1
The Normal Lung
Functionally, the lung consists of the acini, which are units specialized for exchange of gas between air and blood, and the conducting tissues, airways, and blood vessels that distribute the air and blood to the gas-exchanging units. Disease processes may involve acini, airways, or vessels selectively or in combination. Differing as they do in structure, function, and cellular composition, these tissues react differently in disease. Thus an understanding of lung disease requires knowledge of the structure and function of the tissues that make up the normal lung.1
Gross Anatomy
General Features
The lungs are paired, asymmetrical organs roughly conical in shape. Their normal combined weight averages 850 g in men and 750 g in women.2 The space that they occupy in the thoracic cavity is enclosed by the rib cage dorsally, laterally, and ventrally; the mediastinum medially; and the diaphragm inferiorly. The shape of the expanded lung, after removal from the body, corresponds with the cavities thus defined. The lung has three surfaces: a convex surface abutting the rib cage, more sharply curved posteriorly than anteriorly; a concave mediastinal surface; and a concave diaphragmatic surface conforming to the convexity of the diaphragm as it covers the domes of the liver and spleen. Toward the posterior portion of the mediastinal surface lies the hilum of the lung, the region in which the bronchi, blood vessels, lymphatics, and nerves enter (Fig. 1–1). Distinct, sharp margins are formed anteriorly by the junction of the mediastinal and costal surfaces and inferiorly by the junction of the costal and diaphragmatic surfaces; the posterior transition from the costal to mediastinal surface and the junction of the mediastinal and diaphragmatic surfaces are rounded. The right lung is slightly larger than the left because of the space required to accommodate the heart on the left side of the mediastinum, but the vertical (cephalocaudal) dimension of the right lung is less than that of the left lung because of the higher position of the right hemidiaphragm where it covers the right lobe of the liver.
The lungs can move freely within the thoracic cavity, being attached only at the two hila. The free surfaces of the lung are covered by a serous membrane, the visceral pleura, which is reflected over the hilum to cover the mediastinum, chest wall, and diaphragm as the parietal pleura. The pleural reflection at the hilum continues inferiorly as the pulmonary ligament. In vivo, the parietal and visceral pleura are normally closely apposed, lubricated by a thin film of pleural fluid, the composition of which is similar to that of other interstitial fluids. The exact volume of pleural fluid in normal subjects is not known, but it is not more than a few milliliters.
The lungs are divided into their major subdivisions, the lobes, by clefts or fissures lined by visceral pleura (Fig. 1–1). The right lung has three lobes. The major fissure follows an oblique course from a level above the hilum dorsally to the base of the lung anteriorly, dividing the inferior lobe from the remainder of the lung; a second (minor) fissure, nearly horizontal, separates the remainder into a superior and middle lobe. The left lung is divided into superior and inferior lobes by a single oblique fissure; it has no middle lobe. The homologous region, the anterior and inferior portion of the superior lobe, is known as the lingula because of its tongue-like extension anteriorly into the costophrenic sulcus. It is frequently partially set off from the remainder of the lobe by an incomplete fissure.
FIGURE 1–1 External appearance of fully inflated adult human lungs showing the normal lobe pattern. (A) Lateral surface, right lung. (B) Medial surface, right lung. (C) Lateral surface, left lung. (D) Medial surface, left lung. The hilar structures seen on the medial surfaces are the bronchi (labeled B), pulmonary arteries (labeled A), and pulmonary veins (labeled V). The pleural reflection at the hilus extends inferiorly as the pulmonary ligament (labeled L). Note that the right pulmonary artery enters the lung anterior to the bronchus, whereas the left pulmonary artery crosses over the bronchus.
Mixed venous blood is brought to the lung by the pulmonary artery, which leaves the right ventricle of the heart anterior and to the left of the ascending aorta and branches below the aortic arch. The right main pulmonary artery passes beneath the arch of the aorta to enter the lung anterior to the right main bronchus, with which it is closely associated. The left pulmonary artery passes above the main stem bronchus and lies above it in the hilus, where it passes over the superior lobar bronchus, coming to lie posterior to the bronchus. Within the lung, the pulmonary arteries accompany the bronchi, branching with them until they reach the acini. The pulmonary veins drain independently from the bronchi, with two trunks leaving each lung at the hilus to enter the left atrium separately.
The nutrient blood supply to the bronchi, associated lymph nodes, major pulmonary vessels, and part of the pleura comes from the systemic circulation via bronchial arteries, which vary in number and origin.3 They arise either directly from the aorta or from the intercostal, internal mammary, or subclavian arteries passing along the esophagus and posterior wall of the main bronchi to enter the lung. Within the lung, they remain within the connective tissue sheath of the bronchi, where they extend peripherally to the level of the bronchioles. Bronchial vessels are not found within the acini. Apparently the venous blood provided by the pulmonary artery supplies all the metabolic substrates required by the lung parenchyma, and oxygen is supplied by diffusion from the airspaces. Venous drainage from the walls of the more peripheral bronchi enters the pulmonary veins. Bronchial veins drain only the central bronchi and nearby pleura and empty into the azygos and hemiazygos veins.
