Chapter contents
2.1 The circulatory system 5
2.2 The pulse 6
2.3 Blood 10
2.4 Summary 13
2.5 The pulse in biomedicine 13
2.6 The pulse in contemporary CM clinical practice 17
This chapter introduces the concepts of blood flow and pressure waves, and looks at the radial artery and its essential features, and its relationship to pulse diagnosis. The chapter is intended as a brief summary of a complex and diverse body of literature that is available on the topic, and is presented here to support further discussions on CM pulse diagnosis presented later in this book. It should not be considered definitive but may be used as a basis for further investigations into understanding the physiological basis of the pulse from other literature sources.
2.1. The circulatory system
The circulatory system functions as a transportation system. It distributes the nutrients and substances required for healthy metabolism to tissue cells and organs while removing metabolic waste from those same cells and organs. According to Berne & Levy (1981: p. 1) the components of the circulatory system can in their simplest form be reduced to a pump moving fluids through a distributing network of tubes. Yet this analogy of the circulatory system is overly simplistic when the circulatory system is considered within the context of health. Rather than the passive distributing network implied in the analogy, it is actually a dynamic regulatory system assisting in the maintaining body temperature; regulate volume flow of blood; and act as a communication network between different body regions. These functions of the circulatory system depend on controlled changes in the width of blood vessels (vasoconstriction and vasodilatation) and the pump-like action of the heart to cause blood flow. These functions are all mediated through an innate biofeedback system which helps maintain homeostasis and organ function. For example, by reducing the blood vessel diameter (vasoconstriction), the surface area of the blood vessel exposed to the environment also decreases and body heat is retained while concurrently reducing blood flow to the superficial and peripheral regions of the body. Increasing blood vessel diameter (vasodilatation) increases the surface area of the blood vessel, thus increasing the rate at which body heat is lost to the environment. The supply of nutrients circulating to the tissue cells is regulated by this same vasodilatation/vasoconstriction mechanism. Chemical transmitters, hormones and other chemical markers produced by the body and required for regulating homeostasis are similarly distributed in this manner.
The body’s organs and tissues have varying nutritional and blood flow requirements for their health and normal functioning. Such requirements also vary depending on the time of day and level of activity. This is reflected in the differing density and type of blood vessels found in different regions of the body. For example, the skin has small requirements for copious blood flow and so the capillary network is fine but densely distributed; useful in times of tissue repair due to trauma or for dispersing body heat during exercise. On the other hand, the brain has a high and constant requirement for blood flow and several large arteries serve this purpose by maintaining a continuous supply of blood flow to the organ, while the lungs are rich in small capillary beds to assist in the transfer of metabolic waste for oxygen. Similarly, other organs vary in both the density of blood vessels present and anatomical structure of the vessels depending on function and purpose. The kidneys in particular have a unique circulation, using specialised arterial structures to increase partial blood pressures to filter toxins from the blood.
These concentrations of blood vessels that serve such a specialised function can be termed a microcirculation. Berne & Levy have noted that some chemicals, whether intrinsic or introduced by therapeutic interventions, may have differing affects on the microcirculation and on arterial smooth muscle:
In studies on this interesting and important type of muscle, great care should be taken in extrapolating results from one tissue to another or from the same tissue under different physiological conditions. For example, some agents elicit vasodilation in some vascular beds and vasoconstriction in others (1981: p. 124).
In the context of health assessment, it is logical to conclude that dysfunction or increased demands on an organ, whether in response to neural, hormonal or chemical stimulus, should mean that the circulatory requirements, and hence the microcirculation of that organ, would be affected.
