Peter Sassalos, Ming‐Sing Si, Jennifer C. Romano, Edward L. Bove, and Richard G. Ohye University of Michigan Medical School, Ann Arbor, MI, USA The field of congenital heart surgery has made great advancements over the last 75 years. Today, complete neonatal repairs are preferred to palliative procedures and new frontiers such as mechanical circulatory support and transplantation, hybrid interventions, and adult congenital heart surgery have evolved. With these advances, patients can now survive and enjoy excellent quality of life into adulthood. This progress is perhaps most dramatic in patients with functionally univentricular hearts. The cardiac lesions in this group are some of the most challenging to manage. Although great strides have been taken, there are still significant challenges in understanding, management, and therapies ahead. Many terms are used interchangeably to discuss this group of cardiac lesions, including single ventricle, functional single ventricle, univentricular heart, and functionally univentricular heart. Before an in‐depth discussion can ensue, a proper definition of a functionally univentricular heart must be established. The definition provided by Jacobs and Anderson is the most appropriate: “the entire ventricular mass was functionally univentricular whenever one or other ventricle was incapable, for whatever reason, of supporting either the systemic or the pulmonary circulation” [1]. The segmental approach of Van Praagh [2] and the sequential segmental approach of Anderson [3] account for the way in which the components of the heart are joined together. This still requires an account of the lesions to be found within the heart. In this regard, controversies continued as to how best to describe the chambers within the ventricular mass. In an attempt to resolve these issues, the European Association for Cardiothoracic Surgery (EACTS) and the Society of Thoracic Surgeons (STS), along with societies representing pediatric cardiologists, joined to form the International Society for Nomenclature of Pediatric and Congenital Heart Disease (ISNPCHD). This Society, in turn, then created the International Pediatric and Congenital Cardiac Code (IPCCC) [4, 5]. Based on a consensus of nomenclature from this group [1], the IPCCC includes the following as functionally univentricular hearts listed as “single ventricle (functionally)” [6]: double‐inlet left ventricle (DILV), double‐inlet right ventricle (DIRV), mitral atresia, tricuspid atresia, unbalanced atrioventricular septal defect (AVSD), single ventricle and heterotaxy syndrome, single ventricle other, and single ventricle and total anomalous pulmonary venous connection (TAPVC). Although several other lesions are not listed under “single ventricle” in the IPCCC code, they produce similar pathophysiology and ultimately share a common treatment algorithm. They also satisfy the definition offered by Jacobs and Anderson [1]. These lesions are hypoplastic left heart syndrome (HLHS), pulmonary atresia with intact ventricular septum (PA/IVS), biventricular hearts with straddling atrioventricular valves, and complex forms of double‐outlet right ventricle (DORV). A double‐inlet ventricle is a rare cardiac lesion in which both atrial chambers connect to a dominant ventricle, either through two separate atrioventricular valves or a common atrioventricular valve. Overriding of one of the atrioventricular valves must be greater than 50% to be classified as double‐inlet ventricle. The hypoplastic chamber, which is incomplete in that it lacks an inlet component, is of the opposite morphology. When the dominant ventricle is of left morphology, the connection between the two chambers used to be termed a “bulboventricular foramen.” In fact, it is a ventricular septal defect. In the setting of DILV, it is the size of this channel and the location of the aorta that determine the degree of systemic outflow obstruction [7]. DILV is the commonest variant, and its own commonest pattern is when the ventriculoarterial connections are discordant and the incomplete right ventricle is left‐sided. The right‐sided dominant left ventricle then gives rise to the pulmonary trunk, with the left‐sided incomplete morphologically right ventricle giving rise to the aorta [7]. A less common variant occurs when the ventriculoarterial connections are concordant. In this setting, the incomplete morphologically right ventricle is right‐sided, as it can also be when the ventriculoarterial connections are discordant. In some circumstances, there can be double outlet from either the dominant or the incomplete ventricles. These are rare, as are the other forms of double inlet, which can be to a dominant right ventricle, or a solitary and indeterminate ventricle (DIIV). These latter variants are more often associated with isomerism of the atrial appendages, and typically have double outlet with or without pulmonary atresia [7]. Mitral atresia is defined as atresia of the left atrioventricular connection. When found with aortic stenosis or atresia, the patient is considered to have HLHS. If the atrial septum is restrictive or intact, the prognosis is worse given the usual association of pulmonary venous hypertension. Mitral atresia is usually associated with concordant ventriculoarterial connections or DORV. It is rarely associated with transposition [8]. Tricuspid atresia has been the traditional symbol