Introduction
Gas exchange between the atmosphere and the human body depends not only on the lungs but also in large part on the function of the “ventilatory pump,” which consists of the respiratory control centers located in the brain, the bony rib cage, diaphragm, and intercostal, accessory, and abdominal muscles. A wide variety of neuromuscular disorders can result in dysfunction of the ventilatory pump that in turn can lead to respiratory failure, pneumonia, and even death. Breathing disorders are recognized as the leading cause of mortality in neuromuscular disease, and appropriate interventions can prevent complications and prolong life in individuals with neuromuscular disease affecting the respiratory system. Diseases of the chest wall are discussed in Chapter 98 .
Functional Anatomy of the Respiratory System
The ventilatory pump is designed to bring oxygen into the body to fuel energy generation and remove carbon dioxide as a waste product of cellular metabolism. The system is made up of the cortex of the brain that controls voluntary breathing; the brain stem, which is involved with automatic breathing; the spinal cord and motor neurons that transmit nerve impulses; the respiratory muscles that are the effectors of the system; and a complex system of feedback receptors and nerves that regulate ventilation ( Fig. 97-1 ). The system is remarkably flexible and can precisely maintain carbon dioxide and acid-base balance despite encountering large variations in metabolic demands that result from the activities of daily living. The following is a discussion of each of the components of this complex network.

Central Nervous System
Voluntary Breathing Controllers
Voluntary breathing is controlled by signals from the cerebral cortex. Centers located within the parietal cortex send volitional signals that initiate inspiration and expiration. These cortical areas project to the motor neurons in the spinal cord via the corticospinal tracts. These corticospinal tracts are separate pathways from the reticulospinal pathways that connect the central automatic breathing centers to the motor neurons.
Automatic Breathing Controllers
Automatic breathing is controlled by a complex system that includes respiratory centers in the pons and medulla, nerve tracts in the lower brain stem, the reticulospinal pathways in the spinal cord, and the feedback mechanisms that are both chemical and mechanical in nature. There are thought to be three centers that generate the rhythm and drive to breathe, one located in the pons and two in the medulla. More detailed reviews of this topic are available.
Spinal Cord
The spinal cord and motor nerves conduct nerve impulses from the cortex and brain stem to the anterior horn cells of the motor neurons supplying the respiratory muscles. As noted previously, the nerve fiber tracts in the spinal cord responsible for voluntary (corticospinal tract) and automatic (reticulospinal tract) breathing are separate within the spinal cord. The fibers in both of these tracts travel through the spinal cord to synapse with the lower motor neurons.
Peripheral Nervous System
Lower Motor Neurons
The lower motor neuron has its cell body in the spinal cord (anterior horn cell) but exits the spinal cord to become the spinal nerve roots and the nerves that supply the respiratory muscles. When the nerves arrive at the muscle, they divide into branches known as twigs that, upon reaching the muscle fiber, further divide into bulbous projections called boutons that apply themselves to the muscle membrane at a specialized anatomic junction called the motor end plate. These boutons contain acetylcholine, which is the chemical transmitter that serves to excite the muscle to contract. With nerve firing, there is release of acetylcholine at the motor end plate into the cleft between the nerve and the muscle. The acetylcholine binds to receptors on the muscle side of the motor end plate, which results in a suprathreshold excitatory end plate potential and depolarization of the muscle membrane. A muscle action potential then results in contraction of the muscle fiber.
Total Lung Capacity
Respiratory Muscles.
The respiratory muscles are the mechanical effectors of the breathing system. They are often divided into three major groups: (1) the inspiratory muscles, (2) the expiratory muscles, and (3) the accessory muscles of respiration. The muscles that maintain patency of the upper airway during the respiratory cycle are sometimes also considered muscles of respiration because of their close interaction with the other respiratory muscles.
The diaphragm is the major muscle of inspiration and accounts for approximately 70% of the inhaled tidal volume in the normal individual ( Fig. 97-2 ). Contraction of the diaphragm results in a downward piston motion of the muscle as well as outward and upward movement of the ribs through the zone of apposition. The innervation of the diaphragm is via the phrenic nerve that originates from cervical nerve roots 3 through 5.

The intercostal muscles are thin sheets of muscular fibers that run between the ribs in the costal spaces. There are two sheets of muscle fibers, the external and internal intercostals. The external intercostals function to expand the rib cage during inspiration. The internal intercostals are deeper and function to decrease rib cage size during expiration. The mechanism of action of the intercostal muscles on the ribs is shown in Figure 97-3 . The orientation of the muscle fibers with respect to the ribs results in the increase or decrease in the size of the rib cage; as the muscles contract, the greater torque is applied to the point more distal from the spine. In the case of the external (inspiratory) intercostal muscles, the distal attachment is at the lower rib, so contraction tends to pull the lower rib upward, thereby expanding the chest; for the internal (expiratory) intercostal muscles, the distal attachment is at the upper rib and thus contraction of the muscle tends to pull the upper rib down, thereby decreasing the chest. Innervation of the intercostals is via the intercostal nerves originating from the thoracic spinal nerve roots.

The abdominal muscles (rectus abdominis, internal oblique, external oblique, and transversus abdominis) serve a number of functions in respiration that mainly assist expiration but can also function in inspiration. The internal and external obliques and the transversus abdominis result in an inward movement of the abdominal wall that displaces the diaphragm upward into the thoracic cavity and assists exhalation. The rectus abdominis as well as the internal and external obliques pull the lower rib cage caudally and thereby increase pleural pressure and exhalation. The abdominal muscles also may play a minor role in inspiration ; if their contraction reduces lung volume below function residual capacity (FRC), abdominal muscles can store elastic recoil energy in the chest wall that then assists expansion of the chest wall during the next inspiration. This “inspiratory assist” may be seen during exercise where expiration becomes active.
