Matthew Liava’a1 and Yves d’Udekem1,2,3 1Cardiac Surgery Department, Royal Children’s Hospital, Melbourne, Australia 2Murdoch Children’s Research Institute, Melbourne, Australia 3Department of Paediatrics, Faculty of Medicine, The University of Melbourne, Melbourne, Australia This group of lesions is frequently described as “pulmonary atresia with ventricular septal defect.” This is not incorrect; however, it is more descriptive to consider them as tetralogy of Fallot with pulmonary atresia (TOF/PA). This is because patients with diverse phenotypic arrangements are also correctly described as having pulmonary atresia with ventricular septal defect (VSD). Those with transposition, for example, or those with congenitally corrected transposition, or those with isomeric atrial appendages, or double‐inlet ventricle, or atrioventricular valvar atresia, can all have pulmonary atresia in the presence of deficient ventricular septation. In these latter settings, the intrapericardial pulmonary arteries (PAs) are almost always confluent, and are fed by a patent arterial duct. It is only the group of patients with the intracardiac anatomy of tetralogy of Fallot, but with atresia of the subpulmonary outflow tract, in whom the pulmonary arterial supply is derived exclusively, and with any frequency, from congenital systemic‐to‐pulmonary collateral arteries [1]. In this group of patients, as in tetralogy with pulmonary stenosis, the aortic root is positioned posteriorly within the cardiac base relative to the atretic pulmonary outflow tract and is usually overriding the crest of the ventricular septum. As in tetralogy with pulmonary stenosis, the aorta can sometimes arise exclusively from the right ventricle (RV), but then with fibrous continuity between the leaflets of the aortic and mitral valves in the roof of the interventricular communication. The VSD, again as in tetralogy, is usually bordered posteroinferiorly by fibrous continuity between the leaflets of the aortic and tricuspid valves, thus making it perimembranous. This means that the atrioventricular conduction axis will be at potential risk in the posteroinferior margin of the defect (Figure 22.1A). In a proportion of cases, the caudal limb of the septal band will fuse with the ventriculo‐infundibular fold, producing a muscular posteroinferior rim that will protect the conduction axis (Figure 22.1B). The blockage to the subpulmonary outlet is usually muscular, either at the entrance to, or at the distal end of, the subpulmonary infundibulum. On occasion the atresia can be produced by an imperforate pulmonary valve, which can block a narrowed infundibulum, or be in continuity with the overriding aortic valve. In the latter instance, the septal defect itself will extend potentially to become doubly committed (Figure 22.2). In some patients, the pulmonary arterial supply is derived exclusively from systemic‐to‐pulmonary collateral arteries, in the absence of intrapericardial PAs. In this setting, had the intrapericardial PAs developed, it is impossible to determine whether they would have arisen from the heart itself, making the trunk present an aorta, or from a potentially common arterial trunk. The arterial trunk exiting from the heart, therefore, is logically described as being solitary, but to all intents and purposes it is the aorta. The key feature of the patients grouped together as having tetralogy with pulmonary atresia is the morphology of the PAs, and the origin of their arterial supply [2, 3]. The pulmonary trunk will always be present when the pulmonary valve is imperforate. Even in this setting, the trunk itself may supply only one PA, the other either having no connection with the pulmonary trunk, or else being completely absent. In most cases, the pulmonary trunk itself is atretic; nonetheless, it is usual to find an intrapericardial confluence of the PAs, even in the presence of congenital systemic‐to‐pulmonary collateral arteries. It forms the characteristic “seagull” identified in angiographic studies and it can vary markedly in size depending on its source of arterial supply. The right and left PAs, when present, may not be confluent, but one of them usually retains its connection to the remnant of the pulmonary trunk. It is possible to find nonconfluent PAs in the absence of a remnant of the pulmonary trunk. Each can then be supplied by one of the bilateral arterial ducts, or one lung can be supplied by systemic‐to‐pulmonary collateral arteries, with the other fed by a duct through the persisting extrapericardial PA. In some patients, however, there is absence of the entire intrapericardial arterial tree, with pulmonary arterial supply depending exclusively on congenital systemic‐to‐pulmonary collateral arteries. Figure 22.1 (A) Intracardiac anatomy – perimembranous defect. (B) Muscular inferior rim. Courtesy of Professor Robert Anderson. Figure 22.2 Imperforate pulmonary valve and doubly committed defect. Courtesy of Professor Robert Anderson. It is the presence of the collateral arteries that is the key feature of tetralogy with pulmonary atresia. Irrespective of the