Objectives
- 1.
Explain the circulatory and ventilatory changes associated with exercise.
- 2.
Outline three ways that exercise testing can be performed.
- 3.
Describe the five stages of fetal lung development.
- 4.
Explain fetal circulation and the changes that occur during and immediately after birth.
- 5.
Explain the circulatory and ventilatory changes associated with altitude and the physiologic components of acclimatization.
The lung can adapt to a number of special environments and special circumstances, some of which are described here.
Exercise
The ability to exercise depends on the capacity of the cardiac and respiratory systems to increase oxygen delivery to the tissues and to remove carbon dioxide from the body ( Fig. 12.1 ). During exercise, energy metabolism is increased through muscle contraction and the conversion of glucose to chemical energy during moderate exercise and the generation of lactic acid during strenuous exercise. Also involved in exercise is the central nervous system (CNS), which delivers coordinated signals to the musculoskeletal system, and the autonomic nervous system, which redistributes blood flow among various organs to meet the demands of exercise.

V ˙
o 2 ) and carbon dioxide production ( <SPAN role=presentation tabIndex=0 id=MathJax-Element-2-Frame class=MathJax style="POSITION: relative" data-mathml='V˙’>V˙V˙
V ˙
co 2 ).
Both tidal volume and respiratory rate increase with exercise, resulting in an increase in minute ventilation ( Fig. 12.2 ). The increase in minute ventilation with exercise is linearly related to both CO 2 production and O 2 consumption at low to moderate levels ( Fig. 12.3 ). With maximal exercise, a fit young man can achieve an oxygen consumption of 4 L/min with a minute volume of 120 L/min, almost 15 times resting levels, and a cardiac output that increases only 4 to 6 times above resting level. As a result, in normal individuals, the cardiovascular system and not the respiratory system is the rate-limiting factor in exercise.


V ˙
a ) during exercise to maintain the Paco 2 at 40 mm Hg. Levels of ventilation at a moderate level of exercise CO 2 = 2 L/min) change with different levels of Paco 2 . B, CO 2 production and O 2 consumption are linearly related.
Work of breathing is also increased during exercise secondary to increases in both lung and chest wall elastic recoil and increases in airway resistance. Larger tidal volumes result in higher lung volumes, and both the lung and the chest wall become less compliant at these higher lung volumes, resulting in increased work to overcome the lung and chest wall elastic recoil. In addition, the airway resistance component of work of breathing increases with the higher flow rates generated during exercise ( Table 12.1 ). These normal changes in work of breathing are exaggerated in individuals with abnormalities in pulmonary mechanics due to airway obstruction or to changes in lung compliance or in oxygenation and can result in exercise limitation.
Variable | Moderate Exercise | Severe Exercise |
---|---|---|
Mechanics of Breathing | ||
Elastic work of breathing | ↑ | ↑↑ |
Resistance work of breathing | ↑ | ↑↑ |
Alveolar Ventilation | ||
Tidal volume | ↑↑ | ↑↑ |
Frequency | ↑ | ↑↑ |
Anatomic dead space | ↑ | ↑ |
Alveolar dead space (if present) | ↓ | ↓ |
V D /V T | ↓ | ↓↓ |
Pulmonary Blood Flow | ↑ | ↑↑ |
Perfusion of upper lung | ↑ | ↑↑ |
Pulmonary vascular resistance | ↓ | ↓↓ |
Linear velocity of blood flow | ↑ | ↑↑ |
Ventilation–Perfusion Matching | ↑ | ↑ |
Diffusion through the alveolar–capillary barrier | ↑ | ↑↑ |
Surface area | ↑ | ↑↑ |
Perfusion limitation | ↓ | ↓↓ |
Partial pressure gradients | ↑ | ↑↑ |
Oxygen Unloading at the Tissues | ↑ | ↑↑ |
Carbon Dioxide Loading at the Tissues | ↑ | ↑↑ |
PA o 2 | ↔ | ↑ |
Pa o 2 | ↔ | ↑, ↔, or ↓ |
Pa co 2 | ↔ | ↓ |
pH | ↔ | ↓ |
O 2 extraction ratio | ↑ | ↑↑ |
Lung Volume Changes with Exercise
With exercise, the increase in tidal volume occurs mainly through the inspiratory reserve capacity. Total lung capacity decreases slightly as the central blood volume increases (secondary to increased venous return). Residual volume (RV) and functional residual capacity (FRC) are unchanged or increase slightly. Anatomic dead space increases slightly as a result of airway distention at higher lung volumes. This is associated with a decrease in alveolar dead space as cardiac output increases with exercise. The net effect is no change in physiologic dead space. The ratio of dead space volume to tidal volume (V ds /V t), however, decreases as V t increases.
Pulmonary Blood Flow During Exercise
Cardiac output increases linearly with oxygen consumption during exercise ( Fig. 12.4 ). The increase in cardiac output is secondary to primarily an increase in heart rate and increased venous return due to deeper inspiratory efforts. Mean pulmonary artery and mean left atrial pressures increase out of proportion to changes in pulmonary blood flow. As a result, there is a decrease in pulmonary vascular resistance. Recruitment of pulmonary blood vessels occurs, especially in upper regions of the lung, and this is associated with a decrease in the regional inhomogeneity observed at rest.