The accessory muscles of respiration (sternocleidomastoid, scalenes, trapezii, latissimus dorsi, platysma, and pectoralis major and minor muscles) can expand the rib cage and assist inspiration during situations of increased ventilatory demand such as during exercise or when other inspiratory muscles are impaired as in tetraplegia or chronic obstructive pulmonary disease. It is now clear that some of them function during minimal exertion and even during quiet tidal breathing.
The muscles of the upper airway are also considered to be muscles of respiration because they maintain patency of the upper airway and allow air to flow into and out of the lungs without interruption. Some of these also participate in protection of the lower airway during swallowing, a key function in the defense of the respiratory system. The upper airway muscles include the abductors of the vocal cords, the palatal elevators, retractors of the tongue, and dilators of the nares. These muscles are innervated by cranial nerves V, VII, and IX-XII. The central control centers for these muscles are the same as those described previously for the more commonly considered ventilatory muscles.
Controller Feedback
The respiratory control mechanisms depend on both neural and chemical receptors found in peripheral and central sites. An excellent discussion of this topic is available. The automatic respiratory centers in the brain stem respond to feedback from these receptors and adjust neural output to the ventilatory and upper airway muscles that expand the chest wall and maintain upper airway patency (see Fig. 97-1 ).
Neural receptors are present in the upper airway, respiratory muscles, lungs, and pulmonary vessels. Once stimulated, these receptors project signals to the central respiratory controller via the vagus nerve. The respiratory centers then adjust their output to the respiratory muscles to alter ventilation and modulate reflexes such as cough and sneeze. There are several different types of neural receptors. Muscle spindles and slowly adapting pulmonary stretch receptors predominantly respond to changes in thoracic cage and lung volume, respectively. As the chest wall and lung inflate, these receptors are stretched and dampen the output from the inspiratory centers in the medulla. These receptors are involved in the “Hering-Breuer” reflex in which inspiration is halted as lung volume increases. These are critical for initiating cough. Rapidly adapting irritant receptors respond to changes in lung volume, to chemical stimuli such as histamines and prostaglandins, and to noxious stimuli. C fibers in the airways and lung are stimulated by chemical stimuli in the local environment. The C fibers likely mediate the hyperventilation that is seen in normoxic circumstances in a number of pulmonary disorders such as asthma, pulmonary embolism, pneumonia, and pulmonary edema.
Chemical receptors or chemoreceptors are located both peripherally as well as in the central nervous system. The peripheral chemoreceptors, which include the carotid and aortic bodies, are the primary site for sensing low levels of arterial oxygen pressure (arterial P o 2 ) but also respond to a lesser extent to changes in arterial carbon dioxide pressure (arterial P co 2 ) and pH. They are stimulated when the arterial P o 2 falls below 75 mm Hg as well as when the arterial P co 2 increases and pH decreases. Whereas both receptors stimulate ventilation in hypoxia, the aortic chemoreceptors are more important in infants and the carotid receptors are more important in adults. Once stimulated, the carotid bodies send impulses via cranial nerve IX to the nucleus tractus solitarius where neurotransmitters are released that increase ventilation. Although the control of ventilation during exercise is not completely understood, the predominant stimulus for increased ventilation is thought to arise from the peripheral receptors. It is postulated that additional peripheral receptors exist that are yet to be identified because the hyperventilatory response of exercise cannot be fully accounted for by the carotid bodies. The central chemoreceptors are crucial in the adjustments of ventilation to acid-base disturbances and arterial P co 2 . There are four groups of chemosensitive neurons in the brain stem: the locus ceruleus, the nucleus tractus solitarius, the midline raphe, and the ventrolateral quadrant of the medulla. The central chemoreceptors are responsible for most of the response to carbon dioxide. They respond to increases in arterial P co 2 by their detection of the fall in pH of the cerebrospinal fluid associated with an increase in cerebrospinal fluid arterial P co 2 , which closely follows an increase in serum arterial P co 2 . Of note, it appears that the parasympathetic nervous system is important in the response to cerebrospinal fluid pH changes because inhibition of acetylcholine transmission can abolish the central pH response in animals.
Diseases Affecting the Respiratory System
The diseases of the neurorespiratory system can be organized most logically in the framework of functional anatomy. Diseases of the central nervous system known to affect the respiratory system are listed in Table 97-1 and those affecting the peripheral nervous system are listed in Table 97-2 .
Cerebral Cortex | Brain Stem/Basal Ganglia | Spinal Cord |
---|---|---|
Stroke | Stroke | Trauma |
Neoplasm | Neoplasm | Infarction or hemorrhage |
Cerebral degeneration Seizures | Poliomyelitis | Demyelinating disease |
Central alveolar hypoventilation | Disc compression Syringomyelia Tumor Epidural abscess | |
Progressive bulbar palsy | ||
Multiple system atrophy | ||
Anoxic encephalopathy | ||
Encephalitis | ||
Multiple sclerosis | ||
Parkinson disease | ||
Chorea | ||
Dyskinesias |
Motor Nerves/Anterior Horn Cell | Neuromuscular Junction | Myopathies |
---|---|---|
Acute idiopathic demyelinating polyneuropathy (Guillain-Barré syndrome) Motor neuron disease Amyotrophic lateral sclerosis Spinal muscular atrophy Primary lateral sclerosis Critical illness neuropathy Vasculitides Toxins (e.g., lithium, arsenic, gold) Metabolic Diabetes Porphyria Uremia Diphtheria | Myasthenia gravis Lambert-Eaton myasthenic syndrome Toxins Botulism Snake venoms Scorpion bites Shellfish Crab poisoning Drugs Antibiotics Neuromuscular junction blockers Anticholinesterase inhibitors Corticosteroids Lidocaine Quinidine Lithium Antirheumatics | Muscular dystrophies Myotonic dystrophy Poly- and dermatomyositis Glycogen storage diseases Pompe disease Forbes-Cori disease Thick filament myopathy Mitochondrial myopathy Nemaline body myopathy Severe hypokalemia Hypophosphatemia |
Central Nervous System Diseases
Cortical and Brain Stem Disorders
Diseases of Voluntary Breathing.