Subunits of Lung Structure
In addition to the lobes of the lung, various subunits of lung structure are recognized. The bronchopulmonary segments are important to thoracic surgery because they are subunits of a lobe that can be resected relatively conveniently with little hemorrhage or air leakage from the raw surfaces. Generally, they are the unit of lung supplied by the first generation of bronchi below the lobar bronchi, are roughly pyramidal in shape, and are pleural based. The nomenclature of the segments is given in Table 1–1, and their pleural projections outlined in Fig. 1–2. A detailed discussion of the many variations in segmental anatomy can be found in Boyden’s monograph.4
When the pleural surface of the lung is viewed, connective tissue septa outline polygonal units ~1 cm in diameter. On the cut surface of the sliced lung, these septa extend a variable distance into the lung, in completely enclosing units known as pulmonary lobules or secondary lobules of Miller5 (Fig. 1–3).
The acinus is the basic unit of gas exchange. The unit is supplied by a single terminal bronchiole; it consists of respiratory bronchioles, alveolar ducts, alveolar sacs, and alveoli. Individual acini cannot be recognized grossly. A lobule consists of three to five acini; although it is a less meaningful structure than an acinus in terms of lung function, the lobule provides a convenient reference for gross pathology. The terminal bronchioles branch near the center of the lobules, and their acini terminate in alveolar sacs abutting the connective tissue septa. Consequently, lesions affecting the proximal portions of acini predominate near the center of the lobules, and the lesions of the distal portions of the acini adjoin the lobular septa.
Right Lung | Left Lung |
---|---|
Upper lobe 1. Apical 2. Posterior 3. Anterior | Upper lobe 1, 2. Apical-posterior 3. Anterior lingula |
Middle lobe 4. Lateral 5. Medial | Middle lobe 4. Superior 5. Inferior |
Lower lobe 6. Superior 7. Medial basal (cardiac) 8. Anterior basal 9. Lateral basal 10. Posterior basal | Lower lobe 6. Superior 7. Anteromedial basal 8. Lateral basal 9. Posterior basal |
FIGURE 1–2 Pleural projections of the bronchopulmonary segments. Numbering is explained in Table 1–1. There is no segment number 7 in the left lung.
FIGURE 1–3 Sectioned surface of a lung showing lobules partially enclosed by connective tissue septa. The extent of development of the septa varies from lung to lung and from one region to another in the same lung.
Airways
Classification
Although the conducting airways share a common plan in that all are muscular tubes lined by a ciliated epithelium, they differ in detail depending on size.6,7 The airways ~2 mm or more in diameter have walls reinforced by cartilage and are called bronchi. Conducting airways without cartilage are called bronchioles. In many species, rodents, for example, there is an abrupt change from the bronchioles to gas-exchanging tissue formed of alveoli. In humans and other primates, transitional airways called respiratory bronchioles have a bronchiolar structure over a portion of their circumference but also have alveoli over a portion. The term terminal bronchiole is used to identify the most distal generation of bronchioles completely free of alveoli, that is, the parent generation to the respiratory bronchioles.
Bronchi
The bronchi are lined by a pseudostratified ciliated columnar epithelium that rests on a homogeneous eosinophilic membrane 1 to 3 μm thick, often regarded as the basement membrane by light microscopists (Fig. 1–4). As seen by electron microscopy, the bronchial epithelium rests on a typical slender basal lamina, but a layer of closely packed collagen fibers lies just beneath it; it is the combined structure that is recognized by light microscopists as basement membrane. Beneath the basement membrane is the lamina propria or subepithelial compartment (often mistakenly termed submucosa), which contains loose connective tissue with bundles of longitudinally arranged elastic fibers.
FIGURE 1–4 Mucosa of a major bronchus. Basal cells, goblet cells, and ciliated cells rest on a thin basement membrane (×400).
The Bronchial Wall
In the trachea and main bronchi, cartilage and smooth muscle are segregated in different parts of the airway wall, whereas in intrapulmonary airways they occur together. The anterior walls of the trachea and main bronchi are formed with V-shaped rings of hyaline cartilage, which open dorsally. The flat dorsal wall is composed of transversely disposed smooth muscle (the trachealis muscle). In the intrapulmonary bronchi, smooth muscle bundles lie deep to the zone of subepithelial elastic fibers and wind down the airway wall in a spiral with a shallow pitch. Consequently, the predominant effect of contraction of the smooth muscle is airway narrowing. Loose connective tissue and bronchial glands occupy the space between the muscle and the outermost layer of the bronchi, which consists of heavy circumferential bundles of collagen fibers and hyaline cartilage. In the intrapulmonary bronchi, the cartilage takes the form of variably shaped islands that diminish in size and number progressively with the decreasing caliber of the bronchi (Fig. 1–5). Their distribution was investigated in detail by Hayward and Reid,6 who found that, in the axial bronchial pathways, there was a high density of cartilage that effectively provided circumferential support for the first four to six generations. The axial bronchi had only scattered plates for another four to six generations. Laterally branching bronchi had circumferential cartilaginous support only at their orifices and occasional cartilage plates for another five to six generations.
FIGURE 1–5 Large intrapulmonary bronchus in a child’s lung. Multiple plates of cartilage completely enclose the bronchus, yet potentially permit it to vary in caliber. The pulmonary artery at the lower left occupies the same connective tissue sheath as the bronchus (×150).
Horsefield7 pointed out that the pattern of cartilage organization is well adapted for an efficient cough mechanism. During coughing, forced expiration against a closed glottis generates marked positive intrathoracic pressure; simultaneously, the airways markedly narrow. When the glottis abruptly opens, the high pressure produces very rapid flows through the narrowed tubes to dislodge obstructing material. The extrapulmonary airways have no external support, and the cartilage rings enable them to remain open even when compressed by positive intrathoracic pressure. The intrapulmonary bronchi are resistant to collapse, being tethered by the surrounding lung tissue, and the incomplete cartilaginous plates provide additional buttressing while still permitting the bronchi to narrow.
