The use of attitudinally correct nomenclature is all the more important with regard to the atrioventricular valves, as current nomenclatures used to describe the leaflets are neither standardized nor attitudinally correct [5]. The tricuspid valve is situated between the right atrium and right ventricle, and is so named because, in the majority of cases, there are three major leaflets or cusps. These are currently referred to as the anterior, posterior, and septal leaflets, and were most likely termed in this manner during extra-corporal examinations of the heart. Figure 1.2 shows an anterior view of a human heart in an attitudinally correct orientation, with the tricuspid annulus shown in orange; the theorized locations of the commissures between the leaflets are shown in red. In order for the “anterior” leaflet to be truly anterior, the tricuspid annulus would need to be orthogonal to the image. However, the true location of the annulus is in an oblique plane as shown in the figure, thus the leaflets would be more correctly termed antero-superior, inferior, and septal. Additionally, the mitral valve normally presents with two leaflets commonly referred to as the anterior and posterior. However, the leaflets are not strictly anterior or posterior, and would be better described as antero-superior and postero-inferior or aortic and mural.
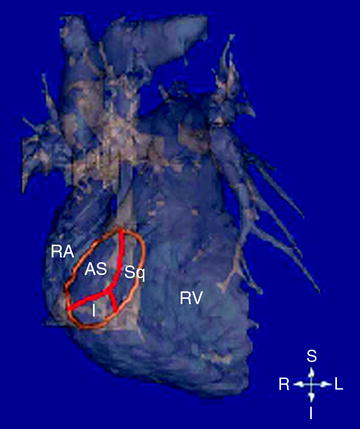
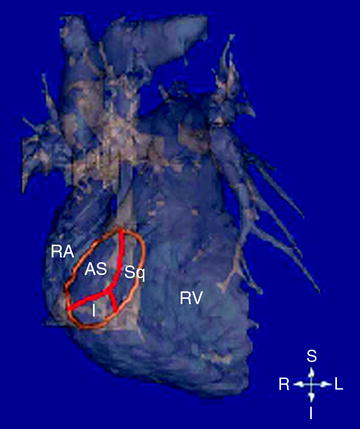
Fig. 1.2
Volumetric reconstruction of a human heart created from magnetic resonance imaging (MRI). One can observe the anterior surfaces of both the right ventricle and atrium. The tricuspid annulus is highlighted in orange, and was superimposed onto the MRI image. AS antero-superior; I inferior; L left; R right; RA right atrium; RV right ventricle; S superior; Sq septal (Iaizzo PA (ed) The handbook of cardiac anatomy, physiology, and devices, 2nd edn., 2009, Humana Press, Totowa NJ)
Importantly, note that we will use this attitudinally correct anatomical nomenclature for the remainder of the chapter.
1.3 The Cardiac Skeleton
Before describing the specific anatomies of the atrioventricular valve, it is important to understand the anatomical cardiac framework that holds these valves in position at their respective atrioventricular junctions, and thus consequently the relationships of each valve to the others [6]. Figure 1.3 shows an anatomical plate of a human heart with the atria and great arteries removed highlighting the close proximity of all four cardiac valves to each other. Traditionally, the four valves of the heart have been described as being supported by a fibrous framework or cardiac skeleton made of dense connective tissue passing transversely through the base of the heart between the atria and the ventricles. However, current interpretations of the extent of the skeleton are often greatly exaggerated. As described by Wilcox, Cooke and Anderson [6] and by Bateman et al. [7] the strongest part of the skeleton is the area of fibrous continuity between the leaflets of the mitral and aortic valves. This fibrous strap, thickened at both ends by the fibrous trigones, anchors the aortic-mitral valvar unit within the base of the left ventricle (Fig. 1.4). The coronet-like support of the aortic valvar leaflets extends antero-cranially from the region of fibrous continuity, and is often considered to represent an aortic valvar annulus, but there are no anatomical structures supporting the semilunar hinges of the aortic valvar leaflets (Fig. 1.5) [7]. The right fibrous trigone is itself continuous with the membranous part of the ventricular septum, and is an integral part of the aortic coronet (Fig. 1.4). The trigone and membranous septum together are usually described as the central fibrous body. The so-called annular components of the atrioventricular valves then extend inferiorly and posteriorly from the central fibrous body and the left fibrous trigone, respectively (Fig. 1.5) [7].
