Fig. 8.1
The supply and drainage configuration of the coronary arterial and venous systems [28]. Reproduced with permission of Springer in the format Educational/Instructional Program via Copyright Clearance Center
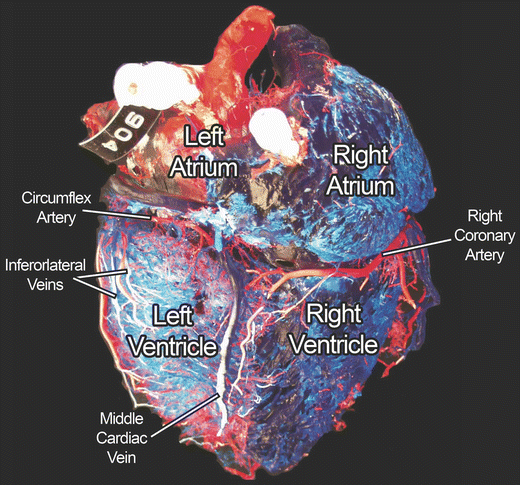
Fig. 8.2
Red and blue plastics were injected into the arterial and venous systems of this heart, respectively. The rest of the myocardial tissue was “corroded” away with digestive enzymes, thus only the extensive coronary system remains. The heart is viewed from the diaphragmatic surface. Figure courtesy of Alexander Hill (Medtronic, Inc.)
Coronary blood flow is critical in supporting cardiac function. If disease states or acute events occur that obstruct coronary flow, consequences are commonly detrimental and sometimes fatal. For example, changes in electrocardiograms can be recorded within beats when there is inadequate blood flow delivered to a given region of the heart. Whenever coronary blood flow falls below what is required to meet metabolic needs, the myocardium is considered ischemic; the pumping capability of the heart is impaired, and there are associated changes in electrical activity (e.g., increased risk of fibrillation). Prolonged ischemia can lead to myocardial infarction, commonly called a heart attack , which can cause irreversible myocardial cell death. Coronary artery disease, which is associated with obstruction of arterial blood flow, is the most common type of heart disease and currently the leading cause of death in the USA in both males and females [4]. For more details on cardiac oxygen and nutrient delivery and its association with ischemia, refer to Chap. 21.
8.2 Coronary Arteries
8.2.1 Coronary Arteries: Anatomical Description
Oxygenated blood is pumped into the aorta from the left ventricle. Located just above the aortic valve are the ostia of the left and right coronary arteries, which are situated in the left and right sinuses of Valsalva, respectively. In general, coronary arteries traverse the surface of the heart and are encased in varying amounts of epicardial fat. However, some branch arteries course directly into the myocardium [4]. Figure 8.3 shows the anatomy and nomenclature of the coronary artery system. Note that there is a high degree of variability in the human heart. (See Coronary Tutorial on the Atlas of Human Cardiac Anatomy; www.vhlab.umn.edu/atlas.)
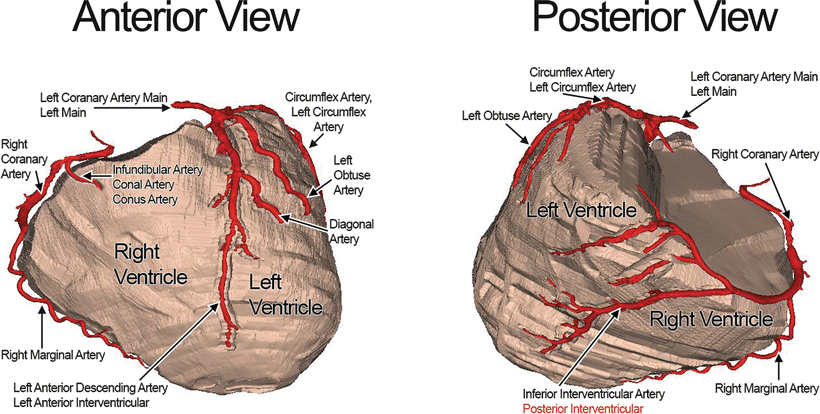
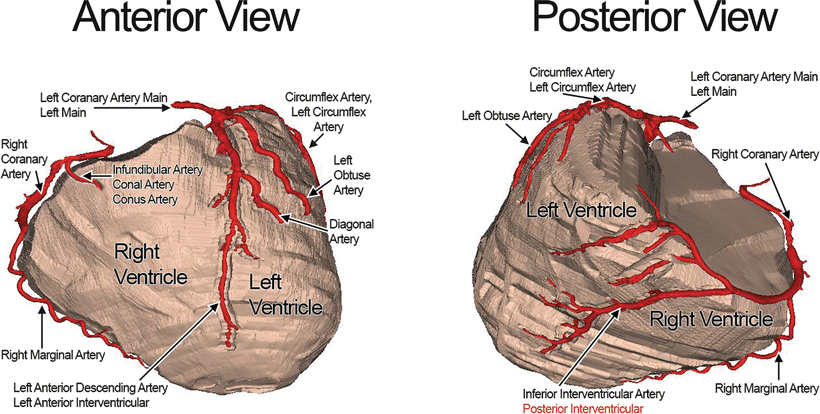
Fig. 8.3
Anterior (left) and posterior (right) views of the 3D reconstructed coronary arteries and ventricular tissue of a perfusion-fixed human heart generated from a contrast-CT scan. The atria have been removed for better visualization of the coronary arteries. Some of the arteries have varying nomenclatures present in the literature. The names highlighted in red are common in the literature, but are considered to be attitudinally incorrect
Traditionally, some of the coronary arterial names were based on the heart oriented in the valentine position. This chapter refers to nomenclatures based on the heart’s orientation in the chest cavity. See Chap. 2 for details on the clinical importance of using attitudinally correct descriptions .
8.2.2 The Left Coronary Artery and Its Branches
The left coronary artery and its branches supply the majority of oxygenated blood to the ventricular myocardium and additionally to the left atrium, left atrial appendage, pulmonary artery, and aortic root [5, 6]. The left coronary artery main, also called the left main for short, typically originates at the left sinus of Valsalva and courses in the left anterior direction. After approximately 1–2 cm, the left main bifurcates into the left anterior descending artery and the circumflex artery, at a location between the left atrial appendage and the pulmonary trunk [7]. Some individuals possess a third artery that branches off the left main called the intermediate artery. This vessel usually supplies the left margin of the heart [7, 8].