of functionally univentricular hearts, as it was the first condition to be treated with the Fontan procedure. Tricuspid atresia is defined as atresia of the right atrioventricular connection and is most commonly due to absence of the right atrioventricular connection. In this setting, the fibro‐adipose tissue of the right atrioventricular junction separates the floor of the right atrium from the incomplete right ventricle. In rare cases, nonetheless, there may be an imperforate tricuspid valve, which can show evidence of Ebstein malformation [7]. Consistent anatomy includes an atrial septal defect as the sole egress of the right atrium, a patent left atrioventricular connection, a hypoplastic right ventricle, and a dominant left ventricle. Variable anatomy includes the presence and size of a ventricular septal defect, variation in the ventriculoarterial connections, and the degree of pulmonary stenosis. A time‐honored system for classification was based on the ventriculoarterial connections. So‐called type I has concordant ventriculoarterial connections with spiraling and normally related great arteries. It is the commonest variant. In type II, the ventriculoarterial connections are discordant, while in type III, which is exceedingly rare, there is a common arterial trunk. Further subclassification depends on pulmonary blood flow, and is applicable to the variants with concordant ventriculoarterial connections. In type a, there is pulmonary atresia; in type b, there is subvalvar or valvar pulmonary stenosis; while type c, which is rare, has no pulmonary stenosis [9]. The remainder of the cardiac anatomy is typically characterized by normal systemic venous connections, usual atrial arrangement, right‐handed ventricular topology, usual coronary arteries, and a normal conduction system [7]. An unbalanced AVSD occurs when the left and right components of the common atrioventricular valve are not equally distributed over the ventricles. This causes unequal blood flow from the atrial chambers to each ventricle. For example, when the valve is displaced rightward, there is increased flow to the right ventricle, resulting in a dominant right ventricle with a hypoplastic left ventricle. In this case, the adequacy of the hypoplastic left ventricle for use in a biventricular repair is, in part, determined by the size of the left atrioventricular valvar component, the left mural leaflet, and the distance between the papillary muscles. This term, as defined by the Nomenclature Committee, is synonymous with the presence of isomeric atrial appendages, as opposed to the usual or mirror‐imaged atrial arrangement. In most instances associated with a functionally univentricular heart, there is right isomerism. As such, there are multiple anomalies, both cardiac and noncardiac [10]. The noncardiac anomalies can involve the lungs, which also show evidence of isomerism, and most of the abdominal organs, which are jumbled up. Abnormal ciliary function can lead to recurrent pulmonary infections and bronchiectasis. There can be absence of the spleen, or multiple spleens, both of which can cause abnormal splenic function and susceptibility to encapsulated bacteria. The liver can be midline, and the stomach is often on the right. The small and large intestines can have abnormal attachments predisposing to malrotation, volvulus, and obstruction [10, 11]. The cardiac anomalies often produce functionally univentricular physiology. Some of these patients, nonetheless, can be managed by biventricular repair. The presentation, based on the degree of systemic and pulmonary blood flow, will depend on the type and severity of anomalies. Abnormal systemic venous connections may consist of bilateral superior or inferior caval veins, interruption of the inferior caval vein with azygous or hemiazygous continuation, variable drainage of the hepatic veins, and aberrant portal veins. There is usually a common atrium due to a poorly developed atrial septum. Common atrioventricular septal defects, often unbalanced, abnormalities of the outflow tracts, and totally anomalous pulmonary venous connection are frequent. There may be pulmonary stenosis or atresia as well as pulmonary arterial abnormalities. Abnormal rhythms, notably atrial arrhythmias and complete heart block, are common [10–12]. The constellation and frequency of anomalies are related to the sidedness. Absence of the spleen is usually associated with bilateral right‐sidedness, or right isomerism, and multiple spleens with bilateral left‐sidedness, or left isomerism [10, 11]. Right isomerism is associated with a worse prognosis than left isomerism [13]. Overall, the so‐called heterotaxy syndrome is made up of a complex group of patients. The diagnosis of a functionally univentricular heart in this setting carries a worse prognosis compared to other types of functionally univentricular hearts. In large series of similar time periods from the Mayo Clinic, the early mortality following a modified Fontan procedure was 9.5% for “heterotaxy syndrome,” compared to 3% for DILV and 2% for tricuspid atresia [14–16]. Totally anomalous pulmonary venous connection is discussed in Chapter 35. It is always present in the setting of right isomerism, even if the pulmonary veins return to the heart. When present in the setting of a functionally univentricular heart, the prognosis is poor. The remaining category of other lesions