The pathways that connect the voluntary respiratory centers of the cortex with spinal motor neurons (corticospinal tracts) can be affected in a number of disorders. Midpontine strokes can damage the corticospinal tracts and cause what is known as the “locked-in syndrome,” a syndrome with total paralysis with the exception of eye movement. Because injury to the reticulospinal tracts causes loss of volitional but not automatic breathing, the response to automatic breathing is preserved. This syndrome is most commonly due to ischemic stroke but can be due to pontine tumor, central pontine myelinolysis, high cervical demyelination, syphilitic arteritis, and head injury. Extrapyramidal disorders such as parkinsonism can also affect voluntary breathing. In these disorders, patients have less ability to alter their breathing pattern voluntarily. They also may demonstrate periodic breathing or a Cheyne-Stokes respiratory pattern.
Diseases of Automatic Breathing.
Automatic breathing but not voluntary breathing is classically disrupted in central alveolar hypoventilation, otherwise known as “Ondine’s curse,” caused by injury to the automatic respiratory centers in the brain stem. Because automatic triggering controls breathing during sleep, individuals with central hypoventilation develop central sleep apnea. Congenital central alveolar hypoventilation is a rare genetic disorder affecting infants and children, although it has now been described in adults as well. Acquired central alveolar hypoventilation can result from unilateral or bilateral medullary infarction, bulbar poliomyelitis, or bilateral cervical tractotomy performed to control chronic pain. Although disorders of the neurorespiratory system generally lead to hypoventilation and the need for ventilatory support, diseases of the automatic respiratory centers in the brain stem can also lead to hyperventilation. This may be caused by diseases such as central nervous system infection or tumor or by stimulation of an otherwise normal autonomic respiratory center by fever, sepsis, pain, pregnancy, medications such as progesterone or salicylates, or high altitude. A variety of irregular breathing patterns have also been associated with central nervous system disease including Cheyne-Stokes respiration and ataxic breathing.
Diseases of the Spinal Cord
Spinal Cord Injury.
Spinal cord injury (SCI) is most commonly due to traumatic injury caused by motor vehicle accidents, falls, sports accidents, and gunshot wounds. In the United States, an estimated 250,000 individuals have suffered significant SCI; the incidence is approximately 12,000 incident cases per year with 78% of the injuries seen in males (National Spinal Cord Injury Statistical Center http://www.spinalcord.uab.edu ). Respiratory complications including atelectasis, pneumonia, and respiratory failure are the leading cause of morbidity and mortality for individuals with SCI. Immediately following acute SCI, a number of physiologic changes can affect the respiratory system and predispose to respiratory complications. These aberrations include (1) cough inefficiency due to expiratory muscle weakness, (2) ciliary dysfunction, (3) mucous hypersecretion possibly due to impairment of the peripheral autonomic nervous system, (4) glottic dysfunction or gastric hypomotility increasing the risk of aspiration, (5) bronchial hyperreactivity due to sympathectomy, and (6) loss of consciousness at the time of the injury, increasing the risk of aspiration.
Acute respiratory failure immediately after the injury may be due to direct disruption of respiratory muscle innervation (cervical cord injury), aspiration at the scene due to loss of consciousness, pulmonary edema, or other traumatic injuries to the chest or head. Many individuals with SCI are intubated at the scene of the accident or shortly thereafter. Prevention of further respiratory complications is important. Preventive measures include encouragement of deep breathing and cough, frequent changes of position, postural drainage, assisted cough (manual or mechanical), and bronchoscopy when other measures fail. Patients with SCI are at high risk for venous thromboembolism with the greatest risk 3 days to 2 weeks after injury. Prophylaxis for deep venous thrombosis is highly recommended during the initial hospitalization.
The extent of respiratory muscle impairment following SCI mainly depends on the level and severity of the spinal injury because the function of respiratory muscles innervated below the level of the cord injury is lost. The innervation of the respiratory muscles is shown in Table 97-3 . The overall pattern of pulmonary function abnormality is one of restriction. In the first few months to a year following the initial SCI, there is a significant improvement in lung volumes and maximal inspiratory and expiratory pressures, possibly due to resolution of spinal cord edema and/or strengthening of the diaphragm and accessory muscles of inspiration.
Muscle Group | Spinal Cord Level | Nerve |
---|---|---|
INSPIRATORY MUSCLES | ||
Diaphragm | C3-5 | Phrenic |
Parasternal intercostals | T1-7 | Intercostal |
Lateral external intercostals | T1-12 | Intercostal |
Scalenes | C4-8 | Cervical (deep branches) |
Sternocleidomastoids | Above spinal cord | Spinal accessory |
EXPIRATORY MUSCLES | ||
Lateral internal intercostals | T1-12 | Intercostal |
Rectus abdominis | T7-L1 | Lumbar |
External and internal obliques | T7-L1 | Lumbar |
Transversus abdominis | T7-L1 | Lumbar |
UPPER AIRWAY MUSCLES | ||
Muscles of mastication | – | CN V, VII |
Laryngeal and pharyngeal | ||
Abductors | – | CN IX-XII |
Adductors | – | CN IX-XII |
In general, the higher the SCI, the more likely that ventilatory assistance will be needed. Treatment of ventilatory failure following SCI can be accomplished by invasive (tracheostomy) or noninvasive (mask or mouthpiece) methods. Many individuals are ventilated through a tracheostomy tube for some time after their injury; however, transfer to noninvasive means can often be achieved even for those requiring full-time ventilatory support. The choice of invasive versus noninvasive ventilation is determined by the degree of local expertise with these forms of ventilation as well as by patient preference. For those with high cervical SCI (C1-2) and intact phrenic nerve function, diaphragm pacing either via a laparoscopic approach or thoracic approach is also a possibility. It should be noted that those with diaphragm pacemakers implanted still need a tracheostomy in place to avoid airway obstruction during sleep owing to the absence of the normal neurologic coordination of diaphragm contraction and upper airway dilation during inspiration. Although traumatic SCI is the major cause of spinal cord pathology, other causes include vascular accidents, demyelinating diseases (multiple sclerosis, transverse myelitis), syringomyelia, tumors, and epidural abscess (see Table 97-1 ).