The intrapulmonary bronchi are enclosed in a sheath of loose connective tissue containing bundles of collagen fibers and adipose tissue, together with the bronchial arteries, venous trunks, lymphatics, and nerves.
The Bronchial Mucosa
The epithelium lining the bronchi functions mainly in the production and propulsion of mucus.8 If dust or organisms in the inspired air should impact on the bronchial wall, they deposit on a thin coating of mucus, produced by specialized secretory epithelial cells in the bronchial surface epithelium and glands located in the connective tissue of the bronchial wall.9 The mucus streams in a generally cephalad direction to the oropharynx, propelled by the beating of cilia in the respiratory mucosa. The rate of movement of the mucus varies with the level of the bronchial tree, being slower in peripheral bronchi and fastest in the central bronchi and trachea, where particles are transported at a rate of 1 to 2 cm/min. The mucous blanket is considered to have two layers: a superficial gel layer rich in macromolecules, providing a viscoelastic barrier, and a watery serous layer beneath it in which the cilia beat. The mucous gel is composed of large polydispersed glycoproteins with molecular weights of several million daltons. They are composed of 75 to 80% O-linked carbohydrate side chains attached to a protein core. The mucin proteins are products of several genes on at least three different chromosomes. Mucin genes expressed in the respiratory tract are also expressed in other mucus-producing epithelia.10,11 In addition to mucous glycoproteins, the bronchial secretions contain antibacterial proteins such as lysozyme and lactoferrin,12 immunoglobulins (Ig) (principally, IgA),12 and locally produced proteinase inhibitors.13
In standard histologic sections, the bronchial surface epithelium consists of three principal cell types: basal, ciliated, and secretory cells (Fig. 1–4). Round nuclei just above the basement membrane belong to the basal cells, small triangular cells that rest on the basal lamina and are excluded from the bronchial lumen by the neighboring columnar cells. They have a high nuclear-to-cytoplasmic ratio, tonofilament bundles, and numerous desmosomes and hemidesmosomes through which they anchor neighboring columnar cells to the basal lamina.14 Although it is controversial whether basal cells are stem cells in the turnover of airway epithelium under normal conditions, they have the capacity to divide and differentiate into columnar cells12 and doubtlessly serve as stem cells following injury to the columnar cells. Above the nuclei of the basal cells are elongated nuclei of columnar cells, ciliated and secretory. Ciliated cells are normally approximately three to five times more numerous than secretory cells.
Ciliated Cells
The ciliated cells are columnar cells attached to the basal lamina and reaching the bronchial lumen. They have a centrally placed oval nucleus, a large Golgi apparatus, and usually several large lysosomal residual bodies lying near the Golgi apparatus just above the nucleus. Mitochondria, which are found in all parts of the cell, are particularly concentrated in a zone near the apex of the cell close to the cilia, which they supply with adenosine triphosphate (ATP). Ciliated cells do not take up tritiated thymidine, and their replacement takes place by proliferation and differentiation of mucus-secreting and basal cells.13
The structure and cell biology of cilia have been reviewed by Satir.15 The cilia are membrane-covered extensions of the apex of the cell, 5 to 8 μm in length and 0.3 μm in diameter. With a few exceptions, the basic structure of cilia is similar in most eukaryotic organisms. The basic machinery of the cilium resides in the axoneme, a complicated structure that arises as an outgrowth of basal bodies, centriole-like structures anchored in the apical cytoplasm. The axoneme consists of nine peripheral microtubule pairs arranged in a circle around two central single microtubules (Fig. 1–6). Each of the peripheral doublet fibers consists of a complete 24 nm microtubule composed of 13 protofilaments (the A subfiber) fused to an incomplete tubule (B subfiber) composed of 10 protofilaments.16 In conventional electron micrographs, there are paired asymmetrical arms at regular intervals of 24 nm along the A subfiber that project toward the B subfiber of the neighboring doublet (Fig. 1–6). These arms contain the majority of the adenosine triphosphatase (ATPase) activity of the cilium.17 Ciliary ATPases are known as dyneins; hence the arms are called dynein arms. Replicas of rapidly frozen and deeply etched cilia have shown a complex substructure to the dynein arms. The outer dynein arms are all similar and equally spaced at 24 nm intervals. Each has a globular head that interacts with two smaller globular units attached to the A subfiber and is connected by a thin stalk to the B subfiber of the neighboring doublet (Fig. 1–7).18 Inner arms occur in groups of three consisting of two arms with two heads each and one arm with three heads. The arms occur in sequence with a 24 to 32 to 40 nm spacing in a specific relationship with the radial spokes.19 When studied biochemically, axonemes contain multiple dynein heavy chains with molecular weights greater than 300,001, each with specific binding sites for smaller associated proteins.
FIGURE 1–6 Cross section of an axoneme of a detergent-extracted nasal cilium viewed from the tip toward the cell. The plasma membrane was removed by the extraction. The axoneme consists of nine doublet microtubules in a ring surrounding two single microtubules (d, dynein arm; n, nexin link; r, radial spoke) (×250,001).