encompasses very rare cardiac defects in which the morphology of the dominant ventricle is unclear. Functionally univentricular hearts share a common pathophysiology. There are intracardiac shunt(s) present with mixing of blood in parallel systemic and pulmonary circulations, contrary to the normal “in‐series” circulation. The circulation associated with functionally univentricular hearts produces abnormal systemic oxygen delivery and volume loading of the functionally single ventricle. These factors contribute to the poor prognosis if left untreated. The clinical presentation depends on the degree of systemic outflow and pulmonary outflow obstruction. Patients with a balanced circulation may be asymptomatic and present later in life. Those with inadequate pulmonary blood flow will present with cyanosis, and those with excessive pulmonary blood flow will present with heart failure. Those with systemic outflow obstruction significant enough to require ductal patency for systemic output may present in shock. These presentations will determine the need for and type of surgical treatment in the neonatal period. In the current era, patients are often born with a prenatal diagnosis of a functionally univentricular heart, which permits appropriate planning and immediate management after birth. A thorough evaluation to assess for additional noncardiac anomalies along with baseline laboratory studies, a chest radiograph, and electrocardiogram are performed. The mainstay of diagnosis is a transthoracic echocardiogram. Cardiac catheterization, computed tomography, and magnetic resonance imaging have roles in select cases. Once a diagnosis of a functionally univentricular heart is made, surgical palliation culminating in a staged Fontan procedure is planned. Depending on the anatomy and the initial presentation, a neonatal procedure may first be required to achieve the optimal pulmonary blood flow, and relieve any systemic arterial or pulmonary venous obstruction. These procedures may include construction of a systemic to pulmonary artery shunt, banding of the pulmonary trunk, atrial septectomy or septostomy, Damus–Kaye–Stansel procedure, or Norwood procedure. The original criteria for the Fontan procedure were published by Choussat and Fontan in 1977 in the textbook Pediatric Cardiology by Anderson and Shinebourne. These “Ten Commandments” are shown in Table 27.1 [17]. Since then, better understanding of the pathophysiology and surgical techniques has relaxed the indications based on the same principles [18–20]. Currently, to be an appropriate candidate for a Fontan procedure, the contemporary criteria shown in Table 27.1 should be present or achievable with intervention. Table 27.1 Summary of criteria for the Fontan procedure. The left column lists the “Ten Commandments” published by Choussat and Fontan in 1977 in the textbook Pediatric Cardiology by Anderson and Shinebourne [17]. The right column lists the contemporary criteria that should be present or achievable with intervention. The earliest surgical procedures available for a functionally univentricular heart were palliative procedures, such as the Blalock–Taussig–Thomas (BTT) shunt introduced in 1945 [21], and banding of the pulmonary trunk in 1952 [22]. The first anatomic corrective repair was ventricular septation, which was achieved in 1956. It is infrequently used today due to poor results [23]. Later described were the Damus–Kaye–Stansel procedure [24, 25], the modified BTT shunt in 1981 [26], and the Norwood procedure in 1981 [27]. At the same time, active research was being performed to prove the idea of the “dispensable right ventricle” [28–30], forming the basis for the work of William Glenn at Yale University [31–33]. His animal studies showed that a circulation with a superior cavopulmonary shunt was possible without the contribution of the right ventricle. This led to the first description of this operation in 1958, now known as the classic Glenn shunt. Through a right thoracotomy, the azygous vein was ligated, the right pulmonary artery and superior caval vein were each divided, and an end‐to‐side anastomosis was performed between the distal right pulmonary artery and lateral wall of the superior caval vein [34]. The advantage of this shunt over systemic to pulmonary artery shunts, such as the BTT, Potts, and Waterston shunts, was an ability to increase effective pulmonary blood flow while avoiding the unfavorable effects of volume loading the functionally single ventricle. The classic Glenn shunt was used over the next decade and was later modified as an end‐to‐side anastomosis between the superior caval vein and right pulmonary artery, without division of the right pulmonary artery [35]. The benefit was to maintain pulmonary arterial continuity, now known to maintain optimal distribution of inferior caval venous blood to both lungs in a Fontan circulation, which is important in the prevention of pulmonary arteriovenous malformations. This modification is now known as the bidirectional Glenn (BDG) shunt. It was not until 1968 that the first complete physiologic repair of a functionally univentricular heart was performed with a Fontan procedure [36]. Francis Fontan believed in the importance of the pulsatile function of the right atrium and the need for unidirectional flow through valved conduits. The originally described Fontan procedure, therefore, consisted of a