Ventilator dependence in patients with SCI is more common with high (cervical) SCI. Because the diaphragm is the major muscle of inspiration (C3-5 spinal nerve roots), those with lesions at C3 and above invariably require ventilatory support and are at the highest risk of respiratory complications. Those with injuries between C3 and C5 vary in their requirements for ventilatory support. Even if they require ventilation initially, these individuals may become independent of ventilatory support eventually. The risk for ventilator dependence in this group of patients is greatest for those with injury close to C3 or age older than 50 years. Individuals with lesions below C5 almost always become independent of continuous ventilator support. In contrast to inspiratory muscle function, expiratory muscle function is impaired after all levels of cervical cord injury resulting in expiratory muscle weakness and ineffective cough. Because an effective cough depends in large part on abdominal and intercostal muscle function (spinal nerve roots T1-L1), cervical, thoracic, and even some high lumbar SCIs can impair the ability to cough and clear secretions. Therefore, measurement of cough function in these patients is very important.
Other Respiratory Effects of Spinal Cord Injury.
For unknown reasons, the frequency of sleep apnea appears to be increased by as much as fourfold in patients with SCI compared to a normal population of similar age. A sleep study should be considered when symptoms of nocturnal hypoventilation such as morning headache, daytime hypersomnolence, or unexplained nocturnal awakenings are present or when daytime hypercarbia (arterial P co 2 > 45 mm Hg), unexplained cor pulmonale, or forced vital capacity (FVC) less than 50% predicted are present. Bronchial hyperreactivity and bronchial hypersecretion are also present in some individuals following SCI likely due to interference with normal autonomic control of the airways after injury.
Peripheral Nervous System Diseases
Diseases of Motor Nerves or Anterior Horn Cells and Diseases of the Neuromuscular Junction
Disorders of the motor nerves and the neuromuscular junction (NMJ) can arise acutely as in acute inflammatory polyneuropathy (Guillain-Barré syndrome) or botulinum toxicity or can arise more chronically as in motor neuron disease (e.g., amyotrophic lateral sclerosis [ALS]) or myasthenia gravis (see Table 97-2 ). The level of motor nerve root involvement dictates the effects of the disorder on the respiratory system.
Acute Diseases Affecting Motor Nerves
Acute Immune-Mediated Polyneuropathy.
Acute immune-mediated polyneuropathy, also referred to as Guillain-Barré syndrome, is a heterogeneous group of diseases that are now thought to be caused by antiglycolipid antibodies. Acute idiopathic demyelinating polyneuropathy is the most common form, making up about 85% to 90% of cases. It is an acute progressive process characterized by symmetrical, ascending muscle weakness progressing over a 2-week period and associated with loss of deep tendon reflexes. It is thought that an antecedent viral infection induces an immune response to viral epitopes that then cross-react with lipid components of the peripheral nerve. The process usually starts in the lower extremities but may start in the arms or the face, head, and neck primarily (Miller-Fisher variant).
Acute idiopathic demyelinating polyneuropathy affects the respiratory system by causing (1) weakness of the upper airway muscles, (2) weakness of the inspiratory and expiratory muscles, and (3) secondary complications such as pneumonia or pulmonary embolism. Approximately 25% to 50% of patients develop respiratory insufficiency severe enough to necessitate intubation and mechanical ventilation. A number of variables used to predict impending respiratory failure and the need for mechanical ventilatory support have been used including FVC, maximal inspiratory pressure (MIP), maximal expiratory pressure, and nocturnal desaturation. Generally accepted guidelines for intubation and mechanical ventilation include an FVC less than 15 mL/kg, an FVC less than 1 L, a decline in FVC to 50% or less of the normal predicted value for that individual or an MIP of 30 cm H 2 O or less. FVC and MIP should be monitored frequently (multiple times per day depending on rapidity of progression). With rapidly progressive disease, the intensive care unit (ICU) is considered the best location for monitoring. Absolute indications for intubation include impaired consciousness, respiratory or cardiac arrest, shock, arrhythmias, blood-gas alterations, or bulbar dysfunction with confirmed aspiration. Noninvasive ventilation is generally not an option for these individuals because they are at a high risk for aspiration because of bulbar muscle involvement. Successful extubation may be achieved once treatment such as steroids, immune globulin, and plasmapheresis is begun and respiratory muscle strength returns. Until respiratory muscle strength returns, scrupulous supportive ICU care including tracheostomy for prolonged mechanical ventilation is required to prevent complications such as ventilator-associated pneumonia and sepsis.
Poliomyelitis.
Poliomyelitis is a viral disease affecting the anterior horn cell and therefore motor nerve caused by a human enterovirus that caused significant epidemics with frequent respiratory complications in the United States and Western Europe during the 20th century. Owing to improved sanitation and vaccine development, polio is no longer a major public health issue in the developed world. However, in the developing world, acute infections still happen.
In the developed world, the major health issue arising from polio currently is the postpolio syndrome, in which new weakness develops in survivors of the polio epidemics of the mid-20th century. The cause of progressive neurologic deterioration in postpolio syndrome is unknown. Theories of pathogenesis suggested include (1) progressive degeneration of reinnervated motor units, (2) persistence of poliovirus in neural tissue, or (3) induction of autoimmunity with subsequent destruction of neural structures. The average time to onset from the time of the initial infection is 35 years but ranges from 8 to 71 years. A high percentage of polio survivors, between 20% and 60% of individuals, complain of new muscle weakness. Although the exact incidence of respiratory system involvement in postpolio syndrome is not known, sleep-disordered breathing is common and noninvasive nocturnal ventilation can be highly effective. Fortunately, it appears that frank hypoventilation associated with the postpolio syndrome is unusual.
