of MMP activity are significantly elevated in a high percentage of chronic wounds when compared to acute wounds, suggesting dysregulation in chronic wounds (Figure 60-3). The activity of these proteases decreases consistently in patients whose ulcers progress from a nonhealing to healing phase.21
The chronic wound can also be heavily colonized or infected by bacteria that can substantially increase the amount of exudate. Thus, a copious amount of exudate produced by the chronic wounds, whatever its cause, can be a barrier to healing.15,16,22,23
Assessment of Wound Healing Capability
With the recognition that there are altered cellular and molecular processes at play in the chronic wound has come the realization that wound management must focus on both the wound and the patient as a whole. The goal of wound management is to attain a healthy, well-vascularized, granulating wound bed. For this to happen, factors that can impede healing must be addressed. The way in which chronic wounds are viewed and managed should be based on a model that is both different from the acute wound paradigm and representative of the complex nature of nonhealing wounds.24
Patient Assessment
The nutritional status of the patient is an overlooked factor in wound healing. Protein calorie malnutrition can have devastating effects on the integrity of the body and on any wounds the patient might have. Protein calorie malnutrition is defined as insufficient intake of both protein and calories. In protein calorie malnutrition, morbidity and mortality increases as a result of the proportionate decline in body weight.25 A decrease in lean body mass of greater than 10% is associated with compromised wound healing, despite therapeutic interventions. Once depletion of lean body mass reaches 30%, the wound becomes a secondary issue as the body seeks to restore and replenish lost muscle.26 Correction of any nutritional deficit should begin at the first visit and be maintained throughout the wound healing process. Assessment of dietary intake, including vitamin supplementation, is a necessary part of any wound healing assessment. Appetite stimulants, such as megestrol (Megace), may be helpful. If assessment shows moderate to severe protein calorie malnutrition, supplementation with oxandrolone has been shown to be beneficial to facilitate restoration of lean muscle mass.27
Assessment of Wound Characteristics
Assessment of the wound includes the location, size, depth, and color of the wound bed (Table 60-1). A cotton swab has been shown to accurately gauge the depth and involvement of deep structures. The depth of a wound can be used to predict the likelihood of healing without extensive debridement of tendon or bone. The most common classification system of diabetic lower extremity wounds is the Wagner system, which grades the depth from superficial skin involvement to deep, involving tendon and bone.
TABLE 60-1 Documentation of Wound Characteristics
Category | Observation to be Documented |
---|---|
Wound size | Length, width, depth, area, volume |
Undermining presence, location, measurement | |
Appearance | Granulation tissue; slough, necrotic, eschar; friability |
Exudate | Amount, color, type (serous, serosanguineous, sanguinous, purulent), odor |
Wound edge | Presence of maceration, advancing epithelium, erythema, even, rolled, ragged |
Diagnostic Studies for the Nonhealing Wound
There are three questions that need to be answered with diagnostic studies. First, what is the depth of the wound? Depth determines treatment, because involvement of deep structures such as tendon or bone reduces the likelihood of healing without removal of the deep tissue and increases the risk of the ascending infection along tendon sheaths. Subfascial infection is particularly worrisome in diabetic patients who have reduced sensation of the foot and reduced ability to fight infection. The simplest test of depth is to probe the wound to determine whether tendon or bone is involved. This inexpensive and simple method has been shown to be as reliable as more expensive tests in determining the presence of osteomyelitis. Other tests such as plain radiograph and bone scan have less sensitivity and specificity than a magnetic resonance imaging (MRI) scan (Figure 60-4). All these tests have false negatives and positives, so that surgical exploration is often necessary to determine the presence of deep infection and osteomyelitis, as well as to debride and culture the wound.
Assessment of the large vessel vascular supply is a critical component of the examination. A palpable pulse indicates a blood pressure of greater than 80 mm Hg in the foot and 70 mm Hg in the hand.28 If a pulse is not easily palpable, use of a vascular laboratory examination is essential. Doppler waveforms and ankle-brachial index (ABI) as well as plethysmography (Figure 60-5) can be used with transcutaneous pressure of oxygen (TcPO2) and skin perfusion pressure to determine the adequacy of blood supply. In patients with falsely elevated “stiff” arteries (high ABI with poor Doppler signals), pulse volume recordings, Doppler waveforms, and toe pressures are more reliable.
Treatment of Nonhealing Wounds
Elimination of Edema
Edema reduces microvascular blood flow and the clearance of bacteria and protein from the wound. It eliminates or reduces the likelihood of healing, and other management is often futile until edema is eliminated. The most challenging wounds are those with edema and ischemia, because patients with limb ischemia often place the limb in a dependent position to relieve ischemic rest pain. Prolonged dependency increases the limb edema and reduces perfusion, which paradoxically lead to an additional need for dependency. In ischemic limbs, the best method to reduce edema is to elevate the limb above the heart. Compression using sequential devices (e.g., Lymphopress, wraps [Unna boot or Profore], or support hose at 20 to 40 mm Hg pressure) should be limited to patients with a documented normal ankle brachial index of greater than 0.80 (Figure 60-6).
