Objectives
- 1.
Describe the anatomy, function, physiology, and regulation of the two circulations in the lung.
- 2.
Describe the distribution of pulmonary blood flow.
- 3.
Explain pulmonary vascular resistance in alveolar and extraalveolar vessels and the effect of lung volume on these resistances.
- 4.
List the anatomic components of the alveolar–capillary network.
- 5.
Describe lymphatic flow and its components.
- 6.
Explain the mechanisms of pulmonary edema formation.
The systemic circulation is composed of the vascular system supplied by the left ventricle that pumps blood into the aorta for distribution to the rest of the body. In contrast, the pulmonary circulation is composed of the vascular system that conducts blood from the right side of the heart through the lungs. These two vascular systems exist in parallel, and both receive the entire cardiac output each minute.
The lung is the only organ in the body that receives blood from two separate sources. The pulmonary circulation brings deoxygenated blood from the right ventricle to the gas-exchanging units. At the gas-exchanging units, oxygen is transported across the alveolar and capillary endothelium into the red blood cell, and carbon dioxide is transferred from the blood into the alveolus before it is returned to the left atrium for distribution to the rest of the body. The second blood supply is the bronchial circulation, which arises from the aorta and provides nourishment to the lung parenchyma. The blood supply to the lung is unique in its dual circulation and its ability to accommodate large volumes of blood at low pressure.
Pulmonary Blood Flow
Pulmonary blood flow consists of the entire output of the right ventricle. All of the mixed venous blood from all of the tissues in the body is pumped out of the right ventricle into the pulmonary circulation. As a result, pulmonary blood flow is equal to cardiac output—about 3.5 L/min/m 2 of body surface area at rest and as much as 25 L/min/m 2 during exercise. At rest, the volume of blood contained in the lung (from the main pulmonary artery to the left atrium) is approximately 500 mL, or 10% of the circulating blood volume. Of this 500 mL, 70 mL of blood is contained in the pulmonary capillary bed. Approximately 280 billion capillaries supply the approximately 300 million alveoli, resulting in a surface area for gas exchange of 70 to 80 m 2 .
The pulmonary capillary bed has the largest surface area of any vascular bed in the body. Many of these pulmonary capillaries are closed at rest and open periodically during periods of increased pulmonary blood flow. The network of capillaries is so dense that it may be considered to be a sheet of blood interrupted by small, vertical, connective-tissue supporting posts (see Fig. 1.8 ). At rest, it takes a red cell about 4 to 5 seconds to travel through the pulmonary circulation, with 0.75 second of this time spent in the pulmonary capillaries. The pulmonary capillaries have diameters of about 6 μm, slightly smaller than the diameter of a red blood cell (8 μm). Thus red blood cells must change their shape as they pass through the pulmonary capillaries. This shape change ensures the smallest possible distance between oxygen in the alveolus and the hemoglobin in the red cell.
During exercise, cardiac output and thus pulmonary blood flow can increase to as much as 25 L/min/m 2 and the pulmonary capillary volume increases and approaches about 200 mL. The increase in blood volume in the lung that occurs as a result of the increase in cardiac output during exercise occurs through two different processes ( Fig. 6.1 ). First, closed or compressed capillary segments are recruited and open as the increase in cardiac output raises the pulmonary vascular pressure. Second, previously open capillaries enlarge as their internal pressure rises. Enlargement of open capillaries also occurs in individuals with left-sided heart failure, which is associated with an elevated left atrial pressure and an increase in pulmonary capillary volume.

The pulmonary veins are situated within loose interlobular connective tissue septae and receive blood from many lung units. They return blood to the left atrium through conventional and supernumerary branches. Because of their large numbers and thin walls, the pulmonary veins provide a large reservoir for blood, and these veins can either increase or decrease their capacitance to provide a constant left ventricular output in the face of a variable pulmonary arterial flow.