Chronic Diseases Affecting Motor Nerves
Amyotrophic Lateral Sclerosis.
Amyotrophic lateral sclerosis (ALS) is a progressive neurodegenerative disease with no known cure. The usual clinical presentation is that of an individual with gradually progressive asymmetrical weakness associated with hyperreflexia due to upper motor nerve involvement and muscle fasciculations due to lower motor nerve involvement. Like most neurologic diseases, ALS has no direct effect on the lung; however, it significantly affects the upper airway, chest wall, and diaphragm, thereby impairing respiratory muscle function. Indeed, ALS affects all of the major muscle groups of the respiratory system including (1) upper airway muscles leading to abnormal swallowing and inadequate cough, (2) expiratory muscles leading to inadequate cough, and (3) inspiratory muscles leading to inadequate ventilation. Therefore, all patients with ALS are at significant risk for respiratory complications. Respiratory failure, the leading cause of death in this population, has been reported as the initial clinical presentation of ALS. Patients may complain of dyspnea, difficulty managing secretions, or inability to cough effectively. Monitoring pulmonary function in the outpatient setting is crucial. The FVC is the most commonly used measurement and has prognostic significance. Attention must be given to glottic and cough function as well because aspiration and pneumonia are significant contributors to morbidity and mortality. Sleep-disordered breathing is very common in ALS and noninvasive positive-pressure ventilation (NPPV) has been used both at night and, as the disease progresses, during the day to improve quality and duration of life. Patients often have difficulty managing secretions owing to swallowing dysfunction. Secretions can be reduced by using anticholinergic agents, by low-dose irradiation of salivary glands, or by injecting the glands with botulinum toxin. As ALS progresses, NPPV ceases to be effective and a decision needs to be made concerning the appropriateness of tracheostomy and long-term invasive ventilation. Although tracheostomy can prolong survival substantially, it does not alter the progression of the disease and patients ultimately become completely paretic. Due to different cultural approaches, rates of invasive ventilation vary substantially among countries.
Neuromuscular Junction Diseases
Myasthenia Gravis.
Myasthenia gravis is the most common disease affecting neuromuscular transmission. It is an autoimmune disease characterized by an antibody-mediated immune attack directed at acetylcholine receptors and/or receptor-associated proteins in the postsynaptic membrane of the NMJ. It causes weakness of several muscle groups including the respiratory muscles. The respiratory muscles are particularly susceptible to fatigue during the severe, potentially life-threatening, exacerbations known as myasthenic crises. The clinical considerations during myasthenic crisis are very similar to those for acute idiopathic demyelinating polyneuropathy noted earlier and the indications for intubation are the same. Acute treatment of myasthenic crisis focuses on rapid therapies including intravenous immunoglobulin as well as plasmapheresis and corticosteroid therapy. Assiduous attention to respiratory care provides support of the patient, allowing time for therapy of the underlying myasthenia to be effective.
Myasthenia gravis can also arise as a paraneoplastic syndrome in association with thymoma. It has been estimated that approximately 15% of all patients with thymoma will exhibit myasthenia gravis. Removal of the thymoma may result in amelioration of the myasthenia symptoms. Predictors of improvement after thymectomy have been reported to be age younger than 35 years, less than 24 months from the onset of symptoms, and absence of perioperative steroid use.
The Lambert-Eaton myasthenic syndrome (LEMS) is a myasthenic syndrome associated with small cell lung cancer that can affect the respiratory muscles in a fashion similar to that of myasthenia gravis. In patients with small cell lung cancer, LEMS may be present in approximately 3% of cases. However, in patients with LEMS, as many as 50% to 60% will have a tumor, almost always a small cell lung cancer. The small cell lung cancer may be occult and should be sought for up to 5 years after the diagnosis of LEMS. LEMS is thought to be due to autoantibodies directed against presynaptic voltage-gated calcium channels, thereby interfering with synaptic function. These voltage-gated calcium channels may be shared by the neuroendocrine cells of the small cell lung cancer, thus explaining the association of the two conditions. Although respiratory involvement is often a late finding, frank respiratory failure can be a manifestation of LEMS, and this disorder should be considered in individuals with unexplained neuromuscular weakness. Although LEMS shares a similar pathophysiologic mechanism with myasthenia gravis, the clinical presentation is different; LEMS is characterized by (1) an increase in the compound muscle action potential with repetitive nerve stimulation, a feature not seen in myasthenia, (2) more frequent presence of proximal leg weakness, which is worse in the morning, (3) greater autonomic dysfunction, and (4) frequent association with malignancy.
Botulism.
Botulism is a neuroparalytic syndrome caused by a toxin produced by the gram-positive bacterium Clostridium botulinum that acts at the NMJ. It can be acquired by humans in one of five ways: (1) food-borne botulism, in which there is ingestion of food contaminated with preformed toxin, (2) wound botulism, in which the bacteria growing in the wound produce toxin in vivo, (3) infant botulism, in which there is ingestion of clostridial spores allowing colonization of the host’s gastrointestinal tract by the bacteria and production of the toxin in vivo, (4) adult enteric infectious botulism, which is similar to infant botulism, and (5) inhalational botulism, which follows inhalation of aerosolized toxin released in an act of bioterrorism (see Chapter 40 ).