classic Glenn shunt, anastomosis of the right atrial appendage to the divided proximal end of the right pulmonary artery with or without an interposition of an aortic valve homograft, insertion of a pulmonary valvar homograft at the inferior cavoatrial junction, atrial septal defect closure, and ligation or division of the pulmonary trunk (Figure 27.1) [36]. In doing so, there was separation of the systemic and pulmonary circulation, with drainage of all systemic venous return via the superior caval vein and right atrium to the pulmonary arteries, and all pulmonary venous return via the dominant left ventricle to the aorta. Kreutzer reported his modification of the procedure in 1973, which consisted of inserting a pulmonary autograft to connect the right atrial appendage to the pulmonary trunk, and closure of the atrial septal and ventricular septal defects. The superior cavopulmonary connection and valved conduit at the inferior cavoatrial junction were not performed [37]. Up to this point, the Fontan procedure was only used for tricuspid atresia. In 1976, however, the Fontan procedure was attempted on other functionally univentricular hearts, specifically mitral atresia. New challenges encountered in baffling the pulmonary venous return to the systemic ventricle led to the first description of the lateral tunnel technique in 1987 [38]. At the same time, Marc de Leval described hydrodynamic studies evaluating flow and energy loss using an in vitro model. These studies resulted in a revised Fontan circulation that excluded most or all of the right atrium. The atriopulmonary connection was therefore replaced by a total cavopulmonary connection (TCPC). This procedure consisted of a right‐sided BDG shunt, creation of an “intra‐atrial cavocaval channel” using composite or PTFE (polytetrafluoroethylene) conduit, anastomosis between the transected cardiac end of the superior caval vein to the inferior aspect of the right pulmonary artery with patch augmentation to match the size of the inferior caval vein, and division of the pulmonary trunk (Figure 27.2). The proposed advantages of this TCPC over the prior atriopulmonary connection of the Fontan procedure included technical ease and reproducibility, application to any functionally univentricular heart, avoidance of suturing near the atrioventricular node, decreased risk of arrhythmia by eliminating high pressure in the right atrium, and better flow patterns resulting in decreased turbulence, energy loss, and risk of atrial thrombosis [39]. Figure 27.1 The original Fontan procedure. (A, C) The procedure consisted of a classic Glenn shunt, anastomosis of the right atrial appendage to the divided proximal end of the right pulmonary artery with or without an interposition of an aortic valve homograft, respectively, insertion of a pulmonary valve homograft at the inferior cavoatrial junction, atrial septal defect closure, and main pulmonary artery ligation or division. (B) Contrast injection of the right atrium demonstrates an atriopulmonary connection with opacification of only the left branch pulmonary artery. Source: Reproduced by permission from Fontan F, Baudet E. Thorax. 1971;26(3):240–248. Figure 27.2 The original total cavopulmonary connection type of Fontan procedure. This consisted of a right bidirectional Glenn shunt, creation of an “intra‐atrial cavocaval channel” using composite or polytetrafluoroethylene (PTFE) conduit, anastomosis between the transected cardiac end of the superior caval vein and the inferior aspect of the right pulmonary artery with patch augmentation of the superior caval vein to match the size of the inferior caval vein, and division of the main pulmonary artery. (A) Intra‐atrial cavocaval channel. (B) Completed procedure. Source: Reproduced by permission from de Leval MR et al. J Thorac Cardiovasc Surg. 1988;96:682–695. Despite these advances to the Fontan procedure, there were still less than desirable outcomes. One proposed theory was that the sudden change in volume unloading of the functional ventricle following the Fontan procedure was not well tolerated due to the relationship of ventricular mass to volume. This complete unloading of the ventricle in one stage led to decreased ventricular compliance and diastolic filling, thought to explain the low cardiac output observed as a common early cause of death after the Fontan procedure. Therefore, during the 1980s and 1990s, many advocated staging of the Fontan procedure with a BDG shunt. This provided the ability to volume unload the systemic ventricle earlier in a more tolerated manner. The advantage was more stable physiology in the postoperative period, while minimizing the deleterious long‐term effects of a chronically, volume‐loaded ventricle. Following introduction of staging, early mortality improved [40–45]. This also led to the introduction of the hemi‐Fontan procedure [46, 47]. The bidirectional Glenn and hemi‐Fontan circulations function in a similar fashion and are discussed in depth later in the chapter. Another important modification was introduction of the baffle fenestration, also discussed in detail later [48–50]. Following de Leval’s work, the lateral tunnel was initially the preferred option. Other techniques have since been introduced, which are now more commonly used. The intra/extracardiac conduit was first described to address complex venous anatomy of heterotaxy syndrome by Vargas