Pulmonary arteries and veins with diameters larger than 50 μm contain smooth muscle and are innervated through the sympathetic branch of the autonomic nervous system. Their extensive sensory innervation, located in the outer connective tissue layer (adventitia) of the vessel wall, can be stimulated by changes in vascular pressure (stretch) and various chemical substances. These vessels actively regulate their diameter and thus alter resistance to blood flow. In contrast, pulmonary arterioles and capillaries are devoid of smooth muscle.
Bronchial Blood Flow
The existence of a second, separate circulatory system in the lung with oxygenated blood from the systemic circulation was first observed by Frederich Ruysch in the latter half of the 17th century. This second circulatory system is the bronchial circulation , and it provides systemic arterial perfusion to the trachea, upper airways, surface secretory cells, glands, nerves, visceral pleural surface, lymph nodes, pulmonary arteries, and pulmonary veins ( Fig. 6.2 ). The bronchial circulation perfuses the respiratory tract to the level of the terminal bronchioles. Lung structures distal to the terminal bronchioles, including the respiratory bronchioles, alveolar ducts, alveolar sacs, and alveoli, are directly oxygenated by diffusion from the alveolar air and receive their nutrients from the mixed venous blood in the pulmonary circulation.

Bronchial blood flow comprises only 1% to 3% of the output of the left ventricle. The bronchial arteries, usually three in number, arise either from the aorta or from the intercostal arteries and accompany the bronchial tree and divide with it (see Fig. 1.9 ). The pressure in the bronchial arteries is equal to the systemic pressure and is therefore much higher than the pressure in the pulmonary circulation.
The return of venous blood from the capillaries of the bronchial circulation to the heart occurs either through true bronchial veins or through bronchopulmonary veins. True bronchial veins are present in the area of the hilum and flow into the azygos, hemiazygos, or intercostal veins before entering the right atrium. The bronchopulmonary veins are formed through a network of tributaries composed of both bronchial and pulmonary circulatory vessels, which anastomose and form vessels with an admixture of blood from both circulatory systems (see Fig. 6.2 ). Blood from these anastomosed vessels returns to the left atrium through pulmonary veins. It is estimated that about two-thirds of the total bronchial circulation is returned to the heart via the pulmonary veins and this anastomosis route. This deoxygenated blood, which mixes with oxygen-enriched blood in the pulmonary veins, contributes to the small alveolar–arterial oxygen difference in normal individuals (see Chapter 7 ).
The physiologic function of the bronchial circulation remains somewhat of an enigma; lung transplant studies have shown that adult lungs can function normally in the absence of a bronchial circulatory system. Thus in healthy individuals, these anastomoses are probably of little importance. They may, however, be important in neonates and young children in bringing nutrients to the developing lung and in disease states in which reciprocal changes in flow in the two circulations provide a steady supply of nutrients. For example, in individuals with a pulmonary embolus , the bronchial circulation increases to the areas of the lung normally supplied by the pulmonary arteries; as a result, pulmonary infarction (death of the lung tissue) rarely occurs even with large pulmonary emboli. Similarly, in individuals who have undergone lung transplantation in which the bronchial circulation has been removed, necrosis (death) of the airway cells and other structures supplied by the bronchial circulation does not occur because of an increase in pulmonary circulation to these areas.
Pressures and Flows in the Pulmonary Circulation
The pulmonary circulation begins in the right atrium. Deoxygenated blood from the right atrium enters the right ventricle via the tricuspid valve and is then pumped under low pressure (9–24 mm Hg) into the pulmonary artery through the pulmonic valve ( Fig. 6.3 ). The main pulmonary artery, also known as the pulmonary trunk, is about 3 cm in diameter; it branches quickly (∼5 cm from the right ventricle) into the right and left main pulmonary arteries, which supply blood to the right and left lungs, respectively. The arteries of the pulmonary circulation are the only arteries in the body that carry deoxygenated blood. The deoxygenated blood passes through a progressively smaller series of branching vessels (vessel diameters: arteries >500 μm, arterioles 10–200 μm, capillaries <10 μm) ending in a complex, mesh-like network of capillaries with very thin walls and large internal diameters relative to their wall thickness. The sequential branching pattern of the pulmonary arteries follows a pattern similar to airway branching, such that there are supporting vascular structures for each airway (see Fig. 1.9 ). The pulmonary circulatory system functions to (1) reoxygenate the blood and remove CO 2 , (2) aid in maintenance of fluid balance in the lung, and (3) distribute the metabolic products of the lung.