C. botulinum produces a number of toxins that can produce paralysis by binding to synaptotagmin I and II receptors that mediate toxin entry into the nerve cell cytoplasm. Once intracellular, the toxin produces irreversible disruption in stimulation-induced acetylcholine release. The botulinum toxin is one of the most potent toxins known. Recovery from the injury requires regrowth of new synapses, a process than can take 6 months. The clinical syndrome is one of progressive paralysis with early cranial nerve involvement causing blurred vision, dysphagia, dysarthria, and facial weakness. Descending muscle weakness is the usual course and involvement of the upper airway, diaphragm, and intercostal muscles often leads to the requirement for intubation and mechanical ventilation. All patients should be monitored in the ICU and intubation should be undertaken for those who cannot protect their airway or who have a vital capacity of less than 30% predicted. Botulism antitoxin (intravenous botulinum immune globulin) is available for use in the United States and is given to infants younger than 1 year of age. For adults in the United States, an investigational equine serum heptavalent botulism antitoxin is available through the Centers for Disease Control and Prevention. For all patients, supportive care is the mainstay of therapy, and when necessary, intubation and mechanical ventilation are critical to improved survival. Prolonged hospitalization is common.
OTHER NEUROMUSCULAR JUNCTION TOXINS.
A number of other toxins can affect the NMJ. The insecticides organophosphates and carbamates are potent inhibitors of acetylcholinesterase, leading to high levels of acetylcholine in the NMJ and resulting in parasympathetic and sympathetic overstimulation, skeletal muscle paralysis, and sometimes respiratory failure. Several species of tick produce toxins that can inhibit NMJ neurotransmission (tick paralysis) by inhibiting influx of sodium ions and preventing presynaptic terminal axon depolarization and the release of acetylcholine at the nerve terminal. Snake venom from a number of families of snakes produces neurotoxins that can cause paralysis, respiratory failure, and death owing to inhibition of neurotransmission. Finally, a wide range of medications can cause NMJ blockade including D-penicillamine, aminoglycoside antibiotics, fluoroquinolone antibiotics, phenytoin and other anticonvulsants, lithium, β-blockers, glucocorticoids, and magnesium sulfate.
Diseases of the Respiratory Muscles
A large number of disorders, both acute and chronic, can directly affect the respiratory muscles (see Table 97-2 ). Many causes of chronic muscle disease result in respiratory muscle dysfunction including genetic muscular dystrophies, myopathies, and myotonias as well as inflammatory myopathies and those associated with systemic diseases.
Duchenne and Becker Muscular Dystrophies.
Duchenne and Becker muscular dystrophies are both progressive myopathic disorders caused by mutations of the dystrophin gene on chromosome Xp21. Dystrophins are glycoproteins that coat sarcolemmal, sarcomeric, and cytosolic muscle proteins. In Duchenne muscular dystrophy, dystrophin is absent, whereas in Becker dystrophy, a milder variant, dystrophin is reduced in quantity or quality. Both disorders are inherited as X-linked recessive traits and are characterized by progressive muscle wasting and weakness. Typically, weakness of limb muscles is more severe in Duchenne muscular dystrophy. Both diseases can involve the respiratory muscles, leading to substantial morbidity and mortality. The diaphragm, however, may be less susceptible than limb muscle because of different gene transcription patterns. Duchenne dystrophy can also involve cardiac muscle.
In Duchenne muscular dystrophy, weakness of proximal muscle groups usually manifests by age 2 to 3 years, while weakness of the respiratory muscles becomes evident later in childhood. In Becker muscular dystrophy, weakness manifests later in childhood and individuals can remain ambulatory often into adult life. Because limb muscles are more affected than the respiratory muscles, exercise is initially limited by limb muscle weakness rather than dyspnea due to respiratory muscle weakness. However, with time, the respiratory muscles progressively weaken and dyspnea may appear with minimal exertion or at rest. Inspiratory muscle weakness can be assessed by measuring MIP. In children unable to perform this maneuver, maximal sniff pressures may provide a useful surrogate. With severe inspiratory muscle weakness (MIP < 50% predicted), lung volumes are reduced and vital capacity may decline at a rate of 6% to 11% per year. This restrictive process can be worsened by concurrent scoliosis, kyphosis, or muscle contractures. Glucocorticoids and idebenone, a quinone-like drug that may enhance energy production at the mitochondria and has antioxidant properties, have been used to slow the rate of decline of lung function. Therapies based on genetic manipulation such as gene transfer or exon skipping to restore the proper reading frame show promise but need further evaluation.
The diagnosis is often suspected by noting an elevated serum creatine kinase. However, this finding is not specific for these disorders. Often, a muscle biopsy is obtained to distinguish muscular dystrophies from inflammatory skeletal muscle disorders and other forms of dystrophy. With muscular dystrophy, there is degeneration and regeneration of muscle fibers as well as replacement of muscle by fat and connective tissue. Immunoblots for dystrophin can aid in distinguishing Duchenne from Becker muscular dystrophy. Analysis for deletions in the dystrophin gene may be useful, but detection of point mutations of this gene may be difficult. Female siblings of individuals with Duchenne or Becker muscular dystrophy should be tested to determine whether they are carriers of the disease. Carriers often have elevated creatine kinase levels. It is important to identify carriers not only for genetic counseling but also because they may be at higher than average risk for development of cardiomyopathy.
The combination of a restrictive pulmonary deficit and expiratory muscle weakness impairs cough and therefore predisposes these individuals to development of respiratory complications such as atelectasis and pneumonia. Methods for preventing atelectasis and infection are discussed later in this chapter.
As the inspiratory muscles further weaken, the restrictive lung dysfunction becomes severe, with total lung capacity and vital capacity dropping to less than 60% predicted. Individuals with a vital capacity less than 30% predicted are at high risk for nocturnal hypoventilation. These individuals often experience symptoms related to nocturnal hypoventilation such as non-refreshing sleep and fatigue. Nocturnal ventilation is usually initiated if symptoms are related to nocturnal hypoventilation, right heart failure, or elevated arterial P co 2 (>45 mm Hg) either at night or during the day. In general, daytime measures of respiratory muscle strength or lung volumes are poor predictors of nocturnal hypoventilation and overnight oximetry or a sleep study should be considered in high-risk individuals Noninvasive ventilation is a therapeutic intervention that typically can be delivered with a positive-pressure device using an interface such as a nasal mask, nasal-oral mask, or mouthpiece (discussed later). Although there are no randomized controlled trials in patients with muscular dystrophy, noninvasive ventilation is likely to improve quality of life, physical activity, and hemodynamics and to normalize arterial blood gases. Extension of noninvasive ventilation into waking hours may provide relief of dyspnea in more severely affected patients. Endurance and strength training targeting the inspiratory muscles may slow the progression of the disease in individuals with mild to moderate weakness. Individuals with Duchenne or Becker muscular dystrophy who require surgery may be at higher risk for respiratory complications. A consensus statement has been published addressing the respiratory and other risks of anesthesia and suggesting methods for avoiding excessive morbidity and mortality.