Extrapolating about Chinese medical ideas then, it is not inconceivable to link such microcirculatory changes posited by Berne and Levy to Chinese medical ideas on pulse diagnosis. In this sense, it can be that such microcirculatory changes when applying CM theory, are detectable elsewhere in the body, and in particular in the radial artery, via signals carried in the haemodynamic pulse wave. Dai et al (1985) hypothesised and demonstrated that disturbances in the arterial blood flow in one region of the body can be detected elsewhere in the body using the arterial pulse wave. They did this through intermittent occlusion of blood flow in the right leg, showing a corresponding reduction in the force level of the pulse in both left and right radial arteries (using pressure transducer measurements). Therefore, it is not illogical to state that changes in an organ’s microcirculation would similarly affect arterial haemodynamics detectable elsewhere in the circulatory system.
At any given point in time, the pulse should reflect the circulatory system’s ability to undertake the distributing and regulatory functions described above. What is described as a ‘pulse’ in the clinical context is foremost, and always, a product of the circulatory system, generated by pressure changes which occur with heart movement. In this context, pulse diagnosis is used to assess heart movement, measuring the rate or frequency of pulse occurrence and whether this is occurring in a rhythmic fashion. From the assessment of pulse movement, it is inferred that the heart is moving and functioning in a particular fashion. Yet these two ‘movement’ characteristics, rate and rhythm, do not adequately explain or describe the range of information that CM literature, or indeed biomedicine, claim as being able to obtain from assessment of the pulse. Thus, in addition to pulse rate and rhythm, there are several other characteristics of the pulse that are assessed. (These are discussed in Chapter 6 and Chapter 7.) In a CM diagnostic framework, each is used to infer the function of a particular aspect of the body via the degree of change occurring in each of the pulse characteristics. The practitioner aims to determine whether the perceived changes in the pulse have arisen due to internal organ function, compromised blood flow, or external conditions such as viruses, bacteria or other environmental factors. It is the body’s response to these factors or attempt to maintain homeostasis or balance which is of interest in the process termed ‘pulse diagnosis’. Investigation and observation of changes in the pulse and their attribution to health is the aim of pulse diagnosis. This leads us to the next question — exactly what is the pulse?
2.2. The pulse
The pulse is a physiological phenomenon propagated throughout the arterial system. It is generally viewed as a travelling pressure wave caused by the rhythmic contraction and relaxation of the heart. Thus the pulse can be described in terms of systole, when the pulse amplitude increases or the heart contracts; and diastole, when the amplitude decreases or the heart relaxes (Box 2.1). A pulse can also be thought of in terms of any regular movement or change in differential pressure that manifests as a rise and fall of fluid in a vessel. In terms of clinical practice, a ‘pulse’ is usually associated with a palpable pressure movement. For example, the radial artery, located at the wrist overlying the radius, is a major site at which to feel the pulse.
Box 2.1
Some definitions
• Systole: The period when the left ventricle of the heart is in contraction forcing blood into the aorta. Systolic pressure is the maximal pressure exerted in the arteries by the heart during systole.
• Diastole: The period when the left ventricle of the heart is in relaxation following systole, when it is refilling with blood. Diastolic pressure is the baseline or resting pressure during diastole, when the heart is at rest. Diastole is the constant pressure that is always present in the artery.
• Blood pressure: The pressure exerted on the walls of the blood vessels. Blood pressure measurements describe the maximal systolic pressure over the resting basal diastolic pressure. Pressure is measured in millimetres of mercury (mmHg). Average blood pressure is 120/80 mmHg.
The pressure wave produced with heart movement is often considered the ‘pulse’, but for purposes of pulse diagnosis using manual palpation the pulse encompasses more than the pressure wave alone. In addition to pressure waves, O’Rourke et al. (1992) note the pulse also encompasses flow waves; the actual movement of blood. They note there is even a third type of wave called diameter waves produced with vessel diameter changes, but these waves are very similar to the pressure wave (O’Rourke et al 1992: p. 17) and, as such, will not be discussed any further in this book. Each of these waves is distinctly different when looking at objective measurements of their contours. Therefore, what is regarded as a single ‘pulse wave’ is in fact a composite of at least two different types of waves. There is the pressure wave, the actual force caused by heart movement, and there is the flow wave, determined by how the pressure wave acts on the actual flow movement of blood. How each contributes to the overall pulse formation probably determines the spectrum of diverse pulse qualities in the CM literature.