source of arterial supply, the final common pathway is the capillary network supplying the air sacks of the lungs. The capillaries are themselves connected to an intrapulmonary plexus of arteries, which ramifies within the bronchopulmonary segments. Different parts of the plexus can be supplied with blood from different systemic sources. When all the intrapulmonary arteries are in continuity with confluent intrapericardial PAs, there is usually uniform supply to all the segments of both lungs, thus producing a unifocal arrangement. It is usually the persistently patent arterial duct that provides such unifocal supply (Figure 22.3). In rare cases, however, the confluent PAs can be fed in unifocal fashion through an aortopulmonary window, or through a coronary arterial fistula. In some of the patients with seemingly ductal supply, the feeding vessel is found more proximally than expected. This unusual finding can then be explained on the basis of persistence of an artery derived from the fifth aortic arch, although the existence of this enigmatic vessel remains contentious. It is the finding of intraparenchymal PAs fed from multiple sources, the arrangement described as multifocal supply, that creates the clinical complexity in tetralogy with pulmonary atresia. These multiple vessels are the congenital systemic‐to‐pulmonary collateral arteries (Figure 22.4). It is exceedingly rare to find such arteries in a lung that also receives pulmonary arterial supply via the persistently patent arterial duct. It is reasonable to presume, therefore, that the duct will be absent when a lung is supplied by systemic‐to‐pulmonary collateral arteries. The systemic‐to‐pulmonary collateral arteries themselves, however, do usually coexist with confluent intrapericardial PAs (Figure 22.4). It is rare for confluent PAs in this setting to supply all the bronchopulmonary segments in unifocal fashion. In most patients, the different arteries supply different segments of the two lungs. The individual collateral arteries, which typically number from two to six and arise from the descending aorta, the brachiocephalic arteries, or rarely from the coronary arteries, then feed individual intrapulmonary segments, or groups of segments. They usually do communicate with the confluent intrapericardial pulmonary arterial tree. It is then necessary to determine the proportions of each lung supplied by the various pathways, taking note that, in the absence of the intrapericardial PAs, systemic‐to‐pulmonary arteries will exclusively feed the entirety of both lungs. Figure 22.3 Unifocal supply through patent arterial duct. Courtesy of Professor Robert Anderson. Figure 22.4 Multifocal supply showing collateral arteries coexisting with intrapericardial arteries. pulm., pulmonary. Courtesy of Professor Robert Anderson. The developmental relationship of the congenital collateral arteries to the bronchial arteries has yet to be fully established. Some have no independent course within the lung parenchyma, extending directly as simple conduits from a systemic artery to the origin of the intraparenchymal PAs at or near the hilum. Other collateral arteries extend into the lung along the bronchial tree, branching in the pattern of a bronchial artery, and also supplying the bronchial wall. Their origin as bronchial arteries, therefore, cannot be excluded, but nor can it positively be proved [3, 4]. Overall, although potentially complex, the pulmonary arterial supply in the setting of tetralogy with pulmonary atresia can be simplified by recognition of three major patterns. In the first, and most favorable, arrangement a persistently patent arterial duct supplies confluent right and left PAs. In the second pattern, there are confluent intrapericardial PAs, but they will be supplied by systemic‐to‐pulmonary collateral arteries in multifocal fashion. The ultimate supply is via the collateral arteries, but with potential anastomoses with the intrapericardial network at hilar, lobar, or segmental levels (Figure 22.5). The third pattern is encountered when there is absence of the intrapericardial PAs, with all the bronchopulmonary segments then supplied by multiple systemic‐to‐pulmonary collateral arteries. The key to complete clinical diagnosis in the setting of congenital arteries, therefore, is to establish the course of each congenital collateral artery, to establish whether it runs directly into the lung or makes connections with intrapericardial PAs, and to identify with precision the sites of these anastomoses. Figure 22.5 Sites of anastomosis between systemic and intrapericardial pulmonary arteries. Courtesy of Professor Robert Anderson. Only 1–2% of children with congenital heart disease will have TOF/PA [5]. Approximately 20% of patients with tetralogy of Fallot diagnoses have pulmonary atresia rather than stenosis. The risk is also higher in siblings (2.5–3%) and children of adults with tetralogy of Fallot (1.2–8.3%) [6]. There is a significant association with genetic syndromes. The common associations are the 22q11 deletion (approximately 45%), VATER (vertebrae, anus, trachea, esophagus, and