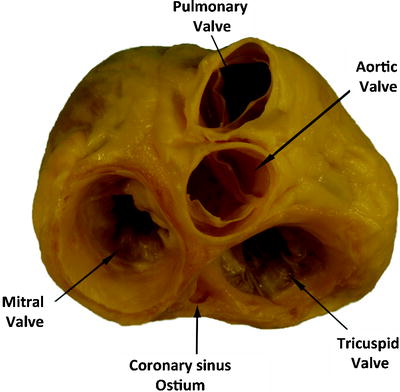
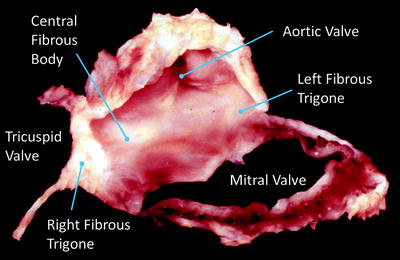
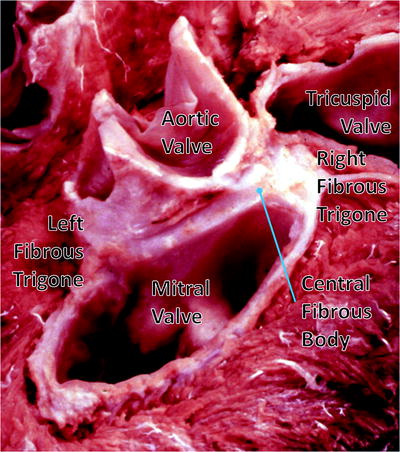
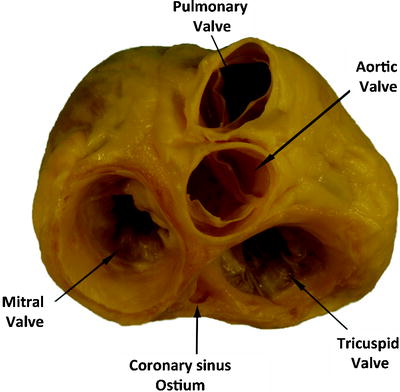
Fig. 1.3
An anatomical plate of a human heart with the atria and great arteries removed showing the relationship between the four valves at the base of the heart. Note the fibrous connection between the leaflets of the mitral valve creating a double orifice valve
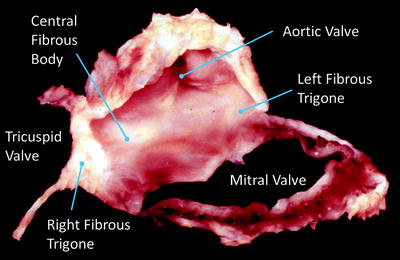
Fig. 1.4
Dissection of the cardiac skeleton showing the aortic valve (center), the mitral valve annulus (below right), and the fibrous sections of the tricuspid valve (to the left). The original image for this figure was kindly provided by Professor Robert H. Anderson. It was initially published in “Cardiac Anatomy” [55], and has been modified for this review. Professor Anderson retains the copyright of the initial image
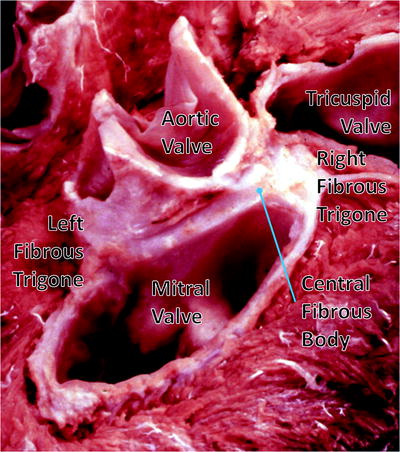
Fig. 1.5
Dissection of the cardiac skeleton with the atria and great vessels removed showing the coronet shape of the aortic annulus and the mitral valve. The original image for this figure was kindly provided by Professor Robert H. Anderson. It was initially published in “Cardiac Anatomy” [55], and has been modified for this review. Professor Anderson retains the copyright of the initial image
It is the exception rather than the rule, however, for these fibrous cords to extend throughout the full circumferences of the left and right atrioventricular junctions. The annulus, as such, is better formed in the mitral as opposed to the tricuspid junction. Even in the mitral junction, nonetheless, it is common to find segments of the valvar leaflets hinged from the fibro-adipose tissue of the atrioventricular junction, rather than from a firm fibrous annulus [8]. In the tricuspid junction, the valvar leaflets are normally hinged from fibro-adipose tissue [9]. It is also the fibro-adipose tissues of the junctions that provide the greatest part of the insulation between the atrial and ventricular muscular masses, with the atrioventricular bundle of the conduction system being the only structure in the normal heart that crosses the insulating plane. The bundle penetrates through the atrioventricular component of the membranous septum. The annuluses, as part of the atrioventricular junctions and rarely being complete fibrous rings, are highly dynamic and change dramatically in shape and size throughout the cardiac cycle from systole to diastole [6, 7].
1.4 The Atrioventricular Valves
In the most basic anatomical sense, the atrioventricular valves are made up of three main components, as seen in Fig. 1.8:
Valve leaflets attached to the respective annulus
Tendinous cords attaching the leaflets to the ventricular myocardium
Papillary muscles providing the anchoring points for the tendinous cords to the ventricular wall