In the context of health, it is important to understand that the pulse wave is not a static artefact of heart movement, nor does the pulse remain in constant shape as it moves through the arterial system. It is rather in a constant state of change, acted on by the characteristics of the vessel conduit, the functional state of the organs and tissue and the quality of blood itself, which is in turn determined by the state of health of the individual. It is the cumulative effect of the whole range of these factors that moulds the pulse wave into the pulse that eventually arrives at the radial artery as felt by practitioners. Therefore, the essential characteristics of how the pulse presents to the practitioner when palpating the pulse infer the nature of illness and an individual’s state of health, an intimate ‘record’ of the bodily environment. Pressure waves, flow waves and blood are discussed in further detail below.
2.2.1. Pressure waves
Heart movement produces pressure which causes arterial blood to flow. Pressure waves are generated by the expulsion of blood with heart contraction into the aorta, causing dilatation of that vessel (Guyton and Hall 2006). Thus the greater the blood volume expelled, the greater the pressure wave produced, assuming the aorta’s elastic characteristics remain constant. Blood flows in a closed system of vessels. Any generated pressure therefore affects the entire system. As the pressure wave rises and falls with heart movement, so the blood movement ebbs and flows. In this closed system of pressure and flow, the pressure wave always precedes the actual blood flow, moving considerably faster than its causative affect on the movement of blood (Berne & Levy 1981: p. 105).
The pressure wave has two distinctive components. The first or systolic component has a rise to maximum pressure, followed by a slow decline with a notch or slight increase in pressure from aortic valve closure when the backflow of blood in the aorta overcomes the expulsion force of blood from the heart (Fig. 2.1). According to O’Rourke et al. (1992: p. 40) the second component of pulse formation is due to the phenomenon of wave reflection. Reflective waves are an echo of the initial primary wave that travels from the heart to the periphery. As the arteries narrow in the periphery this results in increased arterial resistance, which causes the pulse wave to rebound, causing a reflective wave to move back towards the heart. Reflective waves occur from the lower and upper body regions. Because the blood vessels of the lower body are larger, the reflective wave affect is also larger than the reflective wave resulting from arterial narrowing in the upper limb. However, both the lower and upper reflective waves have systemic effects and are not confined to the limb in which they were generated. In this way, reflective waves from the lower body interact with the pressure waves in the upper part of the body.
![]() |
Figure 2.1Features of the radial arterial pulse wave. |
Reflective waves are visualised as a secondary pressure peak in the pulse pressure wave, usually occurring in the diastolic component of the pulse period. Depending on the degree of arterial stiffness, reflective waves can augment the initial primary pressure wave, moving from diastole into the systole component due to arterial hardening (Fig. 2.2). When this occurs the reflective wave merges with the primary pressure wave moving away from the heart, resulting in a greater and longer
duration of pressure during systole than would normally be seen. This often occurs in individuals with illness and conditions in which the arteries become stiff or inflexible. This causes a maximal systolic blood pressure rise above the usual pressure range regarded as healthy for that individual’s age. (An increase in arterial tension also increases the overall flow rate of blood through the arteries).
Box 2.2. Sphygmography
Diagrammatic representations of pressure waves called sphygmograms or sphygmographs are easy to obtain using pressure sensors. Many CM texts on pulse diagnosis use representation of these graphs to attempt to illustrate different pulse types. Although a useful educative tool, they are limited in their use to represent changes in the pulse wave that occur with different illnesses and caution should be used when looking at these, or mistaking them for the ‘pulse’. This is because many of the CM pulse qualities are not solely dependent for their formation on pressure variations or pressure waves, which are the sole purpose of this form of measurement.