Each of the A subfibers is also joined on its inner aspect to the B subfiber of the neighboring doublet by a series of fine, highly extensible filaments known as nexin links. At regular intervals of 15 nm along both central singlet tubules, there are paired curved projections 18 nm in length, nearly perpendicular to the plane of the singlet tubules. These projections were known collectively as the central sheath before their structure was known in detail. Radially oriented spokes attached to the A subfibers of the peripheral doublet project inward to the central sheath, ending in a slight enlargement, the spoke head. The spokes are arranged in clusters of three, with an overall spacing of 90 nm, so that the spacing of each group of three radial spokes exactly matches six central sheath projections.20
FIGURE 1–7 Diagram of the structure of the dynein arms based on conventional images and three-dimensional replicas of rapidly frozen cilia. (A) Conventional image as seen in thin sections. The A and B subfibers of the microtubular doublet are labeled. Two angulated dynein arms and a radial spoke with spoke head are shown attached to the A subfiber. (B,C) The components of the outer arm as seen in the replicas. (B) The configuration of the absence of adenosine triphosphate (ATP). (C) With ATP. (From Goodenough UW, Heuser JE. The substructure of the outer dynein arm. J Cell Biol 1982;95: 798–815. Used with permission.)
The B subfibers, dynein arms, and radial spokes terminate 10 to 20 nm from the tip of the cilium, whereas the A subfibers continue into the tip to end in an electron-dense plaque that fills the 20 nm gap between the axoneme and the membrane over the tip. A cluster of five to eight bristles 25 to 30 nm in length anchored to the plaque penetrates the plasma membrane, forming the ciliary crown, a special glycocalyx on the tip of each cilium.21,22 The ciliary crown is postulated to play a role in coupling the motile cilia to the overlying mucous blanket.
At the junction of the cilium and the apex of the ciliated cell, there is a specialized region known as the ciliary neck. In this region, the central singlet micro-tubules terminate, and radial spokes and dynein arms disappear. The doublet fibers are joined to one another and are connected to the plasma membrane by delicate Y-shaped linkers. Where the Y-shaped linkers join the membrane, freeze-fracture preparations show there are distinct circumferential rows of intramembranous particles, the ciliary necklace.23
The basal bodies, of which the cilia are an outgrowth, are cylindrical structures ~0.4 μm in length located in the apex of the cell. They are composed of nine triplet microtubules, two tubules continuous with the A and B subfibers of the axonemal doublet tubules, fused to a third (C) subfiber. The triplets are twisted to an angle of 30 to 40 degrees in a “pinwheel” arrangement. Among the accessory structures attached to the basal bodies are striated rootlets, which extend a few tenths of a micrometer into the cytoplasm, and a lateral triangular projection 0.1 μm in length, the basal foot, which is a nucleating site for cytoplasmic microtubules.24
The beating of the cilia is a complex movement; there is a rapid planar effective stroke in the direction of fluid movement in which the cilia engage the bottom of the mucous gel, followed by a slower recovery stroke in which the cilia recoil while swinging in a clockwise direction beneath the gel.25 The plane of the effective stroke is perpendicular to the plane of the central singlet fibers, and the basal foot protrudes in the direction of the effective stroke.
The mechanisms of ciliary movement are poorly understood. The energy for ciliary beating is provided by ATP. Dynein is activated by Ca2+, and also requires Mg2+ for optimal ATPase activity. The power for ciliary beating is generated by the sliding of groups of peripheral doublets relative to their neighbors driven by changes in the configuration of the globular heads of the dynein molecules. As the sliding is unidirectional, different groups of doublets may be activated during the effective stroke and during the recovery phase of the beat cycle.15 The radial spokes and central pair-sheath complex are thought to participate actively in the conversion of the interdoublet sliding into the complex waveforms taken by cilia.20
The rate of respiratory ciliary beating is by intracellular calcium concentrations, and the coordination of neighboring cells by the passage of calcium-releasing second messengers (such as inositol triphosphate) through gap junctions between the cells.26 Fields of beating cilia are then coordinated in metachronal waves by mechanical interaction of the cilia.
Another function of ciliated cells is control of the depth and composition of the periciliary fluid, which is critical for effective ciliary function. Airway epithelium actively transports ions (sodium away from the bronchial lumen and chloride toward it), while water follows the resultant osmotic gradient.27,28 One of several proteins controlling this flux is the protein encoded by the gene that undergoes mutation in cystic fibrosis, CFTR, a tightly regulated chloride channel localized to the apical membrane of ciliated epithelial cells.
Mucus-Secreting Cells
The mucous gel is secreted by specific secretory cells in the tracheobronchial surface epithelium and in the glands of the subepithelial compartment. Two types of mucus-secreting cells have been identified in the surface epithelium by electron microscopy. Typical goblet cells are characterized by a bulging apex distended with coalescing secretory vacuoles.29 As seen with the electron microscope, these vacuoles contain fibrillar electron-lucent material (Fig. 1–8).
FIGURE 1–8 Goblet cell in the surface epithelium of a bronchus. The cytoplasm is relatively electron dense. The apical cytoplasm is filled with coalescent vacuoles containing electron-lucent mucus (×10,001).
The apex of the goblet cells is covered by microvilli, and the cytoplasm is electron dense and contains a considerable amount of lamellar endoplasmic reticulum compressed into the basal and lateral portions of cytoplasm. Other mucus-secreting cells share the electron dense cytoplasm and other cytoplasmic features of the goblet cells but have only a few small mucus-filled secretory vesicles. These have been called “small mucous granule cells” (SMGC),29 recognizing that these cells may not be a separate type of cell but only in a different phase of the secretory cycle than the goblet cells. A few bronchial columnar cells have neither cilia nor mucous granules. Such “indifferent” cells are infrequent and may represent a waystation between basal cells and one or both of the differentiated columnar cell types.
