Objectives
- 1.
Describe lung volumes and their measurement using three methods.
- 2.
Discuss the uses of spirometry in clinical practice.
- 3.
Define the effort-dependent and effort-independent portions of the flow volume curve.
- 4.
Outline an approach to the interpretation of lung volumes, spirometry, and flow volume curves.
- 5.
Describe the physiology behind the diffusion capacity for carbon monoxide and list three diseases associated with abnormal results.
- 6.
Briefly outline the measurement of resistance and compliance.
Pulmonary function tests are designed to identify and quantify abnormalities in lung function. With the exception of exercise-induced asthma, pulmonary function tests do not diagnose disease. Rather, they describe pathophysiologic processes and help distinguish between cardiac and pulmonary disease. The two major groups of pathophysiologic processes that can be distinguished using pulmonary function tests are obstructive pulmonary disease (OPD) and restrictive pulmonary disease (RLD). Pulmonary function test results are a major contributing element of the history, physical examination, and laboratory data that aid clinicians in making a diagnosis and in assessing response to therapy and disease progression.
Lung Volume Measurement
As a general rule, all lung volumes are measured in liters. The most important lung volume measurement is the vital capacity (VC) or the total volume of air that an individual is able to exhale from a full and maximal inspiration to a maximal exhalation (see Chapter 2 ). When measured as a rapid exhalation, it is called the forced vital capacity (FVC). The FVC varies with age, height, sex, and ethnicity but is approximately 4 L in a healthy adult male.
The VC can also be measured as a slow exhalation (called the slow vital capacity [SVC]). In individuals with normal lung function, the FVC and the SVC are the same. In the presence of premature airway closure, however, the SVC is greater than the FVC because air trapping occurs during a forced maneuver secondary to dynamic airway closure (see Chapter 3 ). The difference between the SVC and the FVC is thus a measure of dynamic airway closure and is used more often by physiologists than clinicians.
Many lung volumes are altered in the presence of disease ( Table 4.1 ). The vital capacity is decreased in both obstructive and restrictive lung diseases. In the presence of chest wall muscle weakness, the chest wall outward recoil pressure is decreased, the lung recoil pressure is less opposed, and the functional residual capacity (FRC) decreases. In contrast, in the presence of air trapping, the FRC increases.
Obstructive Lung Disease | Restrictive Lung Disease | |
---|---|---|
TLC | N→↑ | ↓ |
RV | ↑ | ↓→N |
FRC | ↑ | ↓ |
RV/TLC (%) | ↑ | N→↑ |
The relationship between residual volume (RV) and total lung capacity (TLC) is called the RV/TLC ratio and is often used to help distinguish between restrictive pulmonary disease and OPD. In normal individuals, the RV/TLC ratio is less than 25%. An elevated RV/TLC ratio due to an increase in RV out of proportion to any change in TLC is caused by air trapping secondary to airway obstruction. An elevated RV/TLC ratio due to a decrease in TLC out of proportion to any change in RV is seen in individuals with restrictive lung disease.
Measurement of Lung Volumes
There are three commonly used methods to measure lung volumes: inert gas dilution, plethysmography, and nitrogen washout.
Inert Gas Dilution Technique
In the inert gas dilution technique ( Fig. 4.1 ), a known concentration of an inert gas such as helium, argon, or neon (this example uses helium) is added to a spirometer system that has a known volume. At FRC, the subject is connected to the system and breathes in the closed system until the helium concentration reaches a plateau, indicating equal concentrations of helium in the spirometer system and in the subject’s lung. Because helium is inert, the new helium concentration after equilibrium in the subject’s lung has occurred can be used to determine the subject’s FRC by solving for V 2 using the mass balance equation:
C 1 V 1 = C 2 ( V 1 + V 2 )

Plethysmography
Plethysmography can also measure lung volumes and is the preferred method. The principle of plethysmography is based on Boyle’s law (P 1 V 1 = P 2 V 2 at a constant [isothermal] temperature [Appendix C]). The gas in the lungs is isothermal because of its intimate contact with capillary blood.
A plethysmograph (also known as a body box) is an airtight box with pressure transducers for measuring the pressure inside the box and at the mouth ( Fig. 4.2 ). The mouth pressure measured with an open glottis and no airflow reflects alveolar pressure. The change in pressure inside the box is related to volume by introducing a small volume of gas into the box and measuring the change in pressure. The subject sits inside the airtight box and breathes through a mouthpiece connected to the outside. With temperature constant, the shutter on the mouthpiece closes and the individual gently and slowly pants against the closed mouthpiece ( Fig. 4.3 ). The change in pressure in the mouthpiece reflects alveolar pressure (there is essentially no airflow). Pressure changes inside the box reflect changes in pulmonary gas volume as gas within the chest is alternately compressed and decompressed by the action of the respiratory muscles. If the valve at the mouth closes while the subject is making an expiratory effort, alveolar pressure increases by an amount that is measured by the mouth pressure sensor, lung volume decreases as a result of gas compression, and, with no airflow, the pressure inside the plethysmograph decreases. This pressure change is related back to a change in volume, and all the elements of Boyle’s law are now present to solve for lung volume at FRC.