The leaflets of the atrioventricular valves can be thought as forming a skirt that hangs from the annulus, and are divided into a series of sections that constitute the distinct leaflets of each valve. Due to the extent of variations between individuals with regard to leaflet morphologies, there has been much debate relative to nomenclature on the number of leaflets of both the mitral and tricuspid valves [10–12]. Traditionally, the division of the leaflets has been determined by the presence of commissures which can be described as the peripheral attachment of a break in the skirt [6].
The leaflets themselves are attached to the ventricles via the sub-valvar apparatus of each valve. In general, each apparatus consists of both the tendinous cords and the papillary muscle complexes of each valve. The tendinous cords are usually categorized by (1) those that support the free edges of the valves, (2) those that support the rough zones (the region between the free edge and each annulus), and/or (3) those that attach to the leaflets near to the annulus. Typically, the cords supporting the free edges of the leaflets are known as fan cords due to the presence of multiple fenestrations. Those that attach to the rough zone of the leaflets are distinguished by their larger size and are commonly defined as strut cords. Finally, those that attach near the annulus are known as basal cords. The strut cords are of specific importance as they bear the highest mechanical loads during systole [13]. Furthermore, the number and distribution of the tendinous cords across a given valve are critical to its function; it is well documented that dysfunction of these structures can lead to prolapse of the valves [2, 14, 15]. In general, the cords attach to the heads of the papillary muscles which themselves play an important role in the function of each valve by contracting during systole to cushion the valve closure.
1.4.1 Atrioventricular Valve Function
During systole, when the ventricles are contracting, the sub-valvar apparatus of each valve prevents the leaflets from prolapsing into the atria and additionally aids in ventricular ejection by effectively drawing the apex of the ventricle toward the basal ring. Additionally, it has also been shown that the sub-valvar apparatus plays a crucial role during diastole, while the ventricle is filling, by moderating wall tensions and improving the efficiencies of the ventricular myocardium [16, 17]. During systole in normal/healthy cardiac function, the valve leaflets, which bulge toward the atrium, can be considered to stay pressed together throughout the contraction and therefore do not prolapse. During diastole, when the ventricles are relaxing and the chambers are filling through the open atrioventricular valves, eddy currents that form behind the leaflets and tension in the sub-valvar apparatus keep the leaflets close together.