Mucous Glands
The mucous glands are compound tubular glands (Fig. 1–9) that lie deep to the muscle, in the subepithelial compartment of the bronchi between the cartilage and surface epithelium. They often extend through the gaps between cartilages to occupy the connective tissue of the adventitia. Their three-dimensional organization has been described in detail by Meyrick and colleagues.30 The secretory tubules drain into collecting ducts that discharge into the bronchial lumen at an estimated frequency of approximately one per square millimeter of bronchial surface in the central bronchi. The glands decrease in frequency distally, ultimately disappearing at the same level as the cartilage, at the 10th generation on the average.
FIGURE 1–9 Bronchial mucous gland. The lumen of the bronchus is at the top. One duct branches, giving rise to secretory tubules lined by mucous cells in their proximal portions and serous cells in their terminal portions. The small dark cells in the interstitium between the tubules are mainly plasma cells (×100).
Three types of cells are recognized in the secretory tubules: mucous, serous, and myoepithelial cells (Fig. 1–10). Serous cells are most numerous at the ends of the secretory tubules. Their outline is roughly triangular with a basally placed nucleus and a polarized cytoplasm. The apical portion of the cell contains large eosinophilic granules, whereas the basal portion is strongly basophilic because of its high content of lamellar endoplasmic reticulum. The secretory granules contain asparagines-linked glycoproteins, including several specific proteins found in bronchial secretions [lysozyme (Fig. 1–11), antileukoprotease, and lactoferrin].31,32
Mucous cells have basally located, rather pyknotic nuclei. The entire cell is filled with secretory vesicles with pale electron lucent fibrillar content, which compress the mitochondria and endoplasmic reticulum into a small intervening volume of electron dense cytoplasm. The Golgi apparatus is well developed and is found near the nucleus. The apical surface of the secretory cells is covered with short microvilli. The exposed surface is increased by the presence of intercellular canaliculi.33
FIGURE 1–10 Mucous gland. The serous cell (S) has extensive endoplasmic reticulum in its basal portion and discrete apical granules. The cytoplasm of the mucous cell (M) is more dense, but the secretions are more lucent. A projection of the cytoplasm of a myoepithelial (ME) cell lies inside the basal lamina (arrows) (×6700).
The myoepithelial cells have the cytoplasmic features of smooth muscle. Their cytoplasm is filled with contractile microfilaments with focal, dense attachment bodies, and they express the smooth muscle isoform of actin. However, like epithelium, they are enclosed within the basal lamina of the glands and are joined to the secretory cells by desmosomal junctions. Their appearance and location suggest that their function is to contract, milking the secretions toward the lumen. Cholinergic nerve endings have been identified among the various types of epithelial cells of the glands.33
Initially, both myoepithelial and mucous-secreting cells continue to line the secretory tubules as they coalesce to form the collecting duct. At variable distances along the duct, the lining epithelium changes to cuboidal or columnar cells, which are rather nondescript in the light microscope and are characterized ultrastructurally by the possession of a microvillous apical border and a large perinuclear Golgi apparatus, but no apparent secretory granules (Fig. 1–12). Near the termination of the duct at the bronchial lumen, the epithelium again changes, becoming ciliated.
Plasma cells are often found in considerable numbers in the interstitial tissue around and between secretory tubules. There are nearly equal numbers of IgA- and IgG-containing plasma cells, but only a few cells containing IgM.34 The immunoglobulin in mucus is principally 11S secretory IgA, consisting of two IgA molecules joined by J-protein and complexed to a “secretory piece.” The IgA and J-piece are produced in plasma cells and complexed to the secretory piece at the membrane of the epithelial cells of the secretory tubules and transported across the epithelium to the tubular lumen.35
FIGURE 1–11 Mucous gland stained by the immunoperoxidase method using an antiserum to lysozyme. The serous demilunes show the dark reaction product (×400).
Neuroendocrine Cells
Argyrophilic stains and electron microscopy both demonstrate cells containing large numbers of cytoplasmic granules 0.1 to 0.2 μm in diameter, which have been variously named neuroendocrine cells, Feyrter cells, Kulchitsky (K) cells, amine precursor uptake and decarboxylation (APUD) cells, and “small-granule” cells.36 These cells are more numerous in fetal than adult tissues and are most plentiful in small airways (bronchioles), although they also can be found in large airways and in ducts of mucous glands.37–40 They occur in two anatomic forms (Fig. 1–13). Solitary endocrine cells are found near the basal lamina between columnar cells. These endocrine cells are flask shaped and send out an apical extension between the columnar cells to the bronchial lumen, where they have a small exposed surface. Organized or corpuscular collections of neuroendocrine cells occur near bronchial branch points. The organized clusters, called neuroepithelial bodies by Lauweryns and Goddeoris,41 are innervated by both afferent and efferent nerves and are associated with capillaries of the fenestrated type in the underlying lamina propria.
FIGURE 1–12 Epithelial cell lining the collecting duct of a mucous gland. The luminal surface has a few microvilli. The cell has a relatively large supranuclear Golgi apparatus but no secretory granules. The granules in the cell at the top right have the appearance of lysosomes (×8001).