Exercise results in a more uniform ventilation-perfusion ratio ( <SPAN role=presentation tabIndex=0 id=MathJax-Element-4-Frame class=MathJax style="POSITION: relative" data-mathml='V˙/Q˙’>V˙/Q˙V˙/Q˙
V ˙ / Q ˙
) throughout the lung, with regional ratios close to 1.0 ( Fig. 12.5 ). Increased pulmonary blood flow during exercise increases the diffusion capacity as oxygen uptake increases. The surface area for diffusion increases as pulmonary blood flow to the upper lung regions increases. Increased velocity of blood flow occurs, and this maintains the partial pressure gradient for diffusion. At maximum levels of exercise associated with high blood velocities, diffusion limitation of gas transfer can occur even in healthy individuals.

V ˙
a / <SPAN role=presentation tabIndex=0 id=MathJax-Element-6-Frame class=MathJax style="POSITION: relative" data-mathml='Q˙’>Q˙Q˙
Q ˙
a ) in the lung at rest and during exercise. During exercise the <SPAN role=presentation tabIndex=0 id=MathJax-Element-7-Frame class=MathJax style="POSITION: relative" data-mathml='V˙’>V˙V˙
V ˙
a / a is more homogeneous than at rest.
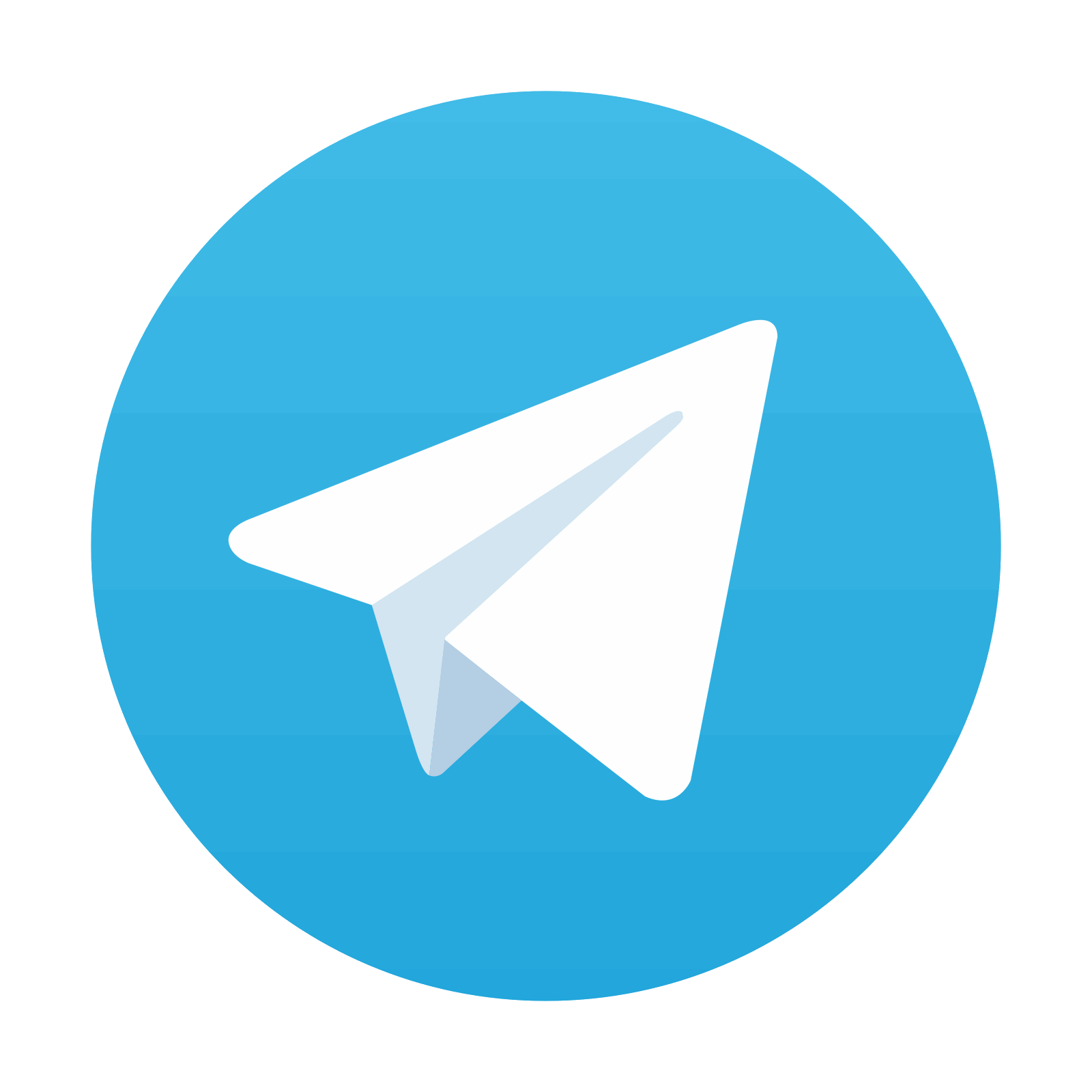
Stay updated, free articles. Join our Telegram channel

Full access? Get Clinical Tree
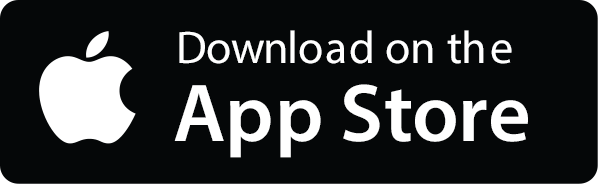
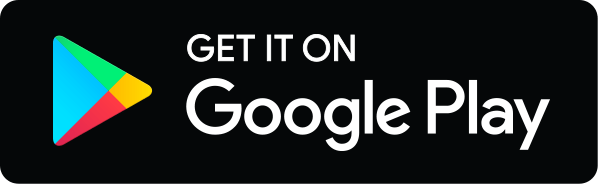