Other muscular dystrophies such as limb girdle muscular dystrophy, fascioscapulohumeral dystrophy, and myotonic dystrophy can affect the respiratory muscles but do not generally cause respiratory impairment until later in the course of the disease.
Chronic Inflammatory Myopathies.
Dermatomyositis (DM), polymyositis (PM), and inclusion body myositis (IBM) are systemic inflammatory diseases of unknown etiology that cause profound skeletal muscle weakness. PM and DM, with a prevalence of roughly 1 : 100,000, affect females more than males. Both DM and PM are characterized by muscle fiber inflammation and necrosis. With DM, the inflammatory process may be due to complement-mediated microangiopathy or related to type 1 interferons. The locus of inflammation in DM is the perifascicular fibers and blood vessels. With PM, the inflammatory response is cell-mediated and involves the muscle fascicles themselves. IBM is characterized by perivascular inflammation, inflammation of the perimysium, the sheath of connective tissue surrounding groups of skeletal muscle fibers, and the presence of characteristic vacuoles. Tau protein and beta-amyloid precursor protein may contribute to muscle injury.
DM and PM involve proximal muscle groups, whereas IBM may involve both proximal and distal muscle groups. DM can be seen in children and adults, whereas PM and IBM are generally seen only in adults with IBM involving those older than age 50 years with a male predominance. DM may be associated with a rash or underlying carcinoma.
Inflammatory myopathies generally cause symmetrical proximal muscle weakness with insidious onset of symptoms due to slowly progressive muscle weakness. Inflammatory myopathies can also involve the diaphragm, intercostal muscles, and accessory muscles but other peripheral muscle groups are usually more severely affected than the respiratory muscles. IBM is similar to PM except onset of symptoms is usually after age 50 years; it is more commonly seen in men than in women. Symptoms related to respiratory muscle weakness usually are not the presenting complaints. However, respiratory muscle weakness can develop in 5% to 10% of the patients with DM and PM and may be found in as many as 75% of individuals when respiratory muscle function is carefully evaluated.
Interstitial lung disease may develop in up to 70% of patients with DM or PM. Interstitial lung disease may be especially aggressive with the presence of antisynthetase antibodies. Individuals with DM or PM should be evaluated for the presence of restrictive pulmonary disease, which may be due to respiratory muscle weakness as well as underlying interstitial lung disease. If respiratory muscle weakness is pronounced (MIP < 30% of predicted), ventilatory failure may ensue. Respiratory muscle weakness can also impair cough, which, in concert with esophageal dysfunction and lymphopenia, can predispose these patients to pneumonia.
Diagnostic workup includes measuring muscle enzymes and autoantibodies, magnetic resonance imaging, and muscle biopsy. Muscle enzymes (creatine kinase, aldolase, and lactate dehydrogenase) can be elevated in all inflammatory myopathies but more so in DM and PM than in IBM. Serologic markers that are specific for DM and PM include autoantibodies such as anti-Jo-1; antibodies to signal recognition particle (anti-SRP antibodies); and antibodies to Mi-2, a nuclear helicase. Type 1 interferon-inducible transcripts can be detected in blood in DM and PM and may play a pathogenic role. Magnetic resonance imaging provides a broad sampling of muscle and is helpful in distinguishing between PM and IBM. With IBM, abnormal changes can be seen throughout the muscle; whereas changes are only noted along fascial planes in PM. The presence of typical inclusion bodies on muscle biopsy is diagnostic of IBM.
Metabolic Myopathies
Glycogen Storage Disease.
A number of enzyme deficiencies can lead to disordered glycogen metabolism, resulting in accumulation of glycogen in tissue including skeletal and cardiac muscle. The glycogen storage diseases that are most likely to affect the respiratory system are acid maltase deficiency (type II—Pompe disease) and debranching enzyme deficiency (type III—Forbes-Cori disease). These disorders usually present in childhood, but some, such as Pompe disease, have separate adult-onset forms. McArdle disease (type V) results from myophosphorylase deficiency and can lead to exercise intolerance. In general, glycogen storage disorders are inherited as autosomal recessive conditions.
Acid Maltase Deficiency.
Acid maltase deficiency, also known as glycogen storage disease type II or Pompe disease, is due to the deficiency of acid α-glucosidase, an enzyme responsible for the degradation of glycogen polymers to glucose. Deficiency of this enzyme allows accumulation of glycogen within cardiac and skeletal muscle lysosomes, resulting in myopathy. Although typically involving infants, this disease can manifest in adulthood. Complete enzyme deficiency results in cardiorespiratory failure and death, usually in the first year of life. When the onset of weakness begins after age 1 year, the disease is less severe and does not involve the heart. Initially, subjects develop symptoms related to muscle weakness. Those with a later onset of symptoms have a better prognosis. With diaphragm involvement, there may be restrictive ventilatory limitation, intolerance of the supine position, and in most severe cases, respiratory failure. Results from trials of enzyme replacement therapy with recombinant acid α-glucosidase are promising, showing stabilization of pulmonary function and increased survival.
Other Metabolic Myopathies.