renal) syndrome, and Alagille syndrome [7, 8]. Alagille syndrome is consistently recognized as a poor prognostic factor, predominantly due to liver dysfunction [7]. There is also a higher incidence of bronchus suis and an enlarged aorta that may cause tracheobronchial compression – especially with a right aortic arch and descending aorta. Commonly associated cardiac anomalies include atrial septal defects (ASDs), multiple VSDs, coronary artery abnormalities, left superior caval vein (SVC), and retroaortic innominate vein [9]. Clinical presentation is variable. Patients with a duct‐dependent pulmonary circulation with confluent PAs become cyanotic as the duct starts to close and may require resuscitation with prostaglandin if they have not been diagnosed prenatally. In contrast, patients with major aortopulmonary collateral arteries (MAPCAs) are often asymptomatic at birth as the collateral arteries provide pulmonary blood flow. Cyanosis develops later, although at variable rates. Finally, some infants and neonates may present with signs of pulmonary overcirculation and congestive heart failure. Thus the presentation of TOF/PA can be anywhere on the spectrum of completely inadequate to manifestly excessive pulmonary blood flow [10]. Cyanotic spells are uncommon but can occur, probably secondary to a drop in systemic vascular resistance resulting in relatively higher systemic peripheral blood flow and decreased pulmonary blood flow through major aortopulmonary collaterals [11]. In previously undiagnosed late‐presenting patients, clubbing and polycythemia will be present, and sometimes stroke or cerebral abscess may be the presenting feature. In the modern era of pediatric cardiology, widespread use of fetal echocardiography has led to improved antenatal diagnoses of most congenital heart diseases. TOF/PA is relatively easy to diagnose in the fetus, even though the multifocal sources of pulmonary blood flow may be difficult to identify. Nonetheless, if the arterial duct is not visible in a fetus with TOF/PA, then the likelihood of MAPCAs is high and all antenatally diagnosed neonates with this condition should undergo a thorough diagnostic evaluation postnatally. Few patients now present beyond infancy in the developed world and in our experience most presentations in late childhood are new immigrants and refugees from developing countries. Echocardiography is the mainstay of diagnosis. It delineates the intracardiac anatomy clearly and can identify associated anomalies such as ASDs, multiple VSDs, left SVC, retroaortic innominate vein, and coronary anomalies. It may also define whether the intrapericardial PAs are ductal dependent and confluent. Chest x‐rays and electrocardiograms are usually more useful for patient management rather than as diagnostic tools. Echocardiography is the sole form of diagnostic tool necessary in patients with a ductus‐dependent lung circulation. Other imaging modalities are required for more accurate determination of any multifocal pulmonary blood supply. Historically, cardiac catheterization has been the technique of choice. MAPCAs’ characteristics and distribution can be ascertained and selective injections of contrast into collateral vessels provide the most accurate demonstration of the nature of pulmonary blood supply to lung segments. Central PAs can be defined if there is a patent arterial duct or connection to a collateral. Aortic root injections for rare coronary collaterals, descending aortic injections with distal balloon obstruction, and selective subclavian artery injections may be necessary to define the entirety of the collateral pulmonary blood supply. Occasionally pulmonary venous wedge angiography may be useful to define the true PAs and/or collateral supply. Collateral stenoses and branch patterns are best visualized by catheterization. In expert hands, the exact distribution of the central pulmonary vessels and the dual supply of lung segments by both branching of the native pulmonary circulation and the collateral arteries can be identified. The hemodynamic evaluation of this condition remains less important than the actual delineation of its anatomy, although some still give relevance to the measured pressures in MAPCAs. It is feared that some segments of lung can remain hypertensive if subjected to very high pressures for prolonged periods. In the majority of cases, however, the pressures measured reflect the pressure in the proximal segment of the collateral vessels, and it is unclear whether the distal pressures of these often circumvoluted vessels is reflected in these measurements. The evidence of the development of localized hypertensive lung segments in these conditions remains anecdotal [12]. Computed tomography (CT) and magnetic resonance angiography (MRA) can provide a guide or roadmap of the collateral arteries. They will identify the presence of central pulmonary vessels, but will not enable identification of their exact distribution. Most of the collateral vessels can be identified, although details on their characteristics such as stenosis and existence of