Fig. 41.1
Images from perfusion-fixed hearts from the Visible Heart® laboratory’s library : (1) calcified aortic valve (upper left panel), (2) pulmonary valve (upper right panel), (3) subvalvular apparatus of the mitral valve (lower left panel), and (4) tricuspid valve from the right ventricle (lower right panel). Modified from The Atlas of Human Cardiac Anatomy [12]
To date, our library of more than 350 hearts of various disease states continues to provide researchers with the ability to investigate how the cardiac anatomy may change/remodel under specific pathologies. In addition to anatomical investigations, these specimens can be employed to provide information as to how a specific device will fit the cardiac anatomies of the intended patient population, or how a delivery system will navigate through the chambers and vasculature of the heart. Such resources have allowed for the placement of multiple prototype devices and rapid comparison of how specific devices interact with the surrounding cardiac anatomies in a variety of human specimens [13, 14]. This information is critical in the development process, as it allows for engineers to highlight challenges regarding implantation of the device or the navigation of the delivery system that may have been overlooked in bench top testing.
Fresh cadaver hearts received by the Visible Heart® laboratory are documented at each stage of the acquisition process to record global anatomical changes during the fixation process, such as tissue weight and overall dimensions. Images of the fresh preparation, the resulting fixed specimen, and the nondestructive imaging of a sample specimen from the library (adapted from the Atlas of Human Cardiac Anatomy [12]) can be seen in Fig. 41.2.
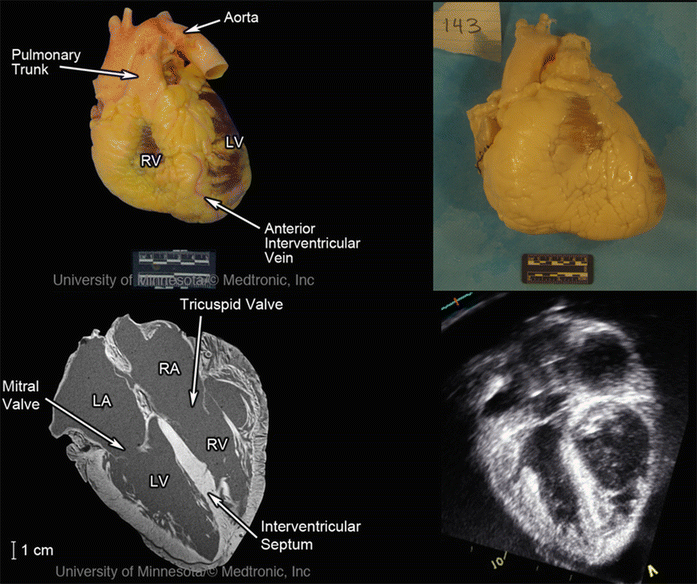
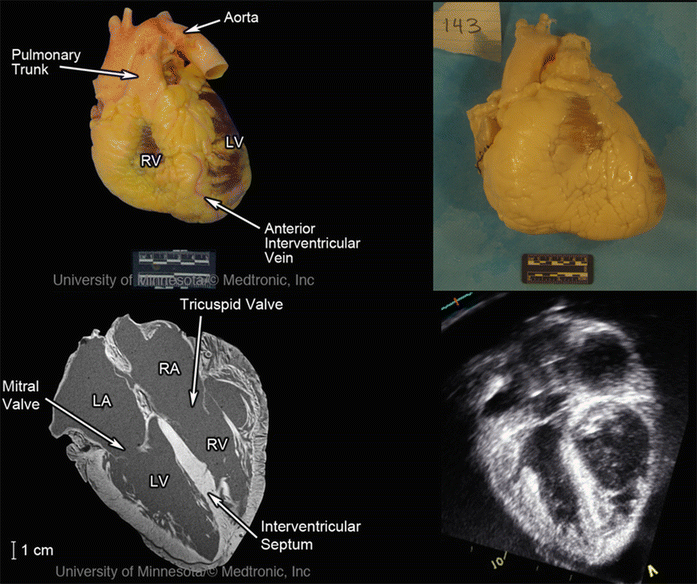
Fig. 41.2
Images of a heart received by the Visible Heart® library and imaged fresh (upper left panel), after perfusion fixation (upper right panel), and scanned in a 3 T Siemens MRI scanner (bottom left panel) and GE Vivid I ultrasound (lower right panel) in the four-chamber long-axis view. Modified from The Atlas of Human Cardiac Anatomy [12]
Recent advances in high-resolution noninvasive cardiac imaging have fostered extensive work in the in vivo analyses of anatomical variations from patient to patient using a variety of imaging modalities:
Nondestructive imaging of specimens from the Visible Heart® library via ultrasound, CT, and MRI has been used to collate a digital database of these hearts for educational and research purposes. The perfusion-fixed specimens are prepared by suspending them in a gel medium, allowing for a full complement of multimodal imaging to be performed on the hearts without changing the orientation [19]. Obtaining high-resolution images has allowed for detailed analyses of cardiac anatomies for a variety of normal and pathologic specimens; this spectrum of imaging is considered not possible with available clinical imaging protocols. For example, the analysis of fiber orientations of specimens obtained from patients in end-stage heart failure, using diffusion tensor MRI [20], provided critical insight into the remodeling of the ventricular tissue in patients with chronic heart failure. In addition, it has been possible to compare the ability of different imaging modalities to assess the anatomical characteristics of specific cardiac pathologies such as aortic stenosis, thus building on the work of other researchers [21, 22]. For additional imaging of such specimens, see Chaps. 6, 7, and 8.