8.2.2.1 Left Anterior Descending Artery and Its Branches
The left anterior descending artery, also known as the anterior interventricular artery, originates at the end of the left main at an angle close to 180°. The vessel curves around the pulmonary artery trunk and continues anteriorly down the interventricular septum to the apex of the heart. The left anterior descending artery typically branches into the diagonal arteries, deep septal perforators, and the left descending septal artery. Although there is a high amount of variation in the course of the diagonal arteries, they typically branch off at an angle and supply the free wall of the left ventricle. The deep septal perforators commonly branch at 90° angles into the interventricular septum. These arteries characteristically supply the anterior two-thirds of the septum, and can also have high degrees of variation. Finally, the left descending septal artery is an important vessel that supplies blood to the moderator band and anterior papillary muscle [7].
8.2.2.2 Circumflex Artery and Its Branches
The circumflex artery, also referred to as the left circumflex artery, stems from the left main and courses along the left atrioventricular groove. The circumflex artery gives rise to small arterial branches that supply the aortic root and myocardium near the atrioventricular groove. In patients who are right dominant, the circumflex artery terminates into the left obtuse artery. The left obtuse artery courses down the left margin of the heart towards the apex. In patients who are left dominant (approximately one-tenth of individuals), the circumflex continues along the atrioventricular groove until it terminates at the cardiac crux, where it branches into the artery that supplies the atrioventricular node [9, 10] and the inferior interventricular artery, traditionally known as the posterior interventricular artery [7].
8.2.3 The Right Coronary Artery and Its Branches
The right coronary artery originates in the right sinus of Valsalva and courses along the atrioventricular groove along the epicardial surface adjacent to the tricuspid valve annulus. The infundibular artery, also known as the conal artery, conus artery, and third coronary artery, can also originate directly from the right sinus of Valsalva or as a branch of the right coronary artery. In three-fifths of the population, the next branch of the right coronary artery is the artery to the sinus node [7, 8]. This artery branches off the circumflex artery in the remaining two-fifths of the population [7, 8]. The right marginal artery is typically the largest branch of the right coronary artery. This major artery supplies the free wall of the right ventricle [7, 10]. In most individuals, the right coronary artery becomes the inferior interventricular artery when it reaches the diaphragmatic surface of the heart. Note that the right coronary artery does not typically taper in diameter until it gives rise to the inferior interventricular artery [4]. The inferior interventricular artery runs toward the apex of the left ventricle and supplies the diaphragmatic surface of the heart.
8.2.4 Abnormal Coronary Artery Anatomy
Many anomalies of the coronary artery system are associated with the origin of the major arteries. The most common ostium abnormality happens when the left and/or right coronary artery originates at an opposite sinus, which has been found in 0.03–0.1 % of patients [7, 11]. There have also been reports of the presence of a single coronary artery that supplies the entire heart [7, 12, 13]. There is evidence that both of these abnormalities may increase the risk of sudden death [7, 12–14]. The right coronary artery has also been reported to originate outside of the sinuses of Valsalva including branching from the brachiocephalic artery, the aorta, or the pulmonary trunk [7]. The risk of these anomalies is dependent on the subsequent course of the artery. For example, an artery that courses between the aortic and pulmonary roots has a higher chance of compression [7, 15, 16]. A left coronary artery originating at the pulmonary trunk, also known as Bland-White-Garland Syndrome , is a more rare (1 in 300,000) and more dangerous anomaly [7, 17] that can cause decreased blood flow and typically requires surgical treatment [7, 18, 19]. Figure 8.4 summarizes examples of coronary ostia abnormalities.
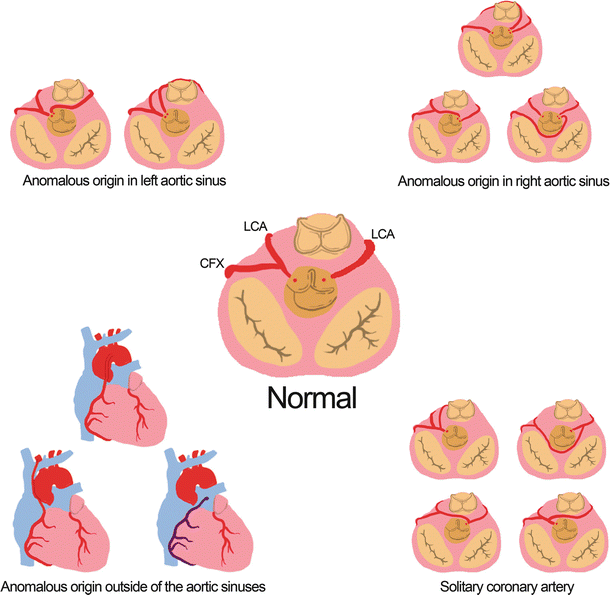
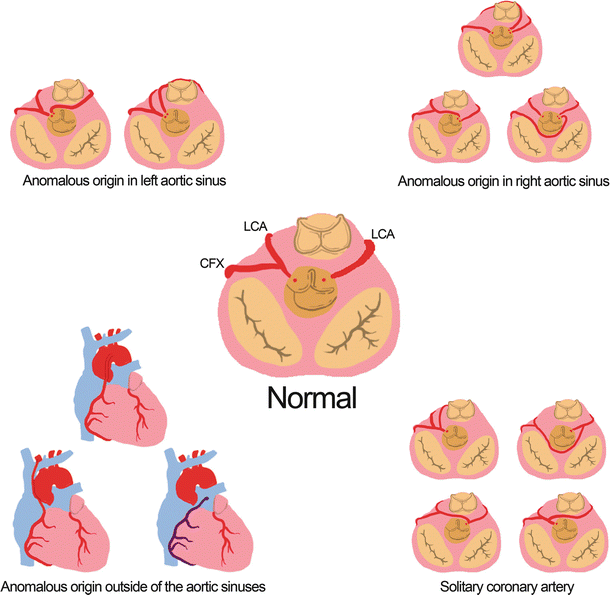
Fig. 8.4
Examples of abnormal coronary arterial origins. The anomalous origins outside the aortic sinuses include the brachiocephalic artery, the ascending aorta, and the pulmonary trunk. LCA left coronary artery, RCA right coronary artery, CFX circumflex artery
Another known anatomical abnormality relative to this vasculature is the presence of coronary arterial fistulas. These are defined as a direct connection between a coronary artery and a cardiac chamber, superior vena cava, coronary sinus, pulmonary artery, or pulmonary vein [7, 19, 20]. The most common fistula sites are located in the right ventricle [7, 21]. It has been reported that these fistulas are benign for approximately half of the patients; the remaining half of patients have reported endocarditis, myocardial ischemia, ruptured aneurysm, and/or heart failure associated with their anomaly [7, 22].