dual supply will be missing [13]. The indication for these three investigations depends on the overarching treatment strategy to be adopted. In patients where a neonatal PA rehabilitation strategy will be employed, CT or MRA provides adequate detail prior to the initial shunting operation and angiography can be avoided. In neonates in heart failure where little doubt exists on the distal pulmonary vasculature, surgery can at times be performed on the sole basis of CT or MRA. For those favoring single‐stage unifocalization, conventional extensive imaging by cardiac catheterization is necessary, even if an intermediate stage of shunting of the central PA is planned. The growth of the central PAs of patients should be followed by CT or MRA. Patients who are failing to grow their PAs may need cardiac catheterization to identify whether stenoses or dual blood supply and its associated competitive flow is the cause of the lack of observed growth. Cardiac catheterization is necessary to collect both anatomic and hemodynamic details to assess suitability for complete repair. At the core of this decision lies the predicted adequacy of the size of the pulmonary vascular bed after complete repair. Traditionally, the measurement of the Nakata index of the reconstructed pulmonary circulation has been the predominant factor guiding this decision. An index superior to 150 mm/m2 is usually considered as suitable to achieve a repair [14]. The total neopulmonary artery index, a calculation of the total surface of the pulmonary blood vessels supplying the lungs notwithstanding their origin, has been quoted to be an equally good estimate of the postoperative pulmonary pressure, with similar values (200 mm2/m2) being considered adequate for repair [15, 16]. Others have suggested basing the decision on preoperative magnetic resonance imaging (MRI) estimates of the pulmonary blood flow calculated as the total pulmonary venous return, or by subtracting SVC and descending aortic flow from ascending aorta flow [16]. Apart from some rare teams, the decision to proceed to repair is no longer based on the number of bronchopulmonary segments perfused by the reconstructed or rehabilitated pulmonary vasculature [17]. In borderline cases of patients undergoing unifocalization, intraoperative flow testing of the pressure achieved in the pulmonary circulation has been maintained by some teams who favor unifocalization procedures [18, 19]. TOF/PA with a duct‐dependent lesion was first treated by palliation with a systemic‐to‐pulmonary shunt [20]. Lillehei described a technique for anastomosing the PA directly to an RV infundibulotomy in 1954, and subsequently repair using a nonvalved conduit was first reported in 1965 and repair using a valved allograft in 1966 [21, 22]. However, until the mid‐1970s medical management was often considered the only option. Surgical treatment was either a systemic‐to‐PA or systemic‐to‐collateral shunt or an RV outflow reconstruction and ligation of MAPCAs, primarily as a palliative strategy [23–26]. Based on the morphologic and physiologic studies of the variable pulmonary blood supply, Haworth and Macartney postulated that pulmonary blood supply in these patients might be normalized by unifocalizing the individual PAs and MAPCAs to a single source [12, 27]. Since then, a number of surgeons and institutions have developed strategies for the management of this lesion. Most of these have been based on the concept of staged unifocalization, with the initial phases of management typically designed to increase flow to the true PAs in an effort to stimulate growth [28–34]. These strategies have provided good results for a select group of patients. However, very few if any institutions have cohorts of infants, and most leave a substantial proportion of patients without complete repair. For patients without MAPCAs, repair can be approached in the same way as simple tetralogy of Fallot – either palliation with a shunt or primary complete repair in the neonatal period, which is usually an institution‐ or surgeon‐dependent choice [35–37]. Exposure of the heart and great vessels and the initiation of cardiopulmonary bypass are performed in the same manner as described for tetralogy with pulmonary stenosis. Depending on the anatomy and surgeon preference, the continuity between the RV and the PAs can be obtained by a transannular patching or by right ventriculotomy and insertion of an RV to PA conduit. At times, the pulmonary atresia can be no more than an imperforate pulmonary valve and the RV outflow tract is no more obstructed than in a severe form of TOF/PA. Transannular patching avoids the implantation of valved conduit and its associated risk of reoperation. The operation then proceeds in a similar way to transventricular repair of tetralogy of Fallot (see Chapter 21). At other times, the atretic pulmonary valve is very small and dysplastic and the RV outflow tract is completely obstructed by muscle bundles. In this instance, transannular patching becomes long and tedious and the implantation of an RV to PA conduit gives a more direct pathway. The