41.4 In Vitro Isolated Heart Models
A comprehensive understanding of the cardiac anatomy provides device designers with fundamental information regarding the anatomical dimensions and variations of the environment into which the device or therapy will be delivered. However, these static human heart specimens do not address the complications surrounding the delivery and function of a device or therapy in a beating heart. Before embarking upon complex and expensive chronic animal testing protocols which are required to prove the efficacy of novel cardiac devices, there is exceptional value in testing in reanimated beating heart models. Our laboratory at the University of Minnesota has reanimated over 1500 large mammalian hearts (canine, ovine, swine, mini-pigs, and human) for such studies in the last 15 years. There have been several other academic institutions and private companies that have developed in vitro large mammalian heart models, with many groups effectively developing systems based upon the mechanical reanimation of cadaveric large mammalian hearts. For example, Richards et al. were able to consistently and reliably quantify mitral regurgitation across a range of severity in explanted porcine hearts and investigate the efficacy of various repair techniques [23]. Further, two other groups have succeeded in studying the electrophysiology of explanted human hearts by sustaining the heart with a pressurized coronary flow of oxygen saturated salt solution via Langendorff perfusion [24, 25]. However, it should be noted that the true reanimation of large mammalian hearts (whereby the heart functions independently of any mechanical or electrical assistance) has only been achieved by a small number of research groups. Araki et al. (Nagoya University, Japan) reported that they were able to complete optical and hemodynamic analyses of cardiac valves in reanimated swine hearts [26]. Most recently, Weger et al. at the Leiden University Medical Center, Netherlands, have monitored transcatheter valve implantations in reanimated swine hearts using their described PhysioHeart system [27]. However, it should be noted that in these preparations, the researchers were limited by the amount of time the heart remained viable, a factor considered key to the accessibility of the heart for device testing.
The Visible Heart® laboratory partnered with Medtronic, Inc. in 1997 to develop the Visible Heart® methodologies, which consist of a large mammalian isolated heart model that can be controlled to function in either Langendorff [25], right-side working, or four-chamber working modes [28]. Over this time and continuing today, we have been developing/optimizing this apparatus for reanimation whereby isolated large mammalian hearts are perfused and then actively pump a clear crystalloid perfusate in the place of blood. Images of a human heart connected to the Visible Heart® apparatus can be seen in Fig. 41.3. This approach has allowed our group to visualize what occurs inside the heart during device deployment procedures and subsequently to determine how such devices interact with the specific anatomies of the heart throughout all the phases of the cardiac cycle.