Figures. 1.6 and 1.7 show image sequences obtained employing Visible Heart® methodologies, as described in Chap. 15. These sequences display the normal cardiac function of the mitral and tricuspid valves respectively [18, 19]; the images were obtained from the atria (above the valve) and from the ventricular apexes (below the valve).
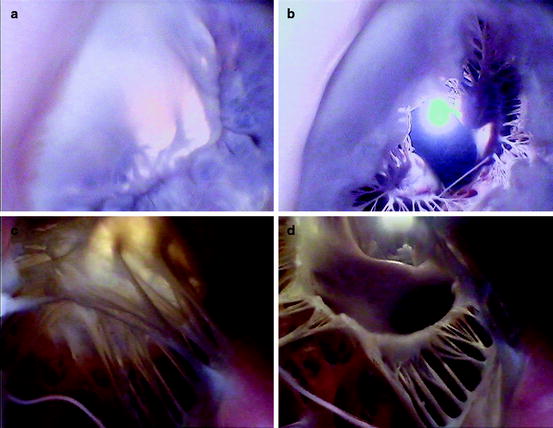
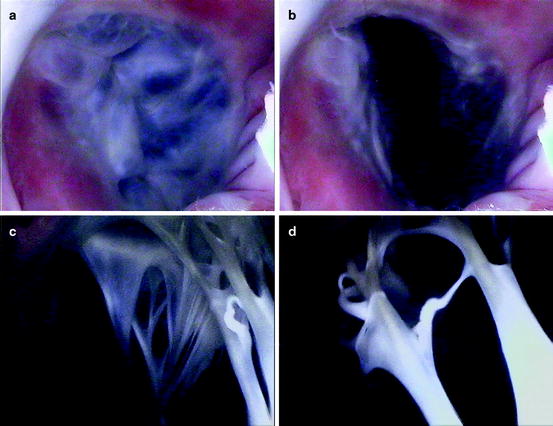
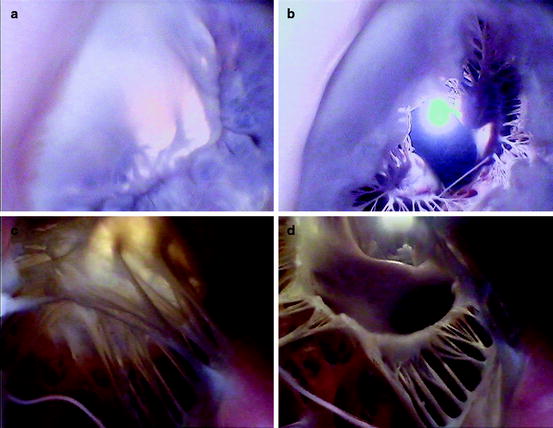
Fig. 1.6
Internal videoscopic images of the mitral valve from above (a, b) and below (c, d) during systole (a, c) and diastole (b, d) obtained employing Visible Heart® methodologies
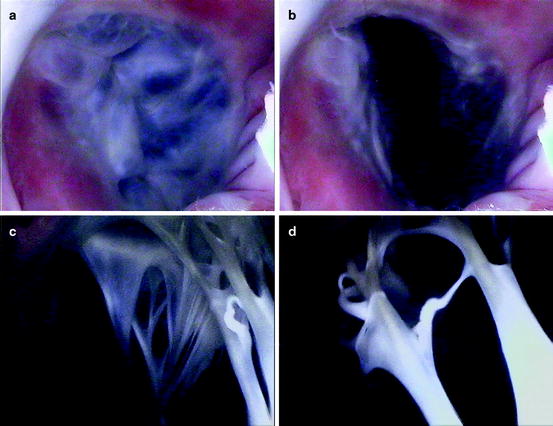
Fig. 1.7
Internal videoscopic images of the tricuspid valve from above (a, b) and below (c, d) during systole (a, c) and diastole (b, d) obtained employing Visible Heart® methodologies
Dysfunction of the atrioventricular valves is usually characterized by one of two symptoms (1) failure of a given valve to successfully close, or (2) failure of a valve to successfully open. Dysfunction of the valves during systole (i.e., failures of the valve to successfully close) is known as valvar incompetence and results in the regurgitation of blood back in a retrograde direction though the atrioventricular junction. Such dysfunction results in a decrease in cardiac output and also increases the pressure within the atria during systole (potentially causing atrial dilation and/or eventually atrial fibrillation). Dysfunction of the valves in diastole (failures of the valve to fully open and allow blood to fill the expanding ventricles) is termed stenosis. This decrease in effective orifice area of the open valve is often due to stiffening or calcification of the valve leaflets. Pathologies of the atrioventricular valves are described in detail in Chap. 6.
1.4.2 Valve Histologies
Interestingly, the atrioventricular valves share very similar leaflet histologies. The atrial sides of the leaflets consist of spongy tissue (lamina spongiosa) comprised of fibrocytes, histiocytes, and collagen fibers [20]. It is these collagen fibers that are considered to supply the mechanical strength required of the atrioventricular valves. The ventricular sides consist of fibrous tissue (lamina fibrosa) and both these layers are surrounded by endothelial cells. Additionally, the valve leaflets have been shown to incorporate both primary sensory and autonomic innervation. In general, it is considered that the anterior leaflet of the mitral valve has twice the innervation of the posterior leaflet [21]. These nerves are typically situated in the lamina spongiosa and extend over the proximal and medial portions of the leaflet [20]. Fibroblasts [22], smooth muscle cells [23, 24], and myocardial cells [25] are also commonly located within the leaflet tissue.