Oxygenation of red blood cells occurs in the alveolus where the pulmonary capillary bed and the alveoli come together in the alveolar wall in a configuration that achieves optimal gas exchange. Gas exchange occurs through this alveolar–capillary network.
Oxygenated blood leaves the alveolus through a network of small pulmonary venules (15–500 μm in diameter) and veins, which quickly coalesce to form larger pulmonary veins (>500 μm in diameter) in which the oxygenated blood returns to the left atrium of the heart. In contrast to arteries, arterioles, and capillaries, which closely follow the branching patterns of the airways, venules and veins run quite distant from the airways.
Unlike systemic arteries, the arteries of the pulmonary circulation are thin-walled with minimal smooth muscle. This has important physiologic consequences, including less resistance to blood flow. Pulmonary arteries are also more distensible and compressible than systemic arterial vessels, and they are seven times more compliant. This highly compliant state requires much less work (lower pressures throughout the pulmonary circulation) for blood flow through the pulmonary circulation compared with the more muscular, noncompliant arterial walls of the systemic circulation. Furthermore, the vessels in the pulmonary circulation, under normal circumstances, are in a dilated state and have larger diameters compared with similar arteries in the systemic circulation. All of these factors contribute to a very compliant, low-resistance circulatory system, which aids in the flow of blood through the pulmonary circulation via the relatively “weak” pumping action of the right ventricle, which is less muscular than the left ventricle. The pressure