in Boston in 1987 [51], and later proposed by Jonas [52]. The extracardiac conduit was described by Marcelletti in 1990 [53]. As the staged Fontan pathway was being developed, others were pursuing transplantation as an alternative treatment for functionally univentricular hearts. A strong proponent of transplantation for primary treatment of functionally univentricular hearts, namely HLHS, was Bailey at Loma Linda [54]. Given both donor constraints and advances made in staged palliation, transplantation is not favored for primary treatment in the current era. It is the original and thoughtful work of these early pioneers, which has undergone extensive modifications over the years, that has resulted in the approaches recognized today. The culmination of their efforts has led to the current surgical management (Tables 27.2 and 27.3). Table 27.2 Key operative milestones in the evolution of the staged Fontan pathway for functionally univentricular hearts. When there is inadequate pulmonary blood flow with ductal dependency, an additional source of pulmonary blood flow is required in the form of a systemic to pulmonary artery shunt. Current preferred techniques include the modified BTT shunt, central shunt, right ventricle to pulmonary artery conduit insertion, and placement of a stent in the arterial duct. Surgical shunts can be performed through a median sternotomy or thoracotomy. The appropriate shunt diameter is determined by multiple variables including patient size, vessel diameter, and shunt length. Table 27.3 Treatment operations in the staged Fontan pathway. Systemic to pulmonary artery shunts include classic and modified Blalock–Taussig–Thomas, Waterson, Potts, central, and right ventricle to pulmonary artery conduits. Pulmonary artery band(s) can be placed through median sternotomy or thoracotomy. Surgical atrial septectomy can be performed using the Blalock–Hanlon technique, caval inflow occlusion, or standard cardiopulmonary bypass. Percutaneous atrial septostomy can be performed using the Rashkind technique, static and cutting balloons, or stent placement. Second‐stage and third‐stage palliation can use a variety of cannulation and bypass strategies. Third‐stage palliation can be done with or without a fenestration. This can lead to pulmonary overcirculation presenting as pulmonary edema, mechanical ventilatory dependence, and heart failure, or as systemic hypoperfusion leading to decreased end‐organ perfusion, metabolic acidosis, and shock. A band can be placed on the pulmonary trunk or one of its branches through a median sternotomy or thoracotomy. The tightness of the band is determined by consideration of the Trusler formula, in conjunction with judgment from clinical and echocardiographic assessment. Material choice is based on surgeon preference. Our institution uses PTFE or silicone reinforced with polyester mesh (Mersilene®, Ethicon, Somerville, NJ, USA). The goal is to prevent the pathophysiologic consequences of excessive pulmonary blood flow, protect the pulmonary vasculature from elevated pressure (especially important in a functionally single ventricle), and relieve symptoms of congestive heart failure. If the functionally univentricular heart is dependent on atrial‐level shunting, an intact or restrictive atrial septum could lead to development of left atrial hypertension, pulmonary venous congestion, and irreversible pulmonary vascular changes, therefore nonrestrictive atrial level shunting is imperative. Surgical atrial septectomy can be performed using the Blalock–Hanlon technique, venous inflow occlusion, or standard cardiopulmonary bypass. Percutaneous atrial septostomy can be performed using the Rashkind technique, static and cutting balloons, or stent placement. If there is obstruction or potential obstruction of systemic outflow, it should be addressed early in a functionally univentricular heart. Depending on the anatomy, surgical options include a Damus–Kaye–Stansel procedure [24, 25] or the hybrid or traditional Norwood procedure, which is routinely performed on patients with HLHS and discussed in Chapter 32 [27]. The second stage creates a superior cavopulmonary anastomosis as the source of pulmonary blood flow while volume unloading the ventricle. Other sources of pulmonary blood flow are typically eliminated at this stage. If the pulmonary trunk is divided, the pulmonary valvar leaflets should be resected or incorporated in the suture line to prevent a blind‐ending pouch distal to the valve, which can be a source for systemic thromboembolism [55, 56]. This stage is typically performed between 4 and 6 months of age. A preoperative cardiac catheterization is traditionally performed to assess surgical candidacy, although selective use of magnetic resonance imaging in low‐risk patients is gaining favor at many experienced centers. Major factors considered include patient growth; systemic oxygen saturation; pulmonary arterial size, pressure, and resistance; the state of the shunt if present; atrioventricular valvar regurgitation; systemic ventricular function and pressure; systemic outflow obstruction; and need for additional intervention [47, 57]. Once deemed an appropriate candidate, the options at this stage include HFP, BDG, or the Kawashima procedure. The hemi‐Fontan procedure is the procedure of choice at the University of Michigan. Despite being technically more complex than a BDG, it