Cells within the leaflets have been shown to elicit two types of contractile activities (1) a brief contraction or twitch at the beginning of each heart beat (reflecting contraction of myocytes in the leaflet in communication with and excited by atrial muscle) which has relaxed by mid-systole and whose contractile activity is eliminated with β-receptor blockade, and (2) sustained tonic contractions (or tone) during isovolumic relaxation, which has been shown to be insensitive to β-blockade, but doubled by stimulation of the neurally rich region of aortic-mitral continuity [26]. These contractile activities within the leaflets are hypothesized to aid in the maintenance of anterior leaflet shape. This, in turn, could help prevent mechanical shock to the leaflets upon valve closure and also aid in optimizing the leaflet shape for funneling blood into the left ventricular outflow tract [26].
The tendinous cords are composed of a collagen core, surrounded by elastin fibers interwoven in layers of loose collagen. Similar to the valve leaflets they also have an outer layer of endothelial cells, but it is the collagen cores that support the greatest degree of mechanical load during systole and allow for the wavy configuration during diastole. The elastin fibers are normally arranged in parallel fashion relative to the collagen fibers and as the cords are stretched during systole, the elastin fibers are also stretched, straightening the collagen. It is hypothesized that it is this composite configuration of elastin and collagen that provides a smooth mechanism for the transmission of chordal forces from the leaflets to the papillary muscles. Additionally, during diastole, the stretched elastin fibers likely help to restore the wavy configurations of the primary collagen cores. The relative amount of collagen and elastin within the given tendinous cords varies according to their relative types, as does the relative amount of contained DNA and their degree of vascularization. Normally the vascularization of the tendinous cords is located between their collagen cores and the elastin fibers, and is further considered to supply nutrients to the leaflets. It has been reported that a higher DNA content within both the anterior and posterior marginal tendinous cords relates to inherently higher rates of collagen syntheses in order to prevent mechanical deterioration compared with other types of tendinous cords [14].