RV incision should be placed below the main level of obstruction, staying away from the left anterior descending artery and avoiding damage to the tricuspid valve’s subvalvar apparatus and the aortic valve. The location of the incision is best guided by the placement of a right‐angle forceps positioned inside the RV cavity by direct vision through the tricuspid valve. After excision of a circumferential button of ventricular wall, the inner edges of the incision are inspected and additional excision of muscle is performed if needed. The VSD is closed with the favored material using continuous or interrupted pledgeted sutures, as for tetralogy with pulmonary stenosis. After closure of the defect, the RV outflow tract is reconstructed. There has been considerable debate on the best possible RV to PA conduit. At a young age, homografts and Contegra bovine jugular vein conduit (Medtronic, Minneapolis, MN, USA) are the favored choices because of their availability in small sizes. Patients who have MAPCAs that provide a significant amount of pulmonary blood flow require a more complex treatment strategy. MAPCAs vary in terms of number, size, origin, course, arborization, and structure [4, 38]. They may constitute the sole source of pulmonary blood flow or may supply as little as a single lung segment. Various classifications have been proposed, but currently there is no agreement on a common classification [17, 39]. There is however an understanding that the severity of this condition varies widely. On the most severe end of the spectrum are patients whose pulmonary circulation depends exclusively on multiple small collaterals with no resemblance to pulmonary vessels. At the most favorable end are patients who have small central PAs distributing themselves to most of the pulmonary segments. Between these two extremes all variations are possible. Two operative strategies are currently used: primary unifocalization and PA rehabilitation [7, 39]. These have been wrongly seen as opposing strategies. This dichotomous view is favored by the complexity of the lesions and the fact that manuscripts detailing these strategies have summarized generic strategies rather than providing a precise description of the anatomy on which such operations are performed. Confusion is increased by the use of the term unifocalization, which encompasses both the translocation of PAs and patching of central pulmonary vessels. The agreed common practices and variations between these strategies are the following. Some neonates present in heart failure in the first weeks of life. It is agreed that, in these instances, the pulmonary circulation is adequate and they are therefore candidates for unifocalization and complete repair. Some of these patients have very well‐developed intrapulmonary vessels, with more often than not a limited number of collateral vessels feeding them. In both strategies, these patients will be directed to procedures aimed at the growth of central pulmonary vessels, in the form of a central shunt, or more rarely in some teams the creation of an RV to PA continuity by opening the main PA and infundibulum with patching. The procedures offered and their relative timing vary in both strategies. Partisans of the rehabilitation strategy contend that it is very difficult to identify the exact branching of these vessels before their growth is obtained, and that the best chance for providing this growth is in the first weeks of life; they therefore would recommend central shunting in most patients within a few weeks. Partisans of a primary unifocalization strategy would perform a one‐stage procedure involving the connection of all collateral vessels to centrally reconstructed vessels or enlarged central pulmonary vessels. The teams favoring this approach seem to delay the procedure to approximately 6–9 months of age. Most of these children are asymptomatic for long periods. There is currently contention about the proportion of these patients who have absent PAs. Large studies from Stanford and Birmingham claim that 20–35% present without any identifiable central vessels, while in a much smaller series of patients recruited from an entire population we found central pulmonary vessels to be present in all patients; however, 10% of the patients had central PAs too small to shunt [7, 17, 39
CHAPTER 22
Tetralogy of Fallot with Pulmonary Atresia and Major Aortopulmonary Collaterals
Clinical Features
Diagnostic Evaluation
In the Neonatal Period
Prior to Final Repair
Suitability for Repair
Surgical Management
Tetralogy of Fallot with Pulmonary Atresia and Ductus‐Dependent Lung Circulation
Tetralogy of Fallot with Pulmonary Atresia with Major Aortopulmonary Collateral Arteries
Indication for Surgery and Surgical Strategy
Neonates with Heart Failure
Central Pulmonary Vessels Distributed to Most Lung Segments
Central Pulmonary Vessels with Uncertain Distribution, Absent Central Pulmonary Arteries
Stay updated, free articles. Join our Telegram channel

Full access? Get Clinical Tree
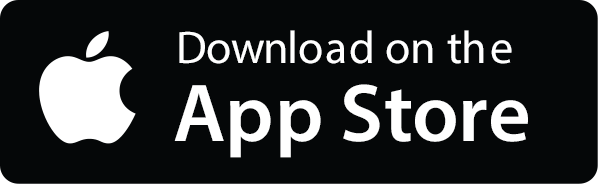
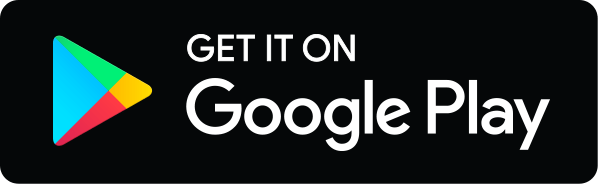