Debridement
The presence of necrotic tissue in a wound is the most obvious marker of a chronic wound. Reestablishing the balance of cytokines, proteases, and growth factors should be the focus of wound treatment. Efficient removal of devitalized tissue (necrotic burden) is an essential step in chronic wound management. The underlying pathogenic abnormalities in chronic wounds cause a continual buildup of necrotic tissue, and regular debridement is necessary to reduce the necrotic burden and achieve healthy granulation tissue. Debridement of devitalized tissue reduces tissue damage and destruction, removes bioburden, and exposes dead space that can harbor bacteria. Debridement can be accomplished in several different ways (Table 60-2), and the method chosen should be dictated by the condition of the wound and the skill of the practitioner, as well as the patient situation.
Enzymatic Debridement
Alvarez and colleagues showed that the papain urea product achieved a better wound response than did collagenase, but there was not a significant difference in wound closure between the two groups.28a Some patients complain of burning when the papain urea is applied, and this may limit its use in some patients.
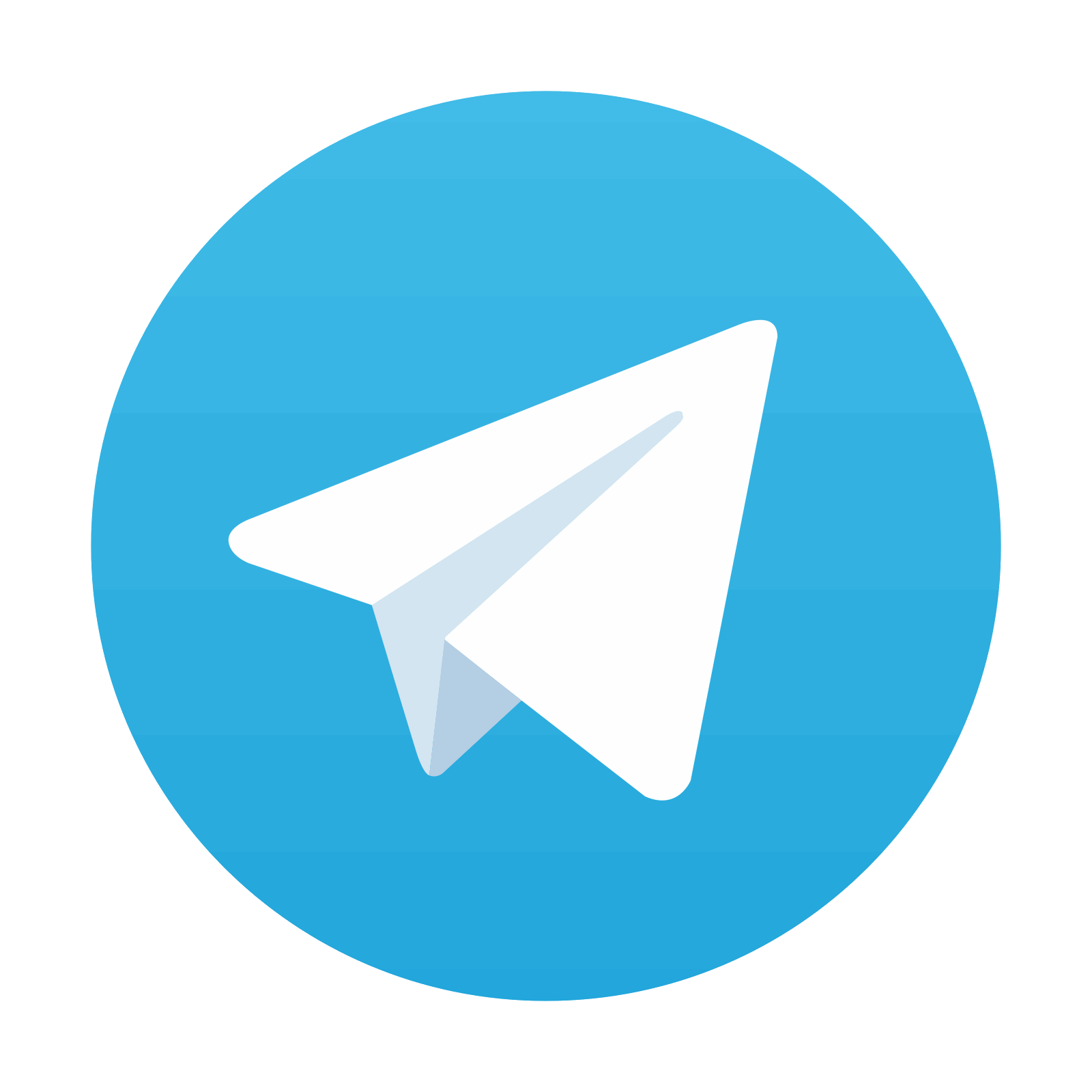
Stay updated, free articles. Join our Telegram channel

Full access? Get Clinical Tree
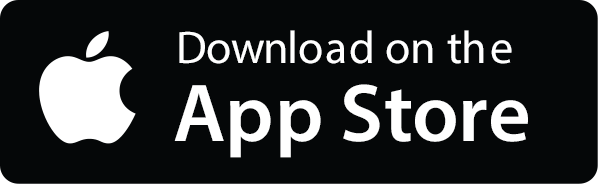
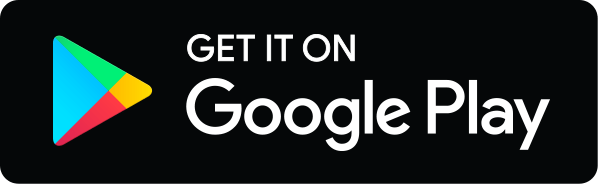