The histochemical properties of the solitary endocrine cells and the cells of neuroepithelial bodies are similar but not identical. Cells of both types are argyrophilic and show formaldehyde-induced fluorescence with a fluorescent spectrum characteristic of 5-hydroxytryptamine. They express neural markers by immunohistochemistry, including neuronspecific enolase, protein gene product 9.5, chromogranin A, leu 7, and synaptophysin.42,43 Peptide hormones demonstrated in neuroendocrine cells include gastrin-releasing peptide, substance P, endothelin, calcitonin, and calcitonin gene–related peptide, a second peptide derived from the primary transcript of the calcitonin gene by alternative splicing. Leucine enkephalin can be identified in a few solitary neuroendocrine cells but not in neuroepithelial bodies.44–46
The functions of neuroendocrine cells are uncertain. Their morphology strongly suggests a secretory function, either paracrine or endocrine. Their greater abundance in the fetus suggests that they may have an important function in utero. One neuroendocrine cell product, gastrin-releasing peptide, has mitogenic activity for airway epithelium and enhances the growth and differentiation of fetal lung.47 Various products of neuroendocrine cells influence ciliary beating, mucus secretion, and smooth muscle contraction. Lauweryns and coworkers48,49 first proposed the hypothesis that neuroepithelial bodies are chemoreceptors sensitive to hypoxia and mediating hypoxic vasoconstriction. Supporting this suggestion are observations that hypoxia activates membrane potassium channel activity in the neuroepithelial bodies and that the activation is linked to the oxygen-binding protein reduced nicotinamide adenine dinucleotide phosphate (NADPH) oxidase,50 properties that neuroepithelial bodies share with the chemoreceptor cells of the carotid body. Furthermore, the cells degranulate when exposed to acute hypoxia, but not when perfused with hypoxemic blood.49
FIGURE 1–13 Bronchial cells. (A) Solitary endocrine cell in a human bronchus (Grimelius argyrophilic stain, ×1100). (B) Neuroepithelial body in a human bronchus (Grimelius stain, ×1100). (C) Solitary endocrine cell in a rabbit bronchiole (formaldehyde-induced fluorescence, ×400). (D) Neuroepithelial body in a rabbit lung (formaldehyde-induced fluorescence, ×400). (E) Granules in an endocrine cell of a human neuroepithelial body (×32,001). (A–D from Cutz E. Neuroendocrine cells of the lung: an overview of morphologic characteristics and development. Exp Lung Res 1982;3:185–208. Used with permission.)
Bronchioles
The walls of the airways less than 1 mm in diameter lack cartilage and consist of smooth muscle enclosed in a thin connective tissue space. The histologic appearance of the bronchioles varies considerably, depending on the degree of expansion of the lung. In unexpanded lung, the mucosa is thrown into longitudinal ridges that appear papillary in cross section, whereas in the fully expanded lung the mucosa is thin with a smooth circular outline. The mucosa is of a simple columnar type. Basal cells are absent, and without the use of special stains for endocrine cells, only two types of cells can be identified: ciliated cells and nonciliated secretory cells, the so-called Clara cells.51 Mucous cells are not normally found, but they may be present in a variety of diseases and are common in lung exposed to chronic respiratory irritants such as tobacco smoke.
The nonciliated cells of membranous bronchioles are columnar cells with a basal nucleus, a well-developed rough endoplasmic reticulum, Golgi apparatus, numerous mitochondria, and secretory granules in the apical cytoplasm (Fig. 1–14). The apical cytoplasm often projects as a dome above the level of the apices of the more numerous ciliated cells and hence can be readily identified in the scanning electron microscope (Fig. 1–15). The secretory granules of human nonciliated bronchiolar cells are 0.3 to 0.8 μm in diameter, membrane limited, and nonuniform in electron density with electron dense condensations against a more lucent background matrix. In respiratory bronchioles, the proportion of ciliated cells decreases, and in distal respiratory bronchioles ciliated cells often disappear completely (Fig. 1–16). The nonciliated cells become cuboidal, and their granules have a homogeneous electron dense matrix.52
FIGURE 1–14 Epithelium of a membranous bronchiole. A Clara cell with a few secretory granules just beneath the apical membrane is sandwiched among several ciliated cells (×5001). Inset: The granules of a Clara cell have a flocculent texture (×31,001).
FIGURE 1–15 Mucosal lining of a bronchiole from a child’s lung. The Clara cells protrude above the level of the cilia (scanning electron micrograph, ×4001).
The bronchioles are lined by fluid that is covered by a surfactant film.53–55 Its lipid components and some proteins probably are transported from the alveoli, but additional proteins in the hypophase are contributed by Clara cells. Clara cells synthesize several secretory proteins, including surfactant apoproteins A, B, and D,56,57 and the low molecular weight inhibitor of leukocytic proteinases, antileukoproteinase. They also produce a unique 10 kd homodimeric protein, which has substantial homology to the rabbit protein uteroglobin.58,59 Its function is unknown, but it binds environmental pollutants, such as polychlorinated biphenyls. Because the cuboidal nonciliated cells of the distal respiratory bronchioles lack the 10 kd specific protein, they are distinct from the columnar Clara cells of more proximal bronchioles.58
Nonciliated bronchiolar cells also serve as stem cells in the repair of bronchiolar injury and can proliferate and differentiate into ciliated cells following necrosis of bronchiolar epithelium.60 Nonciliated bronchiolar cells in rodents are a rich source of mixed-function oxidases and are important in the metabolism of a variety of simple organic chemicals, some of which are transformed to toxic intermediates that selectively damage the nonciliated cells.61,62 Rodent nonciliated bronchiolar cells possess an extensive smooth endoplasmic reticulum, the presumed site of mixed function oxidases, whereas human nonciliated bronchiolar cells have mainly rough endoplasmic reticulum. Because of this difference, it may not be valid to extrapolate the rodent experiments to humans.