![]() |
Figure 2.2The radial arterial pulse wave and augmentation by reflective waves.(After Fig. 3.3.1 in AtCor Medical 2006, A clinical guide: pulse wave analysis, with permission of AtCor Medical Pty.) |
Figure 2.1 illustrates the pressure wave contour typically measured at the radial artery. This is considerably different from the same pressure wave if measured in another part of the circulatory system such as the carotid artery (Fig. 2.3). This is because the anatomy of the arteries varies throughout the circulatory system and this in turn impacts on how the pulse moves through the artery. For example, some arteries are more elastic than others, so their rate of expansion is greater under pressure. This difference is noticeable with palpation when comparing the pulse in the carotid artery in the neck with the pulse in the radial artery in the wrist. The carotid artery has a broader expansive movement because it is more elastic than the radial artery, which has a more distinct arterial wall because it has a large proportion of smooth muscle and thus is not as expansive. The arteries’ capacity to expand with pressure is termed capacitance. The capacitance of a blood vessel is not constant.
![]() |
Figure 2.3Features of the carotid arterial pulse wave. Note the rounded contour of the peak pulse pressure due to the carotid elastic properties and relative large diameter. |
Capacitance varies under different conditions, such as differing levels of physical or psychological stress causing a corresponding increase in vessel hardening or vasoconstriction. In this situation capacitance decreases. In addition to capacitance, the pressure wave is also dependent on the stroke volume or amount of blood ejected from the heart with heart contraction. An increased volume of blood gives an increased expansion of the arterial wall, and decreasing blood volume has the reverse effect (assuming the arterial width remains constant).
The major blood vessels are elastic and relatively wide contributing to smooth and unimpeded flow of blood (Stettler et al. 1986). This means the pressure wave remains similar to that at the aorta and throughout all major arteries until the periphery. At the periphery, the arteries begin to narrow. A decreasing arterial width means the force of the pressure and blood flow are compacted together and the pressure exerted from within the artery increases. This is evident in blood pressure measurements between peripheral vessels such as the brachial and radial arteries and the aorta. Maximal pressure in the peripheral arteries such as the radial artery can be 30% greater than maximal pressures in the aorta. However, the force exerted by the pulse pressure wave on the blood vessels falls substantially on entering the smaller capillaries and other vessels beyond the radial and other arteries. Here, Guyton and Hall (2000) note the combined diameter of all these small vessels is greater than that of the arteries and so the force exerted by the pressure wave decreases because of the relative increase in surface area, and the force of the pressure wave is diluted. It should be noted that it is the pressure differential between the aorta and these small capillary networks which causes blood flow; blood flows from an area of high pressure into an area of low pressure (Guyton & Hall 2000).
In CM it is often said that the ‘Qi moves the Blood, and the Blood follows the Qi’. That is, function acts on the blood and the blood responds. In this sense, the pressure wave causes the blood to flow and the blood flow responds accordingly, producing a tidal flow through the vessels. This tidal flow is termed a flow wave and is quite distinctly separate from the pressure wave.
2.2.2. Flow waves
Flow waves refer to the longitudinal movement of blood through the vessel and are the second major component of the pulse wave. Flow waves depend on there being a sufficient volume of blood. It is obvious that if blood volume becomes insufficient then there would be no flow wave. Flow wave formation also depends on the nature of the essential constituents of the fluid. For example, imagine applying a pressure wave to a container of water, and a similar pressure wave to a container of honey. Because of the innate difference in the ‘thickness’ or viscosity of the two fluids, each pressure wave would have a different impact in the creation of the flow wave. The water would transmit the pressure wave into a flow wave easily, with a noticeable surface movement. Because of the thickness of the honey this would not be as noticeable, most likely producing only sluggish movement. In this way, blood can also vary, becoming more viscous or fluid depending on the relative ratio of cells and fluid that make up the blood. However, assuming sufficient blood volume and appropriate viscosity flow waves are determined by two additional factors. These are ventricular contraction and mechanical characteristics of the arterial wall.