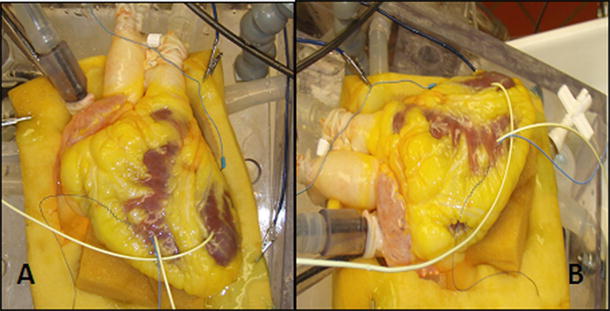
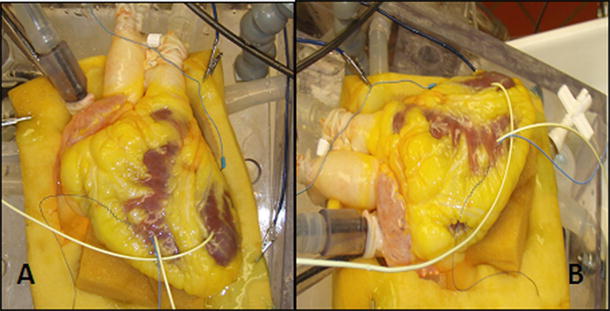
Fig. 41.3
Images of a human heart connected to the Visible Heart® apparatus from an approximation of the anterior-posterior aspect (A) and from the left anterior oblique aspect (B)
Briefly, our approach includes the initial step of removing hearts from humans or animals using standard cardioplegia procedures [28, 29]. Once isolated, cannulae are inserted into the great vessels allowing the placement of endoscopes or devices into all four working chambers. Following reanimation, cardiac and systemic pressures and outputs can be monitored and preloads and afterloads adjusted accordingly to simulate systemic vascular pathologies such as hypertension. Additionally, the isolated heart apparatus allows researchers to quickly switch the perfusion system to operate in Langendorff, right-side working, or four-chamber working modes. During the Langendorff mode, the left-side afterload is held constant with a coronary perfusion pressure of approximately 60 mmHg [28]; thus, the flow through the coronaries is determined by dilation or constriction of the coronary arteries. Right-side working mode combines Langendorff retrograde aortic perfusion with antegrade, or physiologic, flow through the right atrium and right ventricle (adjustable between ~3–5 L/min). During four-chamber working mode, the flow through a heart is normally determined by its intrinsic heart rate, preloads, afterloads, and the relative contractility of the various heart chambers. By controlling the orientation of the heart in our apparatus and determining the preload and afterload pressures exerted on the specimen, we can recreate specific cardiac states. Interestingly, the intrinsic heart rate and hemodynamic performance can be modified by altering the temperature of the buffer or by adding pharmacological agents (e.g., catecholamines or anesthetics), which are discussed later in this chapter. Although no model can perfectly mimic in vivo conditions, to date our apparatus has allowed researchers to simulate a broad range of particular physiological environments that are observed in various clinical settings.
41.5 How Can an Isolated Heart Prep Augment and Complement Bench Top Testing?
The combination of a “live” functional anatomy within a controlled “bench top” experimental setting provides a unique stepping stone between in vitro device testing and in vivo implantation required for implantable medical devices. Figure 41.4 shows how the typical stages of device testing and development compare in terms of the relevance of the testing environment to the intended functional environment, the quantity of data one can reasonably expect to collect, and the cost of performing such investigations. It can easily be observed that as the relevance of a particular testing methodology increases, the relative costs will dramatically increase; thus, the likely number of possible iterations decreases. Consequently, any possible augmentations to device testing prior to chronic animal implants (e.g., via isolated beating heart preps) can, in turn, greatly reduce the overall product development costs and speed clinical use of novel valve repair, implant, and their associated delivery systems.
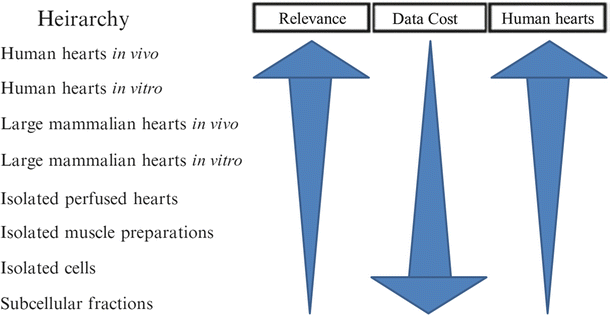
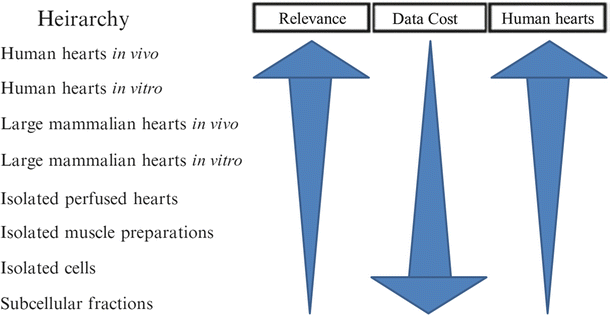
Fig. 41.4
Proposed hierarchy between the relevance of various experimental approaches, the amount of data one can obtain, and the relative costs. For example, if you wish to perform medical device design research in the field of prosthetic valves, ideally you would like to perform human trials in vivo, but this not only raises medical ethical issues but is highly costly and may provide useful data for one specific valve design and procedure. Whereas if you move the research approach downward (i.e., employ an isolated large mammalian heart model in vitro), you can obtain more data at a lower cost, but then you must justify the appropriateness of the chosen model. Yet, in one given study, it may be possible to perform multiple procedures for comparison or at least multiple implants in multiple hearts with fairly consistent anatomies
Due to the large number of cycles seen in the lifetime of a cardiac device, accelerated wear and fatigue testing are required gold standards for the assessment of durability. In accelerated wear testing for bioprosthetic valves , the hydrodynamic conditions are tightly controlled and easily varied allowing the durability of the valve leaflets to be assessed under a variety of predetermined conditions. Similarly, the boundary conditions imposed on the valve frame or commissure posts during fatigue testing can assess frame durability. Isolated heart preparations, including the Visible Heart® methodologies, will never replace these forms of testing, but unique information regarding the device-tissue interactions in the later can be observed. It should be noted that since the hydrodynamics