There have also been reports of duplication of the major arteries. The anterior descending artery is the most commonly duplicated artery with an occurrence rate of approximately 1 % [7, 23]. Most coronary artery duplications do not cause clinical complications; however, it is important to be aware of such duplications during surgical interventions.
8.2.5 Coronary Arteries: Disease
Coronary arterial functional anatomy changes throughout a person’s lifespan; in the elderly, coronary arteries typically become more tortuous, their inner diameters expand, and more calcific deposits can be observed [24]. While these changes are common with aging, certain disease states can significantly increase flow restrictions to the point of causing detrimental damage, myocardial ischemia, and/or death. More specifically, coronary artery disease is generally defined as the gradual narrowing of the lumen of the coronary arteries due to atherosclerosis. An example of an artery with calcifications is shown in Fig. 8.5. Atherosclerosis is a condition that involves thickening of the arterial walls via cholesterol and fat deposits that build up along the endoluminal surface of the arteries. With severe disease, these plaques may become calcified, increase in size, and eventually cause significant stenosis; a stenotic vessel has an increased vascular resistance relative to that of healthy vessels. A steady decrease in arterial cross-sectional area can eventually lead to complete blockage of the artery. As a result, oxygen and nutrient supply to the myocardium decreases below the level of demand, i.e., during exercise and during rest (when more extreme). As the disease progresses, the myocardium downstream from the occluded artery becomes ischemic. Eventually, myocardial infarction may occur if the coronary artery disease is not detected and treated in a timely manner. See Chap. 21 for additional descriptions of cardiac ischemia.
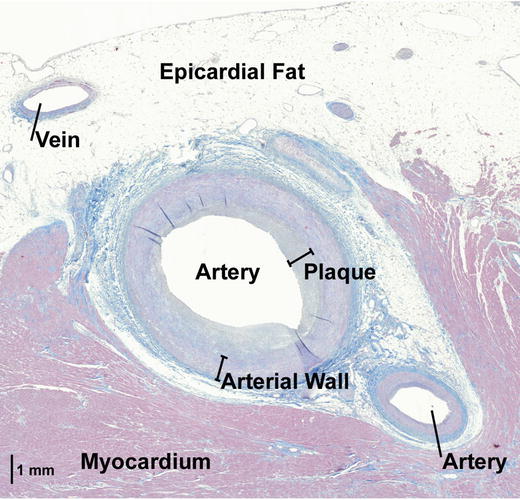
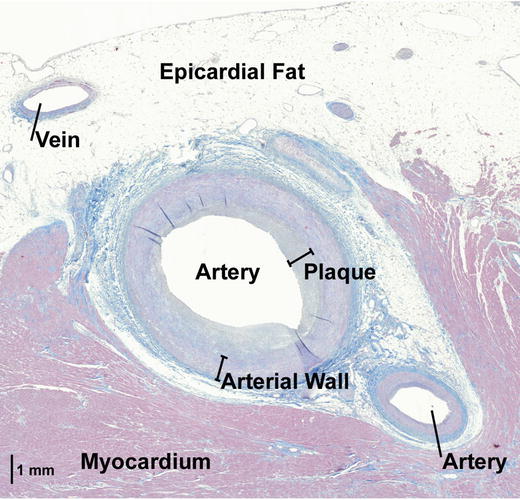
Fig. 8.5
This histologic view of an anterior arterial branch shows a layer of plaque that makes the interior lumen of the artery irregular. Additionally, this figure shows that this particular arterial branch was not completely encased by epicardial fat, but was partially surrounded by myocardium
Myocardial ischemia not only impairs the electrical and mechanical function of the heart, but also commonly results in intense, debilitating chest pain known as angina pectoris. However, anginal pain can often be absent in individuals with coronary artery disease (or in individuals with early disease stages) at rest, but induced during physical exertion or with emotional excitement. Such situations are associated with an increase in sympathetic tone that increases myocardial oxygen consumption (and subsequently ischemia) when blood flow cannot keep up with myocardial metabolic needs. To date, typical treatment for angina resulting from coronary artery disease includes initial pharmacological approaches , such as the administration of: (1) coronary vasodilator drugs (e.g., nitroglycerin ); (2) nitrates to reduce myocardial demand by dilating systemic veins, thus reducing preloads; or (3) β-blockers (e.g., propranolol ).
8.3 Cardiac Capillaries
Capillaries are the smallest blood vessels in the human body and branch numerous times to ensure that every myocyte lies within a short distance (microns) of at least one of these branches. Via diffusion, nutrients and metabolic end products move between the capillary vessels and the surroundings of the myocytes through the interstitial fluid. Subsequent movement of these molecules into a cell is accomplished by both diffusion and mediated transport. Nevertheless, as with all organs, blood flow through the capillaries within the heart can be considered passive and occurs because coronary arterial pressure is higher than venous pressure. Although capillaries are a very important part of the coronary system, their current use for device therapies is relatively nonexistent due to their small diameters (i.e., size of a red blood cell).
8.4 Coronary Veins
Coronary venous flow occurs during diastole and systole, and the coronary venous system drains the myocardium of oxygen-depleted blood.
8.4.1 Coronary Veins: Anatomical Description
The relative size of the coronary venous system dominates the arterial system; there are at least twice as many veins as arteries in human myocardial tissue [25, 26]. In general, veins are considered to be low–resistance conduits to the heart and can alter their capacity to maintain venous pressure [27]. As previously mentioned, the coronary veins are considered to be primarily organized into two subgroups—the greater and smaller cardiac venous system. The greater cardiac venous system is comprised of the coronary sinus and its tributaries, as well as the anterior cardiac veins, atrial veins, and the veins of the ventricular septum [28, 29]. The smaller cardiac venous system, also known as the Thebesian vessels, is comprised of the arterioluminal vessels and venoluminal vessels [28–30].