The papillary muscles themselves can be considered part of the ventricular myocardium and hence are composed of aggregated myocytes. The cells exhibit complex junctions, called intercalated discs, allowing multiple cells to form long cellular networks. Within the papillary muscles, these muscle fibers run parallel to each other along the length of the muscle to increase contractile force and efficiency. The papillary muscles are extensively innervated and have complex vascular systems in order to maintain coordinated contractions with the continuum of the ventricular myocardium [27].
1.5 The Mitral Valve
The left atrioventricular valve, or mitral valve, named by Andreas Vesalius due to its structural resemblance to the cardinal’s mitre, is situated in the left atrioventricular junction and modulates the flow of blood between the left atrium and ventricle. Commonly, the valve consists of an annulus, two leaflets, two papillary muscle complexes, and two sets of tendinous cords, as seen in Fig. 1.8.
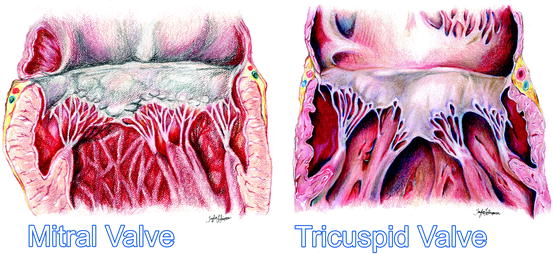
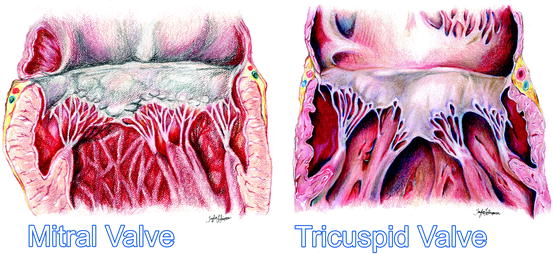
Fig. 1.8
An artist’s rendition of the healthy mitral and tricuspid valves clearly showing the annuluses, leaflets, tendinous cords, and papillary muscles
In 1976 Carpentier described the mitral valve as consisting of two apposing leaflets—a posterior leaflet with three scallops and an anterior leaflet with one scallop. Each region of the leaflets is designated an alphanumeric label to distinguish it from the rest of the valve (Fig. 1.9) [28]. However, when one considers these structures relative to the landmarks of the body (i.e., in an attitudinally correct nomenclature), the leaflets are located in postero-inferior and antero-superior positions. Confusion regarding positional nomenclature can be avoided when adopting the more traditional approach suggested by Vesalius for distinguishing between the leaflets, and recognizing that they are aortic and mural in their locations [29]. The junctions of the two leaflets are commonly referred to as the anterolateral and the posteromedial commissures, however these are more accurately described as superior and inferior. The line of apposition of the leaflets during valve closure is known as the fibrous ridge.
The simplicity and practicality of Carpentier’s anatomic description of the mitral leaflets led to its widespread use after being introduced in 1976 [28]; yet, while this description defines a majority of mitral valve anatomies, there can be wide variability in both the number of scallops within each leaflet and their relative positions [30].