of isolated heart preparations are typically less aggressive environments than what is experienced during accelerated wear testing, the boundary conditions observed for a device in such studies are not directly transferable to accelerated wear test methodologies. Yet on the other hand, they can serve as means to obtain additional information to ascertain the validity of any boundary conditions within the accelerated testing protocol, ensuring that all forms of boundary conditions have been taken into account, i.e., the change in curvature throughout the cardiac cycle of a left-sided pacing lead within the coronary vasculature. Most importantly, such experimentation has provided us with a so-called physiological link between bench top testing of devices and animal testing. Acute phenomena observed during accelerated wear testing and the insights gained with both invasive and noninvasive imaging techniques in animal studies may be directly observed during a device implant study in vitro. For example, a procedural issue observed under fluoroscopy during an in vivo animal implant could be recreated by employing the Visible Heart® approach (under direct visualization) with simultaneous fluoroscopy, thus gaining a better understanding of potential adverse issues. We consider that having the Visible Heart® apparatus as a tool for device design has allowed us to obtain a more rapid understanding of phenomena observed in both bench top and preclinical settings; as such, it is an invaluable tool for a device designer, especially at the early stages of development. See other chapters for addition descriptions and images of cardiac devices that have been implanted in reanimated human hearts [30].
41.6 The Importance of Species Selection in In Vitro Cardiac Device Research
The ultimate utility of studies performed with Visible Heart® methodologies, such as transcatheter valve development , is in part determined by the heart chosen for reanimation. We suggest that the criteria for species selection for acute in vitro studies are slightly different from those for chronic valve assessments, due to the elimination of all systemic factors that may contribute to device performance. In other words, the species of the donor can be chosen specifically for its relative cardiac anatomy rather than for factors such as thrombogenesis, immune response, and/or growth rates.
For years, the canine heart has been used for such experimentation and has provided useful information. Yet it should be recognized that canine hearts have an unusually large amount of collateral coronary circulation (similar to humans in end-stage chronic heart failure), and this in turn results in the inconsistent creation of ischemic (infarct) regions. Sheep have been historically employed for chronic valve implantation studies, as valve function and valve orifice sizes observed in sheep are very similar to those of a human heart. Additionally, the relatively large atria of the sheep’s heart allow for straightforward surgical approaches to the atrioventricular valves. However, it has recently been proposed that swine are an excellent model for acute cardiac device testing, as porcine hearts have very similar anatomy to that of humans with respect to the cardiac valves, conduction system, coronary arteries, and great vessels. Importantly the relationship between the cardiac conduction system and surrounding anatomical features is comparable between swine and human anatomies. Nevertheless, it is important to note that there are some specific variations in animal anatomy that should be known; such interindividual and interspecies variations have been extensively researched [28, 29, 31] and are described in greater detail in Chaps. 6 and 27.
Due to their specific anatomical similarity with human hearts and the relative ease of procurement (excision and reanimation), the mainstay of cardiac research done in the Visible Heart® laboratory is completed using swine hearts . Nevertheless, as previously mentioned, our laboratory has also had the privilege to obtain fresh human heart specimens for reanimation, for both educational and research purposes. Such hearts, if received in a timely manner and with complete anatomies including the great vessels, have been reanimated using the same methodologies as previously described for swine hearts . By reanimating these hearts using a clear perfusate, visualization of the internal cardiac anatomy has provided novel insights into the relative variations of human cardiac anatomy (in healthy individuals) and has highlighted the alterations that occur with various pathologies. Finally, this approach provides the unique opportunity to deliver existing or novel devices within functional human anatomies without the concerns and considerations required in clinical trials; thus, it has allowed researchers to garner invaluable knowledge about their device designs that otherwise could not be generated using animal models.