8.4.1.1 The Greater Cardiac Venous System
The greater cardiac venous system, also referred to as the major cardiac venous system, delivers the majority of deoxygenated blood from the outer layers of the myocardium to the chambers of the heart. These veins are responsible for approximately three-quarters of the myocardial drainage [31]. Similar to some of the major coronary arteries, there are several nomenclatures present in the literature for many of the major veins in the greater cardiac venous system. This chapter refers to the attitudinally correct venous names, although some of these names are currently less common in the literature and in practice. (See Coronary Tutorial on the Atlas of Human Cardiac Anatomy; www.vhlab.umn.edu/atlas.)
Coronary Sinus
The coronary sinus serves as the primary collector of cardiac venous blood [32–34], and is located in the atrioventricular groove on the diaphragmatic surface of the heart [6, 30, 31, 35–38]. Movie 8.1, found in the online supplemental material, shows a representative coronary sinus. The coronary sinus is the largest cardiac vein in terms of diameter. Many major cardiac veins, including the great cardiac vein, the inferior veins of the left ventricle, the oblique vein of the left atrium, the middle cardiac vein, and the small cardiac vein, generally feed into the coronary sinus [6, 28, 30–32, 35, 37, 39–42]. Figure 8.6 summarizes the anatomy and commonly used nomenclatures of the major coronary sinus tributaries. Various landmarks have been described as the location of the coronary sinus origin, including where the oblique vein of the left atrium meets the great cardiac vein and at the valve of Vieussens [28, 31, 32]. The coronary sinus empties directly into the right atrium near the juncture of the interventricular and coronary grooves (also known as the crux cordis area) [31, 39, 43], located on the inferior region of the right atrial septum between the inferior vena cava and tricuspid valve [6, 32, 35, 40, 44]. The atrial orifice can be partially covered by a Thebesian valve as demonstrated in Fig. 8.7, although the anatomy of this valve is highly variable [30–32, 34–36, 38, 39, 42–48].
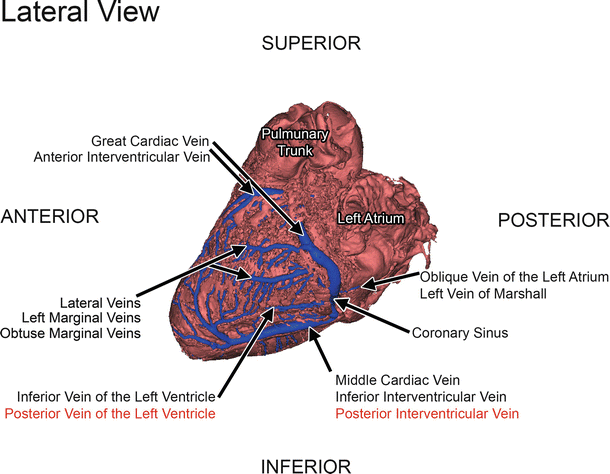
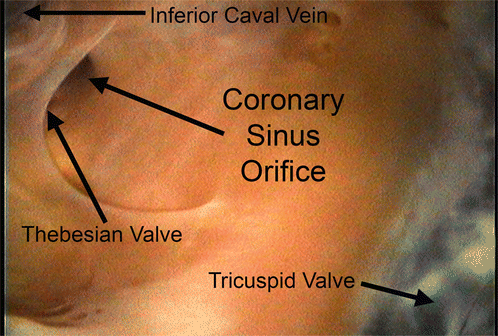
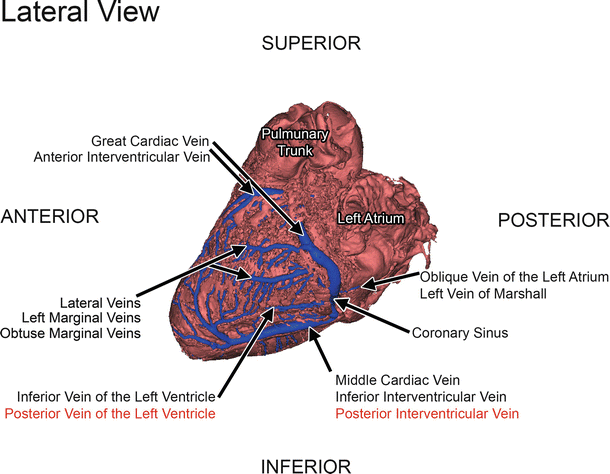
Fig. 8.6
3D reconstruction of the coronary veins and cardiac tissue from a contrast-CT of a perfusion-fixed human heart [28]. This is a left lateral view of the coronary sinus and its major tributaries. Some of these veins have varying nomenclatures present in the literature. The names highlighted in red are common in the literature, but are considered to be attitudinally incorrect. Reproduced with permission of Springer in the format Educational/Instructional Program via Copyright Clearance Center
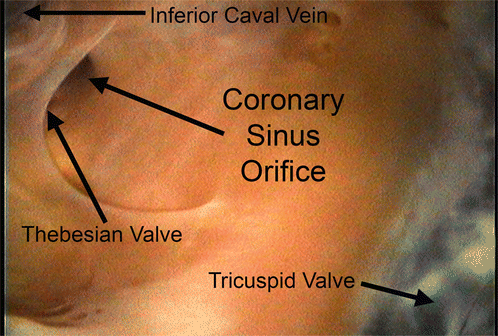
Fig. 8.7
Internal view of the coronary sinus orifice with a Thebesian valve and surrounding right atrial wall from within an isolated functioning human heart [28]. Reproduced with permission of Springer in the format Educational/Instructional Program via Copyright Clearance Center
Anterior Interventricular Vein and Great Cardiac Vein
The anterior interventricular vein courses between the left and right ventricle on the anterior side of the heart. It typically begins around the apex and ends as it reaches the atrioventricular groove. The great cardiac vein, the longest venous vessel of the heart, consists of the anterior interventricular vein and its continuation along the atrioventricular groove [28, 31, 46, 49, 50]. The great cardiac vein is commonly joined by numerous venous contributors from the interventricular septum, anterior aspects of both ventricles, and the apical region [6, 30–32, 37, 49, 51]. As the great cardiac vein courses around the inferior atrioventricular sulcus, it is also joined by venous drainage from the left atrium, including the oblique vein of the left atrium [30]. The lateral veins and left inferior veins from the left ventricle also flow into the great cardiac vein [30, 43]. As the great cardiac vein follows the left atrioventricular groove around the left side of the heart, the great cardiac vein is considered to be in close proximity to the anterolateral commissure of the mitral valve [38]. The great cardiac vein commonly continues until it merges with the coronary sinus described earlier [31, 43, 46, 50]. The great cardiac vein typically enters the coronary sinus at an approximate angle of 180° [49, 52] .