Ventricular contraction determines the amount of momentum imparted into the blood forcing it to flow through the vessel. The strength of ventricular contraction also has a relationship to the volume and speed at which blood is expelled from the left ventricle of the heart during systole (Opie 2004). For example, if the heart were to contract strongly, and assuming sufficient time and blood volume for the heart to have refilled during diastole, then the peak systolic pressure would be achieved more rapidly resulting in a sharper incline to the peak if recorded by a sphygmogram. This would cause a greater volume of blood to flow into the aorta at a given point in time. This volume output is referred to as stroke volume (SV) (see Box 2.3). Obviously, if blood volume is diminished or heart contraction slowed then the flow wave would be similarly affected.
Box 2.3

Cardiac output
The total volume of blood flow in 1 minute is termed cardiac output (CO). CO depends on the blood volume expelled by the heart during systole of each heartbeat, termed stroke volume (SV), and the total number of heartbeats per minute or heart rate (HR). Thus:

This relationship is commonly used in biomedical practice for determining the heart’s pump function in maintaining adequate blood flow for tissue perfusion. Blood pressure and resistance to blood flow also have a direct bearing on cardiac output.
The second additional factor in flow wave formation involves mechanical properties of the arterial wall. When the heart contracts, a pressure wave moves into the aorta causing it to expand. When the pressure is removed during heart relaxation (diastole) the aorta returns or recoils to its normal shape. The recoil releases this potential energy stored during expansion back into the blood, causing the blood to flow forward. In conditions in which the elasticity of the arteries is compromised, recoil is diminished and the blood flow becomes retarded, which compromises circulation and the associated functions of the circulatory system. For example, if the arteries were relatively stiff, then a secondary fluctuation or reflective wave would move from diastole into systole, augmenting the initial flow wave, whereas a relaxed or elastic artery would not produce augmentation of the initial wave. Conditions affecting the arterial wall affect the flow wave. This relationship between arterial properties and characteristic of ventricular contraction is described as vascular impedance.
2.3. Blood
Blood is an important component of the formation of the pulse. It is a complex fluid, composed of a plasma liquid base and several formed particles, the most prominent of which are red blood cells, which make up 99% of all particle types found in blood. Other components include:
• White blood cells
• Platelets
• Proteins (albumins, globulins, fibrinogen) dissolved in the plasma.
For these reasons blood is referred to as a liquid tissue. Blood is the medium in which the pulse wave propagates from the heart to the periphery. Therefore, changes in the blood medium — that is, changes in any of the ratio of its components — will affect the propagation of the pulse wave. Changes can occur in a number of ways. They can arise due to illness, from trauma producing blood loss, or from diet where an individual’s inadequate intake of appropriate food groups adversely affects the quality and quantity of the blood. In this way, the ratio of the different cell components of blood can vary between people and can vary within the same individual over time. Blood may consequently ‘thicken’ or ‘thin’. The relative degree of ‘thickness’ is termed viscosity.