< div class='tao-gold-member'>
Only gold members can continue reading. Log In or Register a > to continue
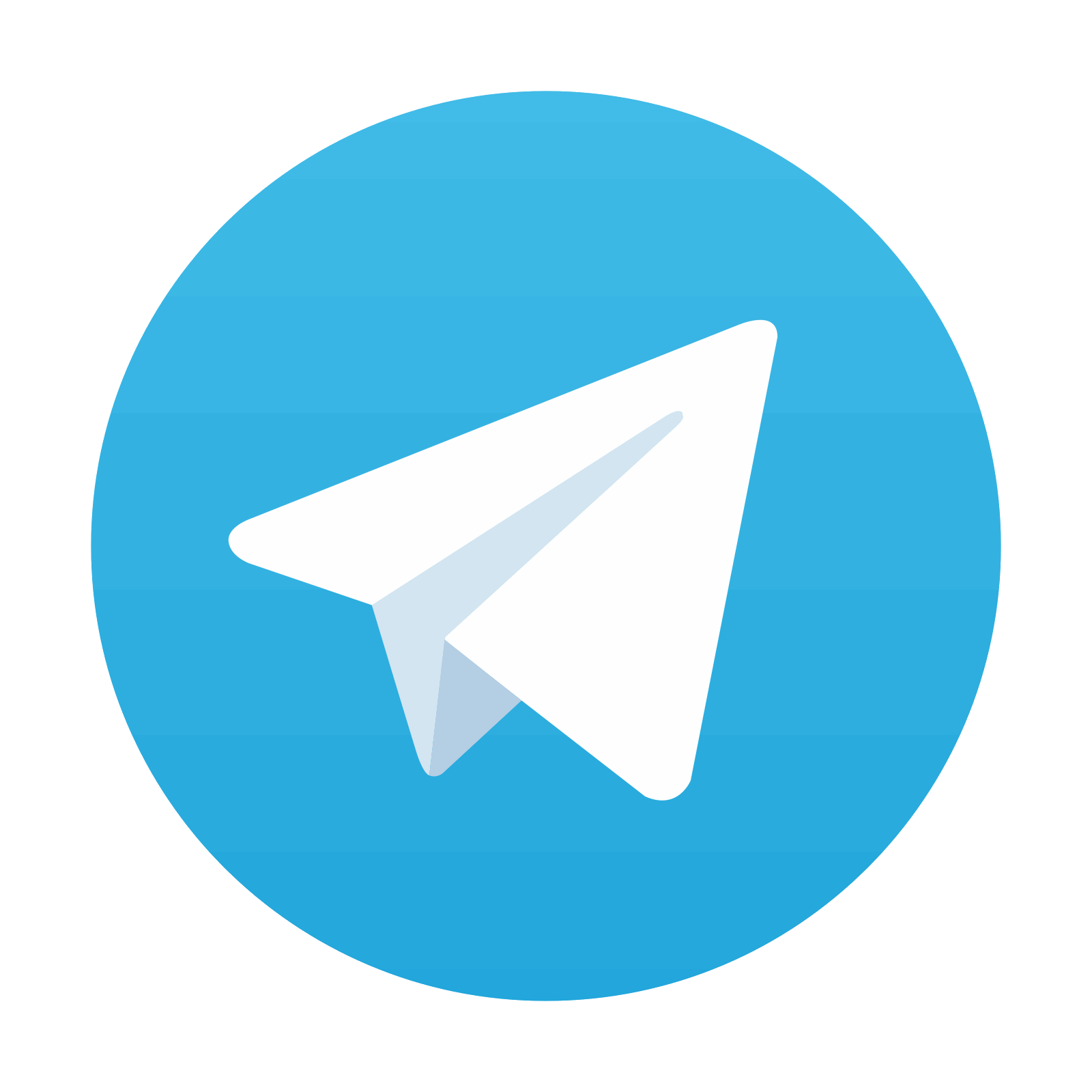
Stay updated, free articles. Join our Telegram channel

Full access? Get Clinical Tree
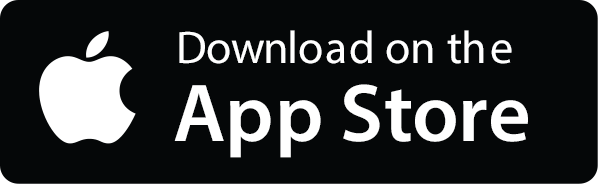
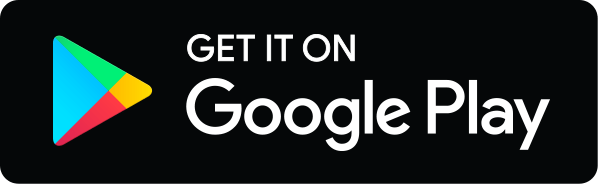