The Acini
General Organization
Acini are units of lung supplied by a single terminal bronchiole. All the air passages constituting an acinus partake to some extent in gas exchange and all have alveoli. The components are respiratory bronchioles, alveolar ducts, and alveolar sacs (Figs. 1–17 and 1–18). Respiratory bronchioles are air passages composed in part of muscular bronchiolar wall covered with cuboidal epithelium and in part of alveoli. Alveolar ducts are conducting structures lined entirely by alveoli and lead to a final generation of alveolus-lined spaces that end blindly, the alveolar sacs. Three-dimensional reconstruction of acinar structure is exceedingly time-consuming, and only a few acini have been analyzed completely. Boyden63 spent 3 years reconstructing a single acinus from the lung of a 6-year-old child by serial sectioning. Others have carefully analyzed and dissected casts (Fig. 1–19),64–66 although in casts of airspace lumina it is often impossible to distinguish respiratory bronchioles from alveolar ducts.
Acini vary considerably in their detailed structure, depending on the space to be filled. Some abut the pleura or perilobular septa, some occupy the niches between bifurcations of bronchi or vessels, and some are entirely enclosed by lung parenchyma. The number of generations of respiratory air passages from terminal bronchiole to alveolar sac is variable even within a given acinus, ranging from 4 to 10. The first-generation respiratory bronchiole is usually mainly bronchiolar in structure, with an average of only three alveoli; subsequent generations of respiratory bronchioles consist mainly of alveoli with a cuboidal bronchiolar type of lining limited to one side where the accompanying artery runs. Usually after two or three generations of respiratory bronchioles, the passages become fully alveolated alveolar ducts. There are from two to six generations of alveolar ducts, the final generation in a given pathway giving rise to as many as six alveolar sacs. Individual respiratory bronchioles or alveolar ducts vary in length, number of alveoli, and angle and pattern of branching. Although dichotomous branching predominates, trichotomy and greater degrees of branching are often encountered.
FIGURE 1–16 Epithelium of a respiratory bronchiole.The epithelial cells are cuboidal and the secretory granules are solid, differing in texture from those in the more proximal bronchiole in Fig. 1–15 (×9001). Inset: Granules at greater magnification (×28,001).
FIGURE 1–17 Schematic diagram of the components of an acinus. AD, alveolar duct; AS, alveolar sac; RB, respiratory bronchiole; TB, terminal membranous bronchiole. From Thurl-beck WM. Chronic obstructive lung disease. In: Somers SC (ed). Pathology Annual. New York: Appleton-Century-Crofts; 1968:377.
The Blood Supply to the Acinus
The pulmonary arteries accompany the bronchi and bronchioles, usually sharing a common connective tissue sheath. The muscular arteries are only slightly smaller than the bronchioles they accompany. Within the acinus, the diameter of the arteries decreases more rapidly than the air passages, so that in the periphery of the lung, the diameters of the arterial branches are much smaller than those of respiratory bronchioles or alveolar ducts they accompany. Some muscular pulmonary arteries do not branch with the bronchial tree but arise as side branches; these vessels have been termed supernumerary.
FIGURE 1–18 Histologic section showing the components of an acinus. A, muscular pulmonary artery; V, vein. The remaining abbreviations are listed in Fig. 1–17.
The muscular arteries give rise to precapillary vessels, which in turn feed the rich capillary network that forms the major part of the alveolar walls. The capillaries are collected into venules that drain into veins at the periphery of the acini, receiving blood from two or three contiguous acini. Where the interlobular septa are well formed, the veins lie within the septa (Fig. 1–18). Thus the blood enters the acini along the bronchioles, is distributed through the alveolar walls, and is collected into veins located at the periphery.5
Alveolar Ducts
The organization of the alveolar ducts has been the subject of several studies because it is critical to the process of ventilation.67–69 The alveoli, which evaginate from the alveolar ducts, vary in size and shape but are on the average hexagonal in outline and 150 to 200 μm in diameter in the fully distended lung. In ordinary histologic sections, the free tips of the interalveolar septa are enlarged and contain smooth muscle and connective tissue fibers. These enlargements are the wall of the alveolar duct proper. Studies using thick or serial sections show that the smooth muscle and accompanying bundles of collagen and elastic fibers spiral down the alveolar duct like a spring. Alveoli are arranged in tiers between the thick spiral fiber bundle, with thinner rings of collagen and elastic fibers at their mouths (Fig. 1–20). The elongation and enlargement of the alveolar ducts during inspiration take place in part by axial elongation of the connective tissue fiber bundles but also in part by uncoiling of the spring.67,68
Alveoli evaginating from adjacent alveolar ducts or sacs are packed in an interdigitating manner similar to the packing of soap bubbles so that the junctions of alveoli are always formed of three alveolar walls.69 The walls separating adjacent alveoli are composed of interwoven networks of connective tissue and capillaries covered by a thin layer of epithelium. The alveolar capillaries are arranged in a net or grid-like pattern (Fig. 1–21), the mesh of which is tightest where the wall separates two airspaces and somewhat more open where an airspace abuts the pleura, interlobular septum, or bronchovascular sheath.5 An analysis of the organization of the capillary net by Sobin and associates70 indicates that the blood flow through the lung is approximated by a laminar flow model in which the blood is spread into a thin film percolating between “posts” (the spaces between capillaries) rather than the more usual model of flow through tubes.
FIGURE 1–19 Partially filled cast of an acinus to demonstrate the variability and complexity of branching. Abbreviations are listed in Fig. 1–17.