Lateral Veins
The lateral veins, also known as the left marginal veins or the obtuse marginal veins, typically course along the left side of the heart and drain the left ventricular myocardium into the great cardiac vein or coronary sinus [31, 49]. They are commonly located in an inferior position at an obtuse angle of the heart [53] and parallel the course of the left marginal branch of the left coronary artery [49].
Inferior Veins
The inferior veins of the left ventricle, previously known as the posterior veins of the left ventricle, typically originate from the lateral and inferior aspects of the left ventricle and course between the great cardiac vein and middle cardiac vein [31, 41, 51, 52]. The vessels generally drain into the coronary sinus. Similar to the lateral veins, the anatomy of the inferior veins is also highly variable [28] .
Middle Cardiac Vein
The middle cardiac vein, also referred to as the posterior interventricular vein or more correctly, the inferior interventricular vein, is a major coronary vein that typically originates near the apex and usually ascends in or very near to the posterior interventricular sulcus [6, 28, 30, 31, 41, 45, 51, 52, 54]. The middle cardiac vein drains into the coronary sinus or directly into the right atrium [28]. The middle cardiac vein is also one of the most consistently present coronary veins [28].
Small Cardiac Vein
The small cardiac vein, also known as the right cardiac vein [6], commonly drains the inferior and lateral wall of the right ventricle. A small vein in comparison to the previously mentioned veins, the small cardiac vein originates in the inferior part of the right coronary sulcus and courses the base of the right ventricle, paralleling the right coronary artery [31]. This vein typically empties into the coronary sinus, but sometimes drains into the middle cardiac vein or directly into the right atrium. The small cardiac vein is not always present in the human cardiac venous system [31, 55].
Oblique Vein of the Left Atrium
The oblique vein of the left atrium, also referred to as Marshall’s vein since it was first reported by John Marshall, delivers deoxygenated blood from the lateral and inferior regions of the left atrium to the atrioventricular groove [28]. As mentioned earlier, the termination of this vein is an anatomical landmark for the origin of the coronary sinus and the end of the great cardiac vein.
Right Marginal Vein
The right marginal vein, also known as the vein of Galen, typically originates at or is superior to the cardiac apex and then courses along the free wall of the right ventricle towards the right atrium. The right marginal vein has highly variable anatomy [29]. The vessel has been cited to drain into the small cardiac vein, the middle cardiac vein, or the right atrium directly [28, 31, 56, 57].
Anterior Cardiac Veins
The anterior cardiac veins, also known as the minor cardiac veins, the accessory cardiac veins, and the unnamed veins of Vieussens [29, 56], return deoxygenated blood from the right ventricle directly to the right atrium. These vessels typically course along the right margin of the heart [31]. There are usually one to three major anterior cardiac veins present on the human heart [29, 56].
Atrial Veins
The atrial veins deliver deoxygenated blood from the atrial tissues back to the cavities of the atrial chambers. The left atrial veins consist of three categories based on the region of the atrium that they drain—the posterolateral veins, the posterosuperior veins, and the septal veins [29, 31]. The posterolateral veins, which include the oblique vein of the left atrium, course along the left atrial posteroinferior and lateral walls and then drain into the coronary sinus. The posterosuperior veins of the left atrium are present in approximately three-fourths of individuals. These vessels drain the posterosuperior region between the pulmonary veins and empty directly into the left atrium or pulmonary veins [31]. The left atrial septal veins, which can be further categorized into the anteroseptal veins and posteroseptal veins, typically drain directly into the right atrium. Anteroseptal veins have been found in almost all human hearts, while posteroseptal veins have been found in approximately one-third [31]. The right atrial veins are located in the intramural region of the right atrial wall and empty directly into the chamber [28, 29].
8.4.1.2 The Smaller Cardiac Venous System
The veins of the smaller cardiac venous system, also referred to as the Thebesian vessels , minor cardiac venous system, and lesser cardiac venous system, are typically smaller than 0.5 mm in diameter and originate from the endocardial layers of the myocardium [28, 29]. These vessels are responsible for draining approximately one-fourth of the deoxygenated blood used up by myocardium and drain directly into their respective heart chambers [31]. The majority of these small veins are present in the atria, although more Thebesian veins have been found in the right ventricle relative to the left ventricle [28, 58].
The main smaller cardiac veins consist of the arterio-luminal and veno-luminal vessels [31], as displayed in Fig. 8.8. The arterio-luminal vessels originate from coronary arterioles or capillaries and empty directly into their respective heart chamber. Similarly, the veno-luminal vessels originate in coronary capillaries and venules, and then empty into their respective heart chamber. The vessels that drain straight from the capillaries to the heart chamber are also referred to as Thebesian vessels [58, 59].
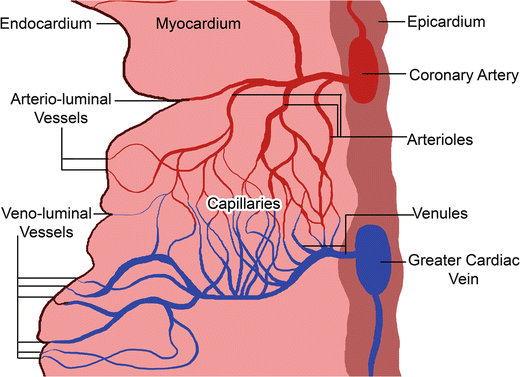
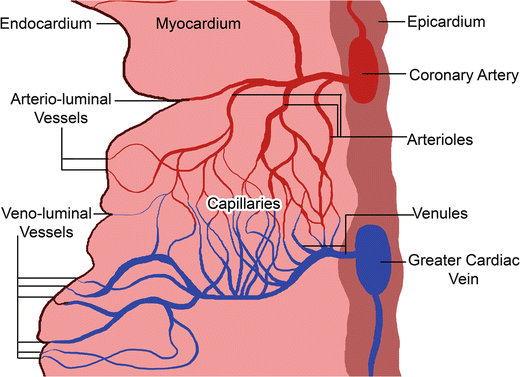
Fig. 8.8
Drawing of the relative coronary systems present within a section through a wall of the heart [28]. The arterio-luminal and veno-luminal vessels of the smaller cardiac system drain directly into the chambers of the heart, Reproduced with permission of Springer in the format Educational/Instructional Program via Copyright Clearance Center
8.4.1.3 Coronary Veins: Valves
The role of venous valves, in general, is to prevent retrograde blood flow. Such valves in the heart are often present at the ostia to the coronary sinus [30–32, 34–36, 39–48], the lateral veins [46, 60], the inferior veins [31], and the middle cardiac vein [30, 31, 43, 61, 62]. A predominant valve can also be identified at the delineation between the coronary sinus and the great cardiac vein [43–49, 52]. Venous valves within the major left ventricular veins have been observed to be comparable in number of valves per vein except in the left marginal vein, which has fewer valves per vein [63]. These valves are most prevalent at the ostia to smaller contributor veins [63]. An example of a valve covering a venous contributor ostia is shown in Fig. 8.9 and in Movie 8.2 in the online supplemental material .