is favored for several reasons (Figure 27.3) [47]. Routine augmentation of the right and left pulmonary arteries ensures optimal pulmonary artery anatomy, and the more complex operation when performed at this stage simplifies the lateral tunnel Fontan when the postoperative hemodynamics are more demanding. At the Fontan stage, the entire cardiac output, with the exception of a fenestration, passes through the lungs. Long anesthetic and cardiopulmonary bypass times, as well as the need for multiple blood products, can negatively impact the lungs, which more seriously affects the early postoperative course following the Fontan. In addition, longer cross‐clamp and bypass times at the Fontan have been associated with increased early and late mortality [58]. Lastly, based on mathematical modeling, the hemi‐Fontan procedure with lateral tunnel Fontan circulation has more favorable flow patterns, with less energy loss and more equal distribution of inferior caval venous blood flow, as compared to the BDG with extracardiac conduit Fontan circulation (Figure 27.4) [59]. Figure 27.3 Angiograms of the hemi‐Fontan pathway. (A) Anterior–posterior projection demonstrates good‐size branch pulmonary arteries. (B) Lateral projection demonstrates lack of right angles, which is theorized to minimize energy loss. Courtesy of Dr. Jeffrey Zampi, Department of Pediatrics, University of Michigan C.S. Mott Children’s Hospital. The current technique used at the University of Michigan differs from the original description by Norwood because of two modifications. First, the right atriotomy does not cross the cavoatrial junction to avoid potential injury to the artery supplying the sinus node, and subsequent sinus nodal dysfunction. Second, a separate PTFE patch, rather than the allograft patch augmentation of the pulmonary arteries, is used to partition the right atrium, facilitating the lateral tunnel Fontan [47]. Figure 27.4 Mathematical modeling of (A) the hemi‐Fontan pathway and (B) the hemi‐Fontan with lateral tunnel Fontan circulation demonstrates more favorable flow patterns with less energy loss and more equal distribution of inferior caval vein blood flow, compared to the bidirectional Glenn with extracardiac conduit Fontan circulation. The model demonstrates the lack of right angles and the anterior–posterior caval offset. Source: Reproduced by permission of Elsevier from Bove EL et al. J Thorac Cardiovasc Surg. 2003;126(4):1040–1047. This technique has been well described in the literature [47, 60]. Cannulae are placed in the ascending aorta, superior caval or innominate vein, and inferior caval vein. Cardiopulmonary bypass is initiated at 28–34 °C, depending on surgeon preference. If present, native pulmonary blood flow or a systemic to pulmonary artery shunt is occluded. The azygous vein is ligated. The right and left pulmonary arteries are mobilized to the lobar branches and controlled. A longitudinal central pulmonary arteriotomy is made and extended leftward beyond the insertion of the ductal stump and rightward to a point directly posterior to the superior caval vein. An aortic cross‐clamp is applied and antegrade cardioplegia is administered. A right atriotomy is performed from the right atrial appendage toward, but not across, the cavoatrial junction. The apex of the right pulmonary arteriotomy is then sutured to the posterior wall of the superior caval vein, such that its external surface serves as part of the augmentation of the right pulmonary artery. This suture line is carried medially where the inferior edge of the central pulmonary arteriotomy is connected to the posterior edge of the right atriotomy. A PTFE patch is sutured at the level of the border of the oval fossa posteriorly, and then incorporated into the eventual closure between the right atriotomy and patch augmentation of the pulmonary arteries anteriorly. This partitions the hemi‐Fontan pathway from the common atrium. The heart is de‐aired. The aortic cross‐clamp is removed. Allograft patch augmentation of the central pulmonary arteries is then performed, thereby completing the connection as the roof of the pathway. Control of the pulmonary arteries is released. The remainder of the operation is conducted in the usual fashion, including direct pressure measurements of the hemi‐Fontan pathway pressure and common atrial pressure to assess the transpulmonary gradient. Intracardiac pressure monitoring lines are typically not left in the hemi‐Fontan pathway to avoid thrombosis (Figure 27.5). The BDG procedure is currently the more commonly performed second‐stage palliation at most centers. Advocates favor this approach for the technical ease and the ability to perform it without cardiac arrest or even without cardiopulmonary bypass [42, 61]. In addition, despite our institution’s preference for the hemi‐Fontan procedure, in some patients that is technically not possible and a BDG procedure is used instead. Most commonly, this is in the setting of totally or partially anomalous pulmonary venous connection to the superior caval vein, where the vein must be divided above the anomalous connection to preserve pulmonary venous return to the atrium. Other examples include a single left superior caval vein in the setting of the usual atrial arrangement, in which the vein does not connect to the morphologically right atrium, and the usual atrial arrangement with right‐sided heart, or