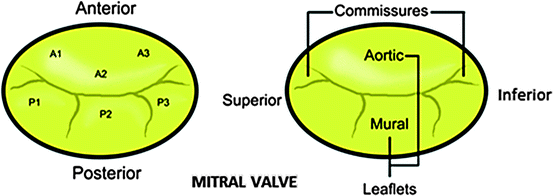
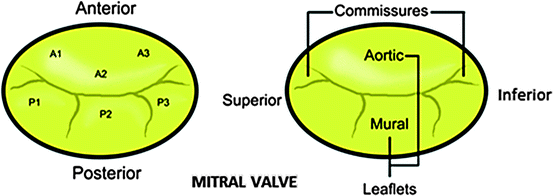
Fig. 1.9
Nomenclature of the mitral valve leaflets: the left diagram shows Carpentier’s 1976 nomenclature the right depicts the modern attitudinally correct nomenclatures
In general, the aortic leaflet is found to be attached to approximately one-third of the annulus circumference and is supported by the aorto-mitral fibrous continuity, which terminates in the left and right fibrous trigones (Fig. 1.10). The mural leaflet is attached to the remaining two-thirds of the annulus and also to the fibrous extensions that continue from the trigones around the mitral valve. However, the lengths of these extensions can be highly variable. Furthermore, a fibrous-fatty tissue surrounds the valve in areas where the cardiac skeleton is not present. The mitral annulus is a highly dynamic feature of the heart, changing dramatically in shape and size throughout the cardiac cycle. It is often described as being saddle-shaped with the highest point of the saddle, the saddlehorn, being found at the midpoint of the area of aortic-to-mitral valvar continuity [31] (Figs. 1.6 and 1.11). Both Delgado and Veronisi and their colleagues reported a series of annular dimensions that were recorded using echocardiography in healthy patients; these data are summarized in Table 1.1 [32–34].
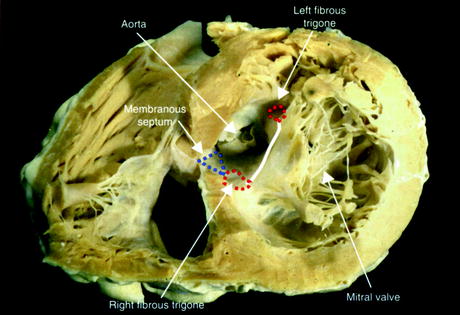
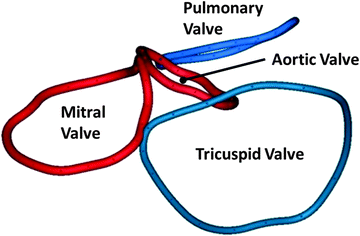
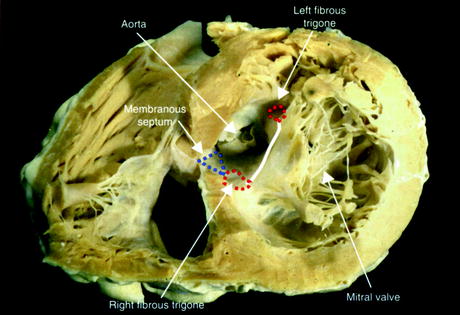
Fig. 1.10
Trigones and the aorto-mitral fibrous continuity within a sectioned human heart. In this case, the cardiac skeleton is being viewed from the apex of the heart. The anterior cardiac surface appears in the upper part of this image, whereas the posterior surface is below [29]
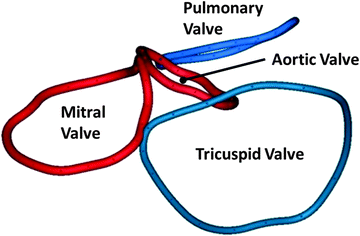
Fig. 1.11
3D reconstruction of the annuluses of the mitral (red) and tricuspid (blue) valves in Mimics ® (Materialise, Leuven, Belgium) from CT scans of a human heart in vivo. The image also shows the location of the virtual rings formed by joining together the basal attachments of the leaflets of the aortic and pulmonary valves
Measured anatomical feature | Data | Sample size |
---|---|---|
9.12 ± 1.71 cm2 | N = 84 | |
9.49 ± 1.25 cm2 | N = 13 | |
2.38 ± 0.40 cm | N = 84 | |
3.00 ± 0.45 cm | N = 13 | |
![]() Stay updated, free articles. Join our Telegram channel![]() Full access? Get Clinical Tree![]() ![]() ![]() |