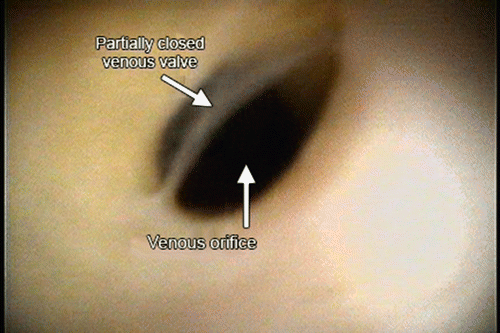
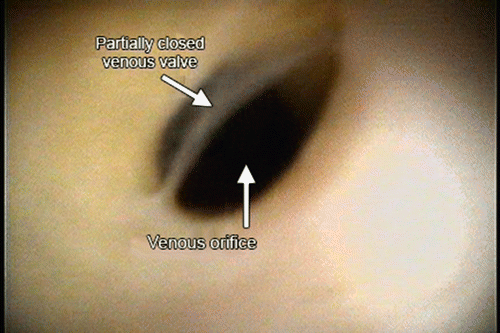
Fig. 8.9
A view of a venous valve located at the orifice of an inferior vein of the left ventricle. The image was obtained using a fiberscope in the coronary sinus of a reanimated human heart. Reproduced with permission of Springer in the format Educational/Instructional Program via Copyright Clearance Center
Abnormal Venous Anatomy
The human coronary venous anatomy is highly variable across patient populations. There have been reports of anatomic abnormalities associated with the cardiac veins. Fortunately, most abnormalities of the venous system do not cause clinical complications. The most common reported coronary venous abnormality is a persistent left superior caval vein, where the veins on the left superior side of the body drain into a left superior caval vein and then directly into the coronary sinus [28]. The persistent left superior caval vein on its own is a non-threatening anatomical variation, but it has been associated with other congenital cardiac malfunctions. Specifically, the abnormality is present in 0.3 % of patients with normal hearts and in 4.5 % of patients with congenital heart disease [64, 65].
Occlusion of the coronary sinus orifice is another reported anomaly of the coronary venous system. This abnormality is extremely rare, but is well reported [66–68]. In these patients, the major cardiac veins are instead drained through the superior vena cava. As a result, therapies requiring access to the coronary sinus ostium must be delivered into the superior vena cava [28].
Similar to the coronary arteries, some of the major cardiac veins have also been reported to be duplicated [29]. For example, the anterior interventricular vein has been observed to be duplicated in 3 % of patients. In these cases, two large veins run parallel ~1 mm apart along the anterior interventricular groove and merge to become one vessel at the atrioventricular groove [49]. The coronary sinus has also been reported to be duplicated with the one coronary sinus in its normal location and the other coronary sinus located intramurally [28, 69] .
8.4.2 Coronary Veins: Disease
Cardiac veins are less likely to develop clinically significant disease states such as atherosclerosis. This is probably due to the inherently lower pressure within the venous portion of coronary circulation and the higher distensibility of the walls of the cardiac veins. When diseases such as heart failure induce myocardial remodeling, the venous system adapts to maintain both blood volume and venous pressure. However, it is generally accepted that the coronary venous system is not affected by atherosclerotic disease, and its “dense meshwork with numerous interconnections” allows blood to return to the heart via numerous pathways [70].
8.5 Microanatomy of Coronary Arteries and Veins
In general, vessels are composed of three layers, which vary in thickness depending on both vessel type and their relative size [71, 72]. These layers are the tunica intima, tunica media, and tunica adventitia, and can be seen in Fig. 8.10. The tunica intima is comprised of endothelium, subendothelial connective tissue, and an internal elastic lamina [29, 72]. The endothelium of vessels entering the heart is continuous with the heart’s endothelium. In veins, the tunica intima and tunica media are less distinct than in arteries, e.g., the venous tunica intima is difficult to distinguish at low power magnification. Concentric layers of smooth muscle, along with collagenic and elastic fibers, make up the tunica media [71, 72]. The tunica media for a vein is much smaller than for an artery of similar size, as can be seen in Fig. 8.11 [72]. The tunica adventitia is composed of longitudinally arranged collagen fibers [72]. In contrast, the tunica adventitia is typically much larger in veins than in arteries.