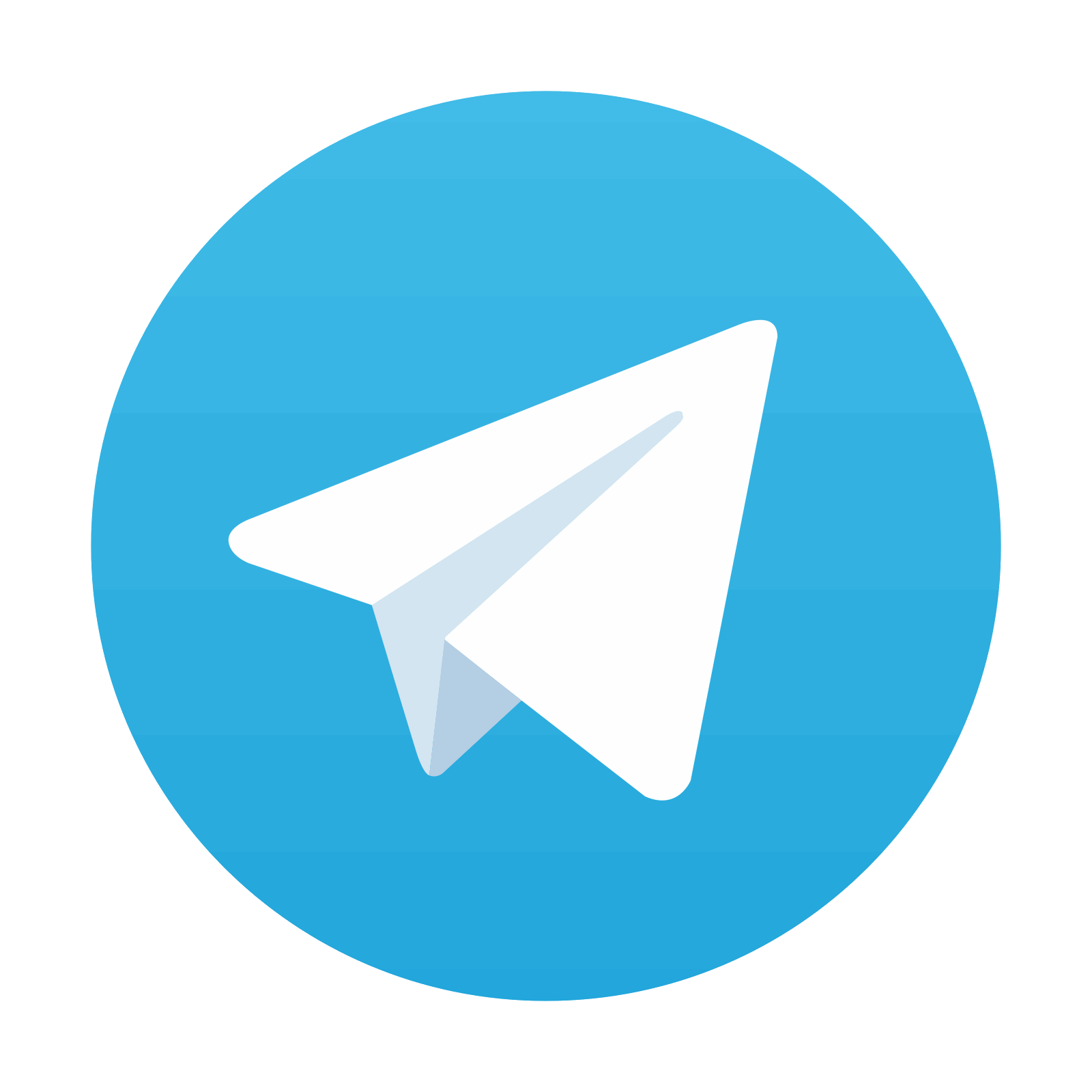
Stay updated, free articles. Join our Telegram channel

Full access? Get Clinical Tree
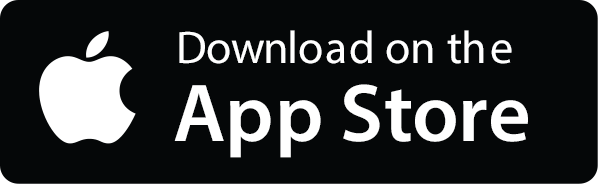
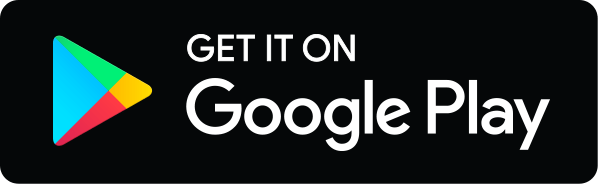