Myopathies can be caused by defects of lipid metabolism (carnitine palmitoyl transferase deficiency) or disorders involving the mitochondria directly. Disorders of lipid metabolism may be caused by an abnormal carnitine transporter protein (“primary” carnitine deficiency) or a deficiency of carnitine secondary to other metabolic diseases. These disorders usually do not cause respiratory disability but involve other skeletal muscle groups (arms and legs) and may involve cardiac muscle.
Mitochondrial disorders include those due to deficiencies of enzymes in the mitochondrial respiratory chain complexes and to defects of phosphorylation-respiration coupling. Disorders of mitochondrial metabolism involving complexes I, II, III, IV, and V cause multiple respiratory chain defects and alter the kinetics of oxygen metabolism during exercise. The main consequences of mitochondrial myopathy are muscle weakness, muscle wasting, and exercise intolerance. Dyspnea that accompanies exercise may be due to an exaggerated ventilatory response to metabolic demands. Individuals may exhibit external ophthalmoplegia, ptosis, and hypertrophic or dilated cardiomyopathy. Occasionally, mitochondrial myopathies cause significant respiratory muscle weakness resulting in nocturnal hypoventilation and the need for assisted ventilation. Treatments that focus on bypassing the defective mitochondria include dietary supplements based on dietary substances involved in adenosine triphosphate production such as creatine.
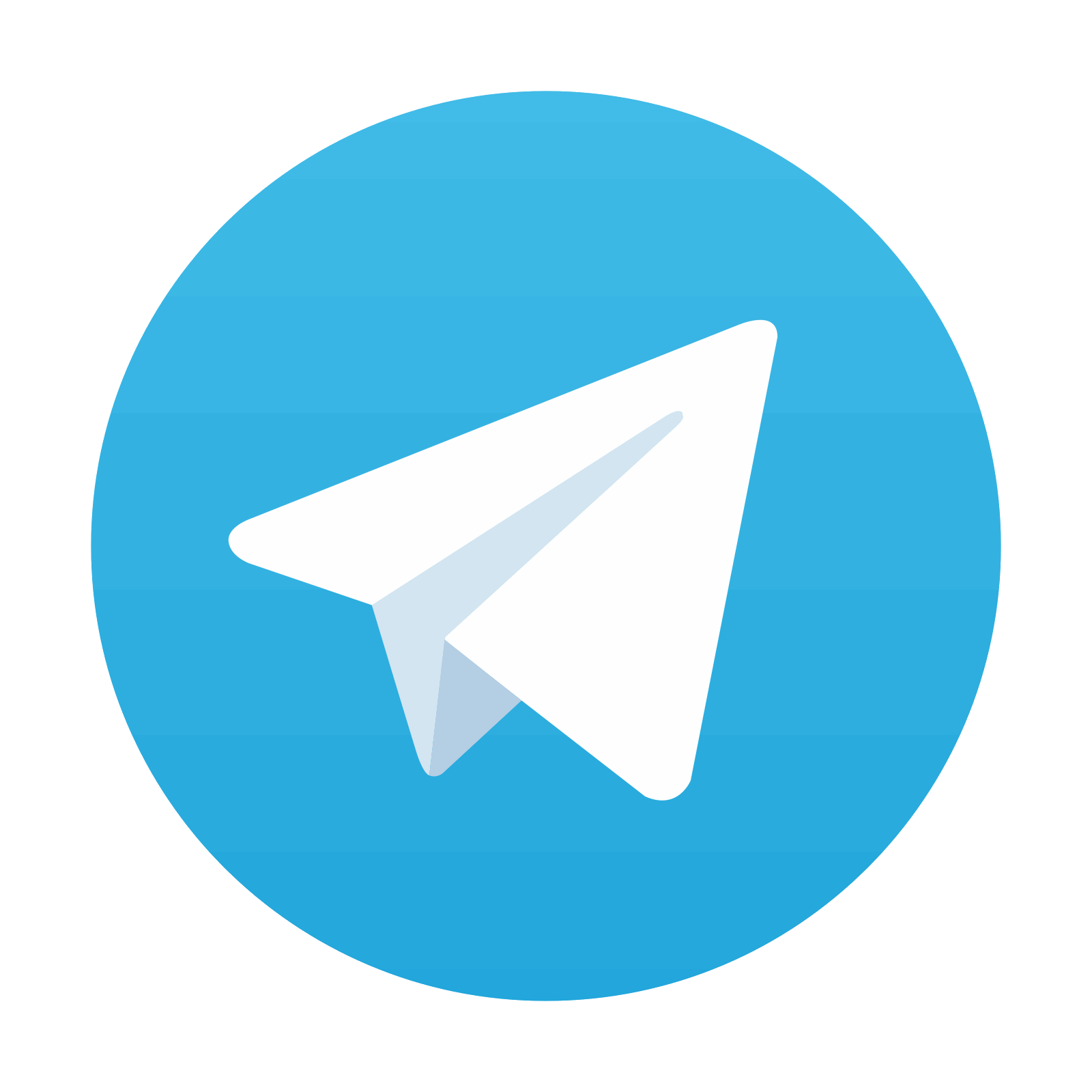
Stay updated, free articles. Join our Telegram channel

Full access? Get Clinical Tree
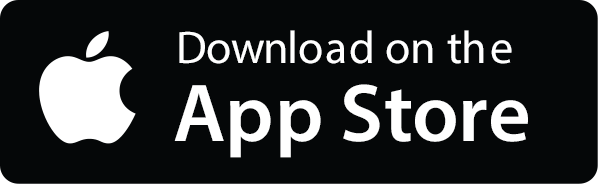
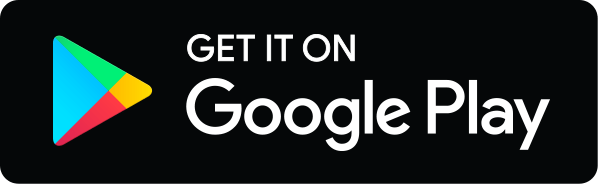