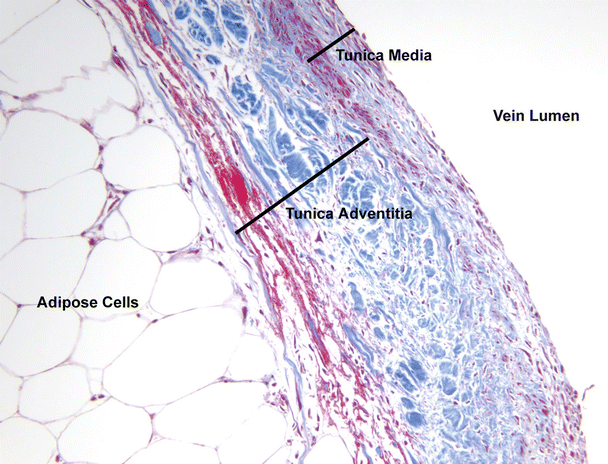
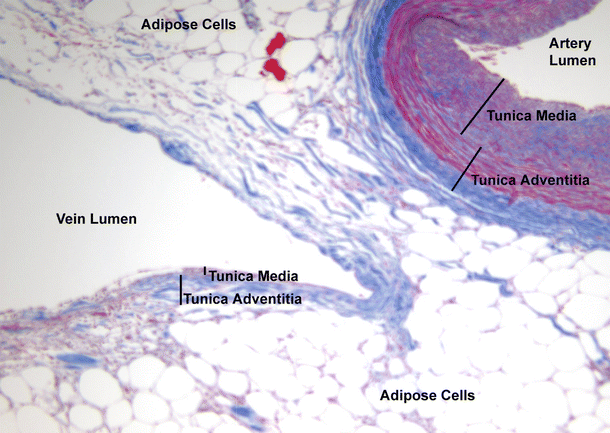
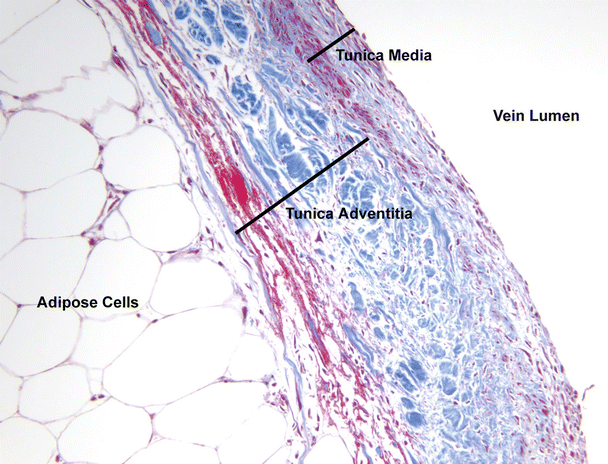
Fig. 8.10
View of coronary venous wall at 20× magnification. To the left are adipose cells and the far right is the vein lumen. The layers of the tunica adventitia and tunica media comprising the venous wall can be seen. The tunica intima cannot be observed at this magnification
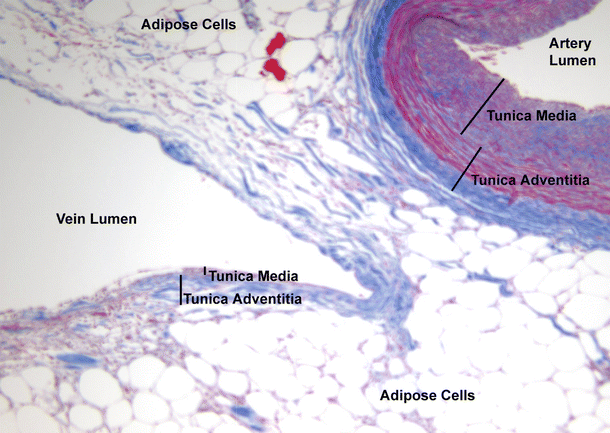
Fig. 8.11
This figure shows the relative differences between coronary arteries and veins . To the left is a coronary vein with a very thin venous wall. Both the tunica media and adventitia can be observed. The tunica intima cannot be observed at this magnification (10×). To the right, the coronary artery is significantly thicker in both the tunica media and adventitia layers. Epicardial fat encases both of these vessels
8.6 Anastomoses and Collaterals
Connections between arteries that supply or veins that drain the same regions, known as anastomoses, provide alternate routes for blood to reach (via arteries) or leave (via veins) the same cardiac region. While numerous anastomoses have been observed on the epicardial surface of the heart, even more are considered present within the myocardium [5]. During fetal development, anastomoses are somewhat prominent; after birth, these channels decrease in both size and functionality [5].
In the general population, anterior interventricular and middle cardiac veins typically have the same origin and termination and follow the same course, while veins between the interventricular sulci are highly variable in number, size, course, and occurrence of anastomoses [31]. In addition, in general, coronary veins have a higher occurrence of anastomoses than coronary arteries [31], resulting in a highly interconnected venous network [6, 26, 70]. More specifically, the apical region of the heart will typically elicit the greatest density of venous anastomoses [66, 73].
Interestingly, in hearts with atherosclerotic damage, small anastomoses develop into larger, more efficient collaterals due to hypertrophic remodeling [5]. Such collaterals may help to protect against ischemia by continuing to allow blood to flow to a given region of the heart when an original arterial route becomes obstructed [39, 73]. Collaterals protect against hypoxia and ischemia by continuing to supply oxygenated blood to a specific region of myocardium [5, 74]. While collaterals increase in size and number with age, whether this increase is related to atherosclerosis is considered unknown [74, 75]. Nevertheless, large and significant collaterals can often be observed near an infarcted area, which may indicate a slow remodeling process [39, 76]. However, in severe stages of coronary artery disease, even extensive collaterals will not allow certain regions of the myocardium to be adequately perfused. To treat coronary artery disease patients, either the arterial branch can be reopened by coronary angioplasty and/or stenting, or a new pathway can be created via coronary artery bypass grafting. If such an obstruction is an acute event, collaterals will not have sufficient time to develop to adequately protect a given heart region from myocardial tissue damage .
8.7 Assessment and Visualization of the Coronary System
Catheterization of the heart is an invasive but commonly employed procedure for visualization of the heart’s coronary arteries, chambers, valves, and/or great vessels. It can also be used to assess: (1) pressures in the heart and blood vessels; (2) function, cardiac output, and diastolic properties of the left ventricle; (3) relative flow of blood through the heart and coronary vessels; (4) regional oxygen content of the blood (e.g., aortic and within the coronary sinus); (5) status of the electrical conduction properties of the heart; and/or (6) septal or valvular defects.
Basic catheterization techniques involve inserting a long, flexible, radio-opaque catheter into a peripheral vein (for right heart catheterization) or a peripheral artery (for the left heart) under fluoroscopy (continuous X-ray observation). Commonly, during this invasive procedure, a radio-opaque contrast medium is injected into a cardiac vessel or chamber. The procedure may specifically be used to visualize the anatomical features of the coronary arteries, coronary veins, aorta, pulmonary blood vessels, and/or ventricles. Such investigations may provide pertinent clinical information about structural abnormalities in blood vessels that may be restricting flow (such as those caused by atherosclerotic plaque), abnormal ventricular blood volumes, inappropriate myocardial wall thickness, and/or altered wall motion. A sample venogram from an isolated heart preparation can be seen in Movie 8.3 in the online supplemental material; the catheter was inserted into the coronary sinus to block off antegrade flow, and then contrast was injected retrograde such that the coronary venous tree was more easily observable. Furthermore, this map of the coronary veins can be used as an aid in the placement of a pacing lead in the coronary veins.
To date, coronary angiography has been the primary visualization modality used clinically. However, multiple contrast injections can potentially be acutely deleterious to patients such as those with compromised cardiac output [77]. More recently, traditional coronary angiography methods have developed. Digital subtraction angiography has been observed to allow examination of venous occlusions and anatomical variants [78]. Rotational angiography has been shown to provide similar visualization of the coronary arteries as traditional methods, but with significantly less contrast volumes that need to be injected and less radiation exposure [79, 80].