The interstitial connective tissue of the airspace walls contains types I, III, and V collagen and elastic fibers.71 Generally, the collagen and elastic fibers run together in bundles that are interwoven through the capillary net (Fig. 1–22) so that in most planes of section, the connective tissue and capillary appear side by side. Thus, in cross section, the capillary has a thin blood–air barrier on one side, where there is no connective tissue bundle, and a thick blood–air barrier on the opposite side, where the connective tissue bundle is found (Fig. 1–23). This organization provides support for the alveolar wall while minimizing the barrier to diffusion.72,73
Alveolar Walls
The principal cells composing the walls of the interalveolar septa are the alveolar epithelium, endothelium, and interstitial cells.74 There are nearly equal numbers of endothelial cells and interstitial cells, and both types are more plentiful than epithelial cells (Fig. 1–24). The epithelial cells, which cover the alveolar septa, are of two types. One, commonly called the type I epithelial cell or membranous pneumonocyte,75
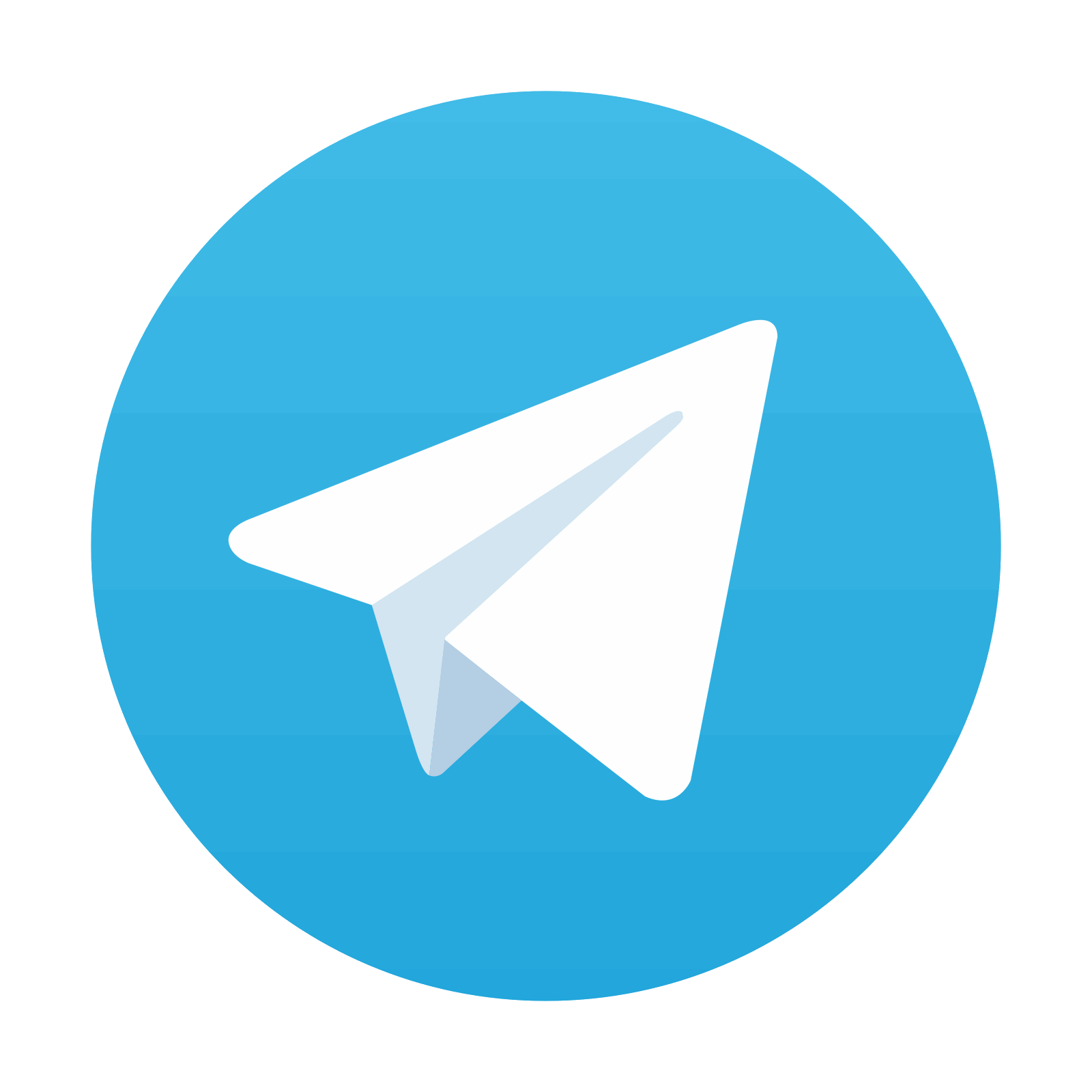
Stay updated, free articles. Join our Telegram channel

Full access? Get Clinical Tree
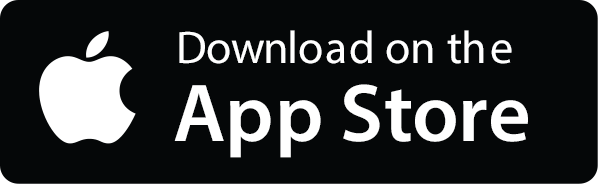
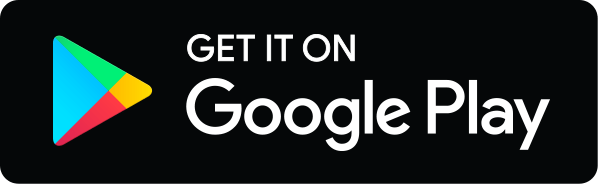