mirror‐imaged arrangement with left‐sided heart, where accessing the morphologically right atrium is difficult due to the overlying ventricular mass. Lastly, various forms of isomerism, where the inferior caval and hepatic veins are on the opposite side to the superior caval vein, can make the procedure impossible. If bilateral superior caval veins are present, we elect to perform a right modified hemi‐Fontan procedure with a left BDG procedure. Figure 27.5 The modified hemi‐Fontan procedure. (A) The cannulation strategy and planned incisions are shown. A longitudinal central pulmonary arteriotomy is made and extended leftward beyond the insertion of the ductal stump and rightward to a point directly posterior to the superior caval vein. A right atriotomy is performed from the right atrial appendage toward, but not across, the cavoatrial junction. (B) The apex of the right pulmonary arteriotomy is sutured to the posterior wall of the superior caval vein, such that the external surface of the superior caval vein serves as part of the augmentation of the right pulmonary artery. This suture line is carried medially where the inferior edge of the central pulmonary arteriotomy is connected to the posterior edge of the right atriotomy. A polytetrafluoroethylene (PTFE) patch is sutured at the level of the limbus of the septum secundum posteriorly and then incorporated into the eventual closure between the right atriotomy and patch augmentation of the pulmonary arteries anteriorly. This partitions the hemi‐Fontan pathway from the common atrium. (C) Allograft patch augmentation of the central pulmonary arteries is then performed, thereby completing the connection as the roof of the pathway. (D) The completed procedure. AP, arterioportal. Source: Reproduced by permission from Hirsch JC et al. In: Pediatric Cardiac Surgery, 4th ed. Chichester: Wiley‐Blackwell; 2013, pp. 619–635. Cannulae are placed in the ascending aorta, superior caval or innominate vein, and right atrium or inferior caval vein. Cardiopulmonary bypass is initiated at 32–34 °C, depending on surgeon preference and without aortic cross‐clamp. Caval venous tourniquets are engaged. If present, native pulmonary blood flow, or a systemic to pulmonary artery shunt, is occluded. The azygous vein is divided. The superior caval vein is clamped above the cavoatrial junction and divided. The stump on the atrial side is oversewn in two layers. A longitudinal arteriotomy is made on the superior aspect of the right pulmonary artery adjacent to the free end of the superior caval vein. Patch augmentation of the pulmonary arteries can be performed if necessary, and the superior caval vein can be spatulated to enlarge the orifice. An end‐to‐side anastomosis is then performed between the superior caval vein and undivided pulmonary artery on that side, with specific details based on surgeon preference. The remainder of the operation is conducted in the usual fashion, as described above for the modified hemi‐Fontan procedure. Modifications of this technique have been described without cardiopulmonary bypass by using a shunt to decompress the superior caval vein [42, 61]. Figure 27.6 The lateral tunnel Fontan procedure. (A) The right atriotomy is performed from the inferior cavoatrial junction to just inferior to the previously placed hemi‐Fontan patch. The atrial septal defect is inspected and enlarged by unroofing the coronary sinus into the left atrium. A lateral tunnel the width of the inferior caval vein is created using a polytetrafluoroethylene (PTFE) patch. A 2.8 or 3.0 mm fenestration is generally placed in the patch. The patch is sutured around the internal orifice of the inferior caval vein. The posterior suture line is carried anterior to the right pulmonary veins and superiorly to the hemi‐Fontan patch. The latter is then removed entirely. The lateral tunnel patch is sutured around the enlarged orifice under the pulmonary arteries. The anterior edge of the patch is then incorporated into the atriotomy closure to complete the lateral tunnel pathway. (B) The completed procedure. Source: Reproduced by permission from Hirsch JC et al. In: Pediatric Cardiac Surgery, 4th ed. Chichester: Wiley‐Blackwell; 2013, pp. 619–635. Table 27.4 Theoretic advantages and disadvantages of each type of total cavopulmonary connection. Note that these advantages are believed to exist by those who advocate each approach, although in most cases there is no well‐supported data in the literature. Therefore, there is no clear consensus on the optimal type and practice patterns vary by surgeon and institution [42, 47, 52, 57–61, 65–72, 74, 77, 78]. BDG/ECC, bidirectional Glenn/extracardiac conduit; BDG/IECC, bidirectional Glenn/intra‐extracardiac conduit; HFP/LT, hemi‐Fontan procedure/lateral tunnel; IVC, inferior caval vein; PA, pulmonary artery. The Kawashima procedure is applied in the setting of patients with left isomerism and interruption of the inferior caval vein, with an azygous or hemiazygous continuation to the superior caval vein. The technique is essentially a BDG procedure that functions as a nearly complete Fontan circulation given this anatomy [62]. Hepatic venous drainage, with the unidentified hepatic factor [63], bypasses the lungs to the common atrium and therefore leads to pulmonary arteriovenous malformations, unless this blood is directed to the lungs during Fontan completion [64]. This