gradient differential for the pulmonary circulation from the pulmonary artery to the left atrium is only 6 mm Hg (14 mm Hg in the pulmonary artery minus 8 mm Hg in the left atrium) (see Fig. 6.3 ). It is almost 15 times less than the pressure gradient differential of 87 mm Hg present in the systemic circulation (90 mm Hg in the aorta minus 3 mm Hg in the right atrium).
Pulmonary Vascular Resistance
Four factors influence blood flow in the lung: pulmonary vascular resistance (PVR), gravity, alveolar pressure, and the arterial to venous pressure gradient. Blood flows through the pulmonary circulation in a pulsatile manner following the pressure gradient in this low resistance system. PVR cannot be measured directly but is most often calculated using Poiseuille’s law, in which R equals the difference in pressure between the beginning of the tube (P 1 ) and the end of the tube (P 2 ) divided by the flow ( <SPAN role=presentation tabIndex=0 id=MathJax-Element-1-Frame class=MathJax style="POSITION: relative" data-mathml='Q˙’>Q˙Q˙
Q ˙
); that is,
R = P 1 − P 2 Q ·
In the pulmonary circulation, PVR is the change in pressure from the pulmonary artery (P pa ) to the left atrium (P la ) divided by the flow ( <SPAN role=presentation tabIndex=0 id=MathJax-Element-3-Frame class=MathJax style="POSITION: relative" data-mathml='Q˙’>Q˙Q˙
Q ˙
t ), which is the cardiac output; that is,
PVR = P PA − P LA Q . T
Under normal circumstances, with normal cardiac output,
PVR = 14 mm Hg − 8 mm Hg 6 L / min = 1.0 mm Hg / L / min
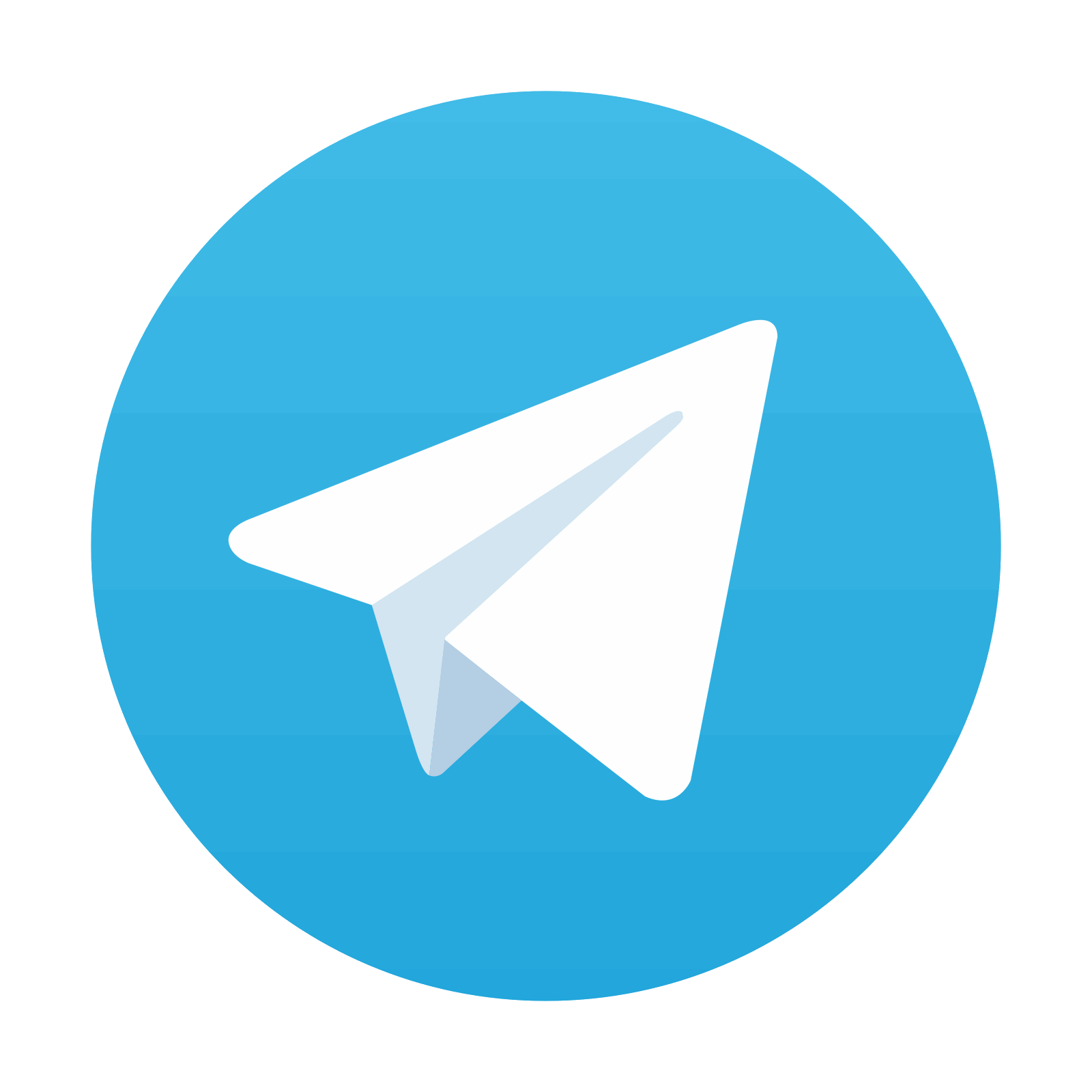
Stay updated, free articles. Join our Telegram channel

Full access? Get Clinical Tree
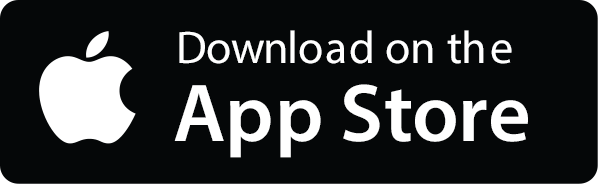
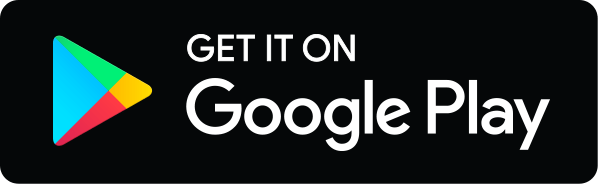