Improving the quality of cardiac visualization allows for better planning of access to the coronary system during intervention and, therefore, decreased patient risk [81]. For example, in coronary artery bypass surgery, planning where sutures should be fixed is essential to avoid suturing to a rigid calcified arterial wall [7]. Several visualization modalities have recently been used and developed to enhance visualization of the coronary system. For instance, prior to lead implantation, tissue Doppler imaging can be utilized to identify the target implant region for a transvenous pacing lead [82, 83]. Additionally, noncontact electrical mapping (see Chap. 32) could be used to identify electrically viable target regions [84].
There have also been recent advances in intravascular imaging during interventions in the coronary system. Intravascular ultrasound (IVUS) is a catheterization method that utilizes ultrasound to identify plaques in vivo undetected by traditionally angiography and to guide coronary stent implantation [85, 86]. Also, IVUS has been used to assess coronary arterial wall elasticity, as this parameter has been associated with an increased risk for heart attack [87, 88]. Another current intravascular visualization method is optical coherence tomography (OCT) . OCT generates infrared light and processes the reflections into in vivo images that can be used in the verification of implanted stents [87, 89]. Currently, OCT provides higher image resolution than IVUS, but it has more limited penetration in the vasculature [87, 89]. Photonic spectroscopy via catheterization, including near-infrared spectroscopy (NIRS) and Raman spectroscopy (RS) can also be applied within the vasculature to assess the chemical composition of plaque [87, 90, 91].
< div class='tao-gold-member'>
Only gold members can continue reading. Log In or Register a > to continue
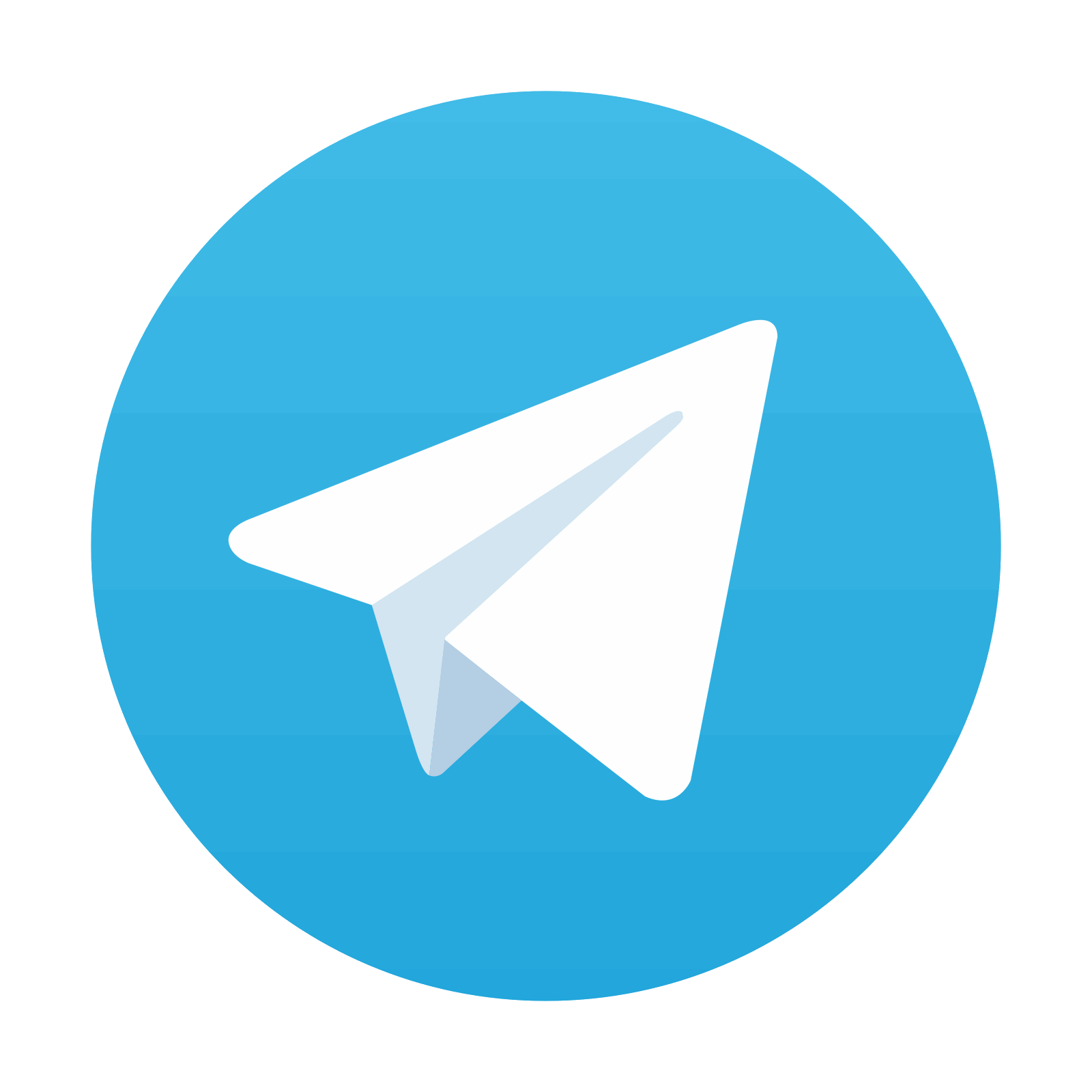
Stay updated, free articles. Join our Telegram channel

Full access? Get Clinical Tree
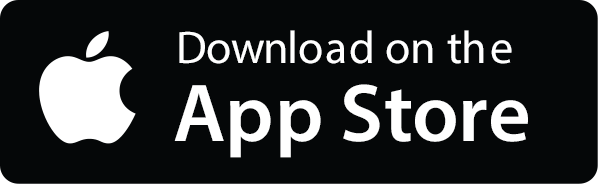
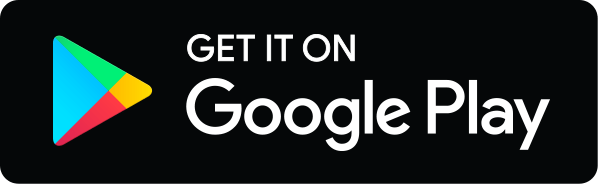