stage completes the Fontan circulation by directing the inferior caval venous blood directly to the pulmonary arteries. This is generally performed between 18 and 48 months of age, depending on the type of Fontan. Extracardiac conduit Fontan patients are typically older to allow a larger conduit to be placed [65]. A preoperative catheterization is performed to assess surgical candidacy at most centers. Once the patient is deemed an appropriate candidate as described earlier, the options at this stage include the lateral tunnel Fontan, the extracardiac conduit Fontan, or the intra/extracardiac conduit Fontan procedure. Each of these options can be either fenestrated or nonfenestrated. The lateral tunnel Fontan is the procedure of choice at the University of Michigan, generally performed between 18 and 24 months of age (Table 27.4). It is performed with technical ease following the hemi‐Fontan procedure and with more favorable flow patterns, as already described (Figure 27.4) [59]. No circumferential prosthetic material is used, preserving growth potential and possibly minimizing thrombogenicity [66]. Fenestration is easily performed. Lastly, it maintains percutaneous catheter access to the common atrium, compared to the extracardiac Fontan. The procedure is not utilized in the presence of anomalous pulmonary venous connections, as is frequent in those with right isomerism, when a lateral tunnel could result in obstruction of pulmonary venous return [57]. This technique has been well described in the literature [47, 57, 60, 66
CHAPTER 27
The Functionally Univentricular Heart
Background
Definition
Classification
Cardiac Lesions
Double‐Inlet Ventricles
Mitral Atresia
Tricuspid Atresia
Unbalanced Atrioventricular Septal Defect
“Single Ventricle with Heterotaxy Syndrome”
“Single Ventricle with Total Anomalous Pulmonary Venous Connection” and Other Lesions
Clinical Presentation
Diagnosis
Indications for Surgical Intervention
Historical criteria
Contemporary criteria
Minimum age 4 years
Unobstructed systemic venous return
Sinus rhythm
Low transpulmonary gradient
Vena cavae – normal drainage
Low pulmonary vascular resistance
Right atrium of normal volume
Adequate‐size branch pulmonary arteries
Mean pulmonary artery pressure ≤15 mmHg
Nonrestrictive atrial septal defect
Pulmonary resistance ≤4 units/m2
Adequate atrioventricular valve function
Pulmonary artery‐aortic diameter ratio ≥0.75
No significant systemic outflow obstruction
Normal function of primitive ventricle (ejection fraction ≥0.60)
Adequate systemic ventricular function with low end‐diastolic pressure
No mitral incompetence
No impairing effects of a previous shunt
Historical Surgical Management
Year
Milestone
1945
Blalock–Taussig–Thomas shunt
1952
Pulmonary artery band
1956
Ventricular septation
1958
Classic Glenn operation
1967
Pediatric heart transplantation
1968
Fontan procedure
1972
Bidirectional Glenn procedure
1975
Damus–Kaye–Stansel procedure
1981
Modified Blalock–Taussig–Thomas shunt
1981
Norwood procedure
1987
Intra/extracardiac conduit procedure
1988
Total cavopulmonary connection
1989
Hemi‐Fontan procedure
1989
Baffle fenestration
1990
Extracardiac conduit procedure
Current Surgical Management
First‐Stage Palliation
Inadequate Pulmonary Blood Flow
First stage
Second stage
Third stage
Well‐balanced circulation
No intervention
Hemi‐Fontan procedure
Bidirectional Glenn procedure
Kawashima procedure
Lateral tunnel Fontan
Extracardiac conduit Fontan
Intra/extracardiac conduit Fontan
Inadequate pulmonary blood flow
Systemic to pulmonary artery shunt
Patent arterial duct stent
Excessive pulmonary blood flow
Pulmonary artery band(s)
Intact or restrictive atrial septum
Surgical atrial septectomy
Percutaneous atrial septostomy
Systemic outflow obstruction
Damus–Kaye–Stansel procedure
Norwood procedure
Excessive Pulmonary Blood Flow
Intact or Restrictive Atrial Septum
Systemic Outflow Obstruction
Second‐Stage Palliation
Hemi‐Fontan Procedure
Bidirectional Glenn Procedure
Variable
HFP/LT
BDG/ECC
BDG/IECC
Applicability
Most patients
All patients
All patients
Age at completion Fontan
Usually younger
Usually older
Usually older
Cardiopulmonary bypass required
Yes/Yes
No/No
No/Yes
Aortic cross‐clamp required
Yes/Yes
No/No
No/Yes
Second stage
Technically more complex
Technically easier
Technically easier
Third stage
Technically easiest
Technically easier
Technically easier
Fenestration
More feasible and durable
Less feasible and durable
More feasible and durable
PA status
Routine augmentation
Selective augmentation
Selective augmentation
Efficiency of blood flow
Optimal
Less optimal
Less optimal
Distribution of IVC flow to each lung
Equal
Can be unequal if caval offset
Can be unequal if caval offset
Growth potential
Yes
No
No
Thrombogenicity
Potentially less
Potentially more
Potentially more
Atrial suture lines
More
Less
Intermediate
Atrial exposure to Fontan pressure
More
Less
Less
Arrhythmias
Potentially more
Potentially less
Potentially less
Percutaneous access to common atrium
Maintained
Not maintained
Maintained
Future transplantation
Requires more PA reconstruction
Requires less PA reconstruction
Requires less PA reconstruction
Kawashima Procedure
Third‐Stage Fontan Circulation
Lateral Tunnel Fontan Procedure
Stay updated, free articles. Join our Telegram channel

Full access? Get Clinical Tree
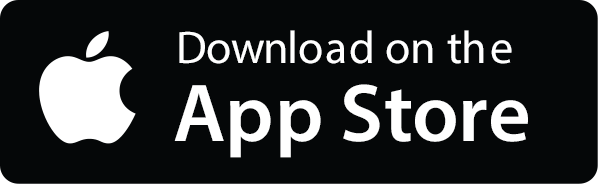
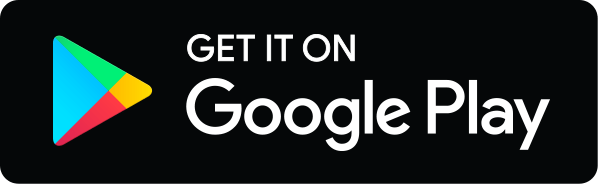