In individuals with normal lungs, FRC measured by helium dilution and by plethysmography are the same. The inert gas technique, however, can markedly underestimate FRC in individuals with OPD, because equilibration of the helium in markedly obstructed airways may not have occurred during the test (these areas have very long time constants). In contrast, plethysmography measures all gas in the lung at FRC, including trapped gas. Because of this, the difference in FRC measured by plethysmography and FRC measured by helium dilution has been used (primarily by physiologists) as a measure of trapped gas.
Nitrogen Washout Technique
The nitrogen washout technique is similar in many ways to the inert gas technique. The subject is connected to the system at end exhalation and breathes nitrogen-free air ( Fig. 4.4 ). At the start of the test, the lung contains 80% nitrogen that is evenly distributed. The volume of exhaled gas is collected into a bag and the nitrogen concentration is measured. Using the simple mass balance equation (C 1 V 1 = C 2 V 2 ), the concentration of nitrogen at the start (C 1 ) (i.e., in the lung) and at the end (C 2 ) (i.e., in the bag) is known, and the volume in the bag is known (V 2 ). The equation is then solved for the volume in the lung (V 1 ). The nitrogen washout technique is used infrequently because, like the inert gas technique, it underestimates FRC in individuals with airway obstruction; it can also be uncomfortable for subjects, and it requires a nitrogen analyzer and bags to collect expiratory gas.

Inert Gas Dilution Technique
In the inert gas dilution technique ( Fig. 4.1 ), a known concentration of an inert gas such as helium, argon, or neon (this example uses helium) is added to a spirometer system that has a known volume. At FRC, the subject is connected to the system and breathes in the closed system until the helium concentration reaches a plateau, indicating equal concentrations of helium in the spirometer system and in the subject’s lung. Because helium is inert, the new helium concentration after equilibrium in the subject’s lung has occurred can be used to determine the subject’s FRC by solving for V 2 using the mass balance equation:
C1V1=C2(V1+V2)

Plethysmography
Plethysmography can also measure lung volumes and is the preferred method. The principle of plethysmography is based on Boyle’s law (P 1 V 1 = P 2 V 2 at a constant [isothermal] temperature [Appendix C]). The gas in the lungs is isothermal because of its intimate contact with capillary blood.
A plethysmograph (also known as a body box) is an airtight box with pressure transducers for measuring the pressure inside the box and at the mouth ( Fig. 4.2 ). The mouth pressure measured with an open glottis and no airflow reflects alveolar pressure. The change in pressure inside the box is related to volume by introducing a small volume of gas into the box and measuring the change in pressure. The subject sits inside the airtight box and breathes through a mouthpiece connected to the outside. With temperature constant, the shutter on the mouthpiece closes and the individual gently and slowly pants against the closed mouthpiece ( Fig. 4.3 ). The change in pressure in the mouthpiece reflects alveolar pressure (there is essentially no airflow). Pressure changes inside the box reflect changes in pulmonary gas volume as gas within the chest is alternately compressed and decompressed by the action of the respiratory muscles. If the valve at the mouth closes while the subject is making an expiratory effort, alveolar pressure increases by an amount that is measured by the mouth pressure sensor, lung volume decreases as a result of gas compression, and, with no airflow, the pressure inside the plethysmograph decreases. This pressure change is related back to a change in volume, and all the elements of Boyle’s law are now present to solve for lung volume at FRC.


In individuals with normal lungs, FRC measured by helium dilution and by plethysmography are the same. The inert gas technique, however, can markedly underestimate FRC in individuals with OPD, because equilibration of the helium in markedly obstructed airways may not have occurred during the test (these areas have very long time constants). In contrast, plethysmography measures all gas in the lung at FRC, including trapped gas. Because of this, the difference in FRC measured by plethysmography and FRC measured by helium dilution has been used (primarily by physiologists) as a measure of trapped gas.
Nitrogen Washout Technique
The nitrogen washout technique is similar in many ways to the inert gas technique. The subject is connected to the system at end exhalation and breathes nitrogen-free air ( Fig. 4.4 ). At the start of the test, the lung contains 80% nitrogen that is evenly distributed. The volume of exhaled gas is collected into a bag and the nitrogen concentration is measured. Using the simple mass balance equation (C 1 V 1 = C 2 V 2 ), the concentration of nitrogen at the start (C 1 ) (i.e., in the lung) and at the end (C 2 ) (i.e., in the bag) is known, and the volume in the bag is known (V 2 ). The equation is then solved for the volume in the lung (V 1 ). The nitrogen washout technique is used infrequently because, like the inert gas technique, it underestimates FRC in individuals with airway obstruction; it can also be uncomfortable for subjects, and it requires a nitrogen analyzer and bags to collect expiratory gas.

Spirometry
The rapidity with which air flows out of the lung (known as the expiratory flow rate) can be measured using spirometry. Spirometry measures the rate at which the lung changes volume during a forced breathing maneuver ( Fig. 4.5 ). When performed from TLC, spirometry measures the FVC. In the “FVC maneuver,” the subject breathes in and out of a mouthpiece that is connected to a spirometry machine. The subject is then instructed to inhale maximally and then exhale as rapidly, as forcibly, and as completely as possible until he or she is unable to exhale further.

The FVC maneuver can be displayed in two ways: as a spirogram (volume/time curve) and as a flow volume loop.
Spirogram Display
In a spirogram, the volume of gas exhaled is plotted against time ( Fig. 4.6A ). The spirogram reports four major test results:
- 1.
The forced vital capacity (FVC)
- 2.
The forced expiratory volume in 1 second (FEV 1 )
- 3.
The ratio of the FEV 1 to the FVC (FEV 1 /FVC)
- 4.
The average midmaximal expiratory flow (MMEF), also known as the forced expiratory flow between 25% and 75% of the VC (FEF 25–75 ).

V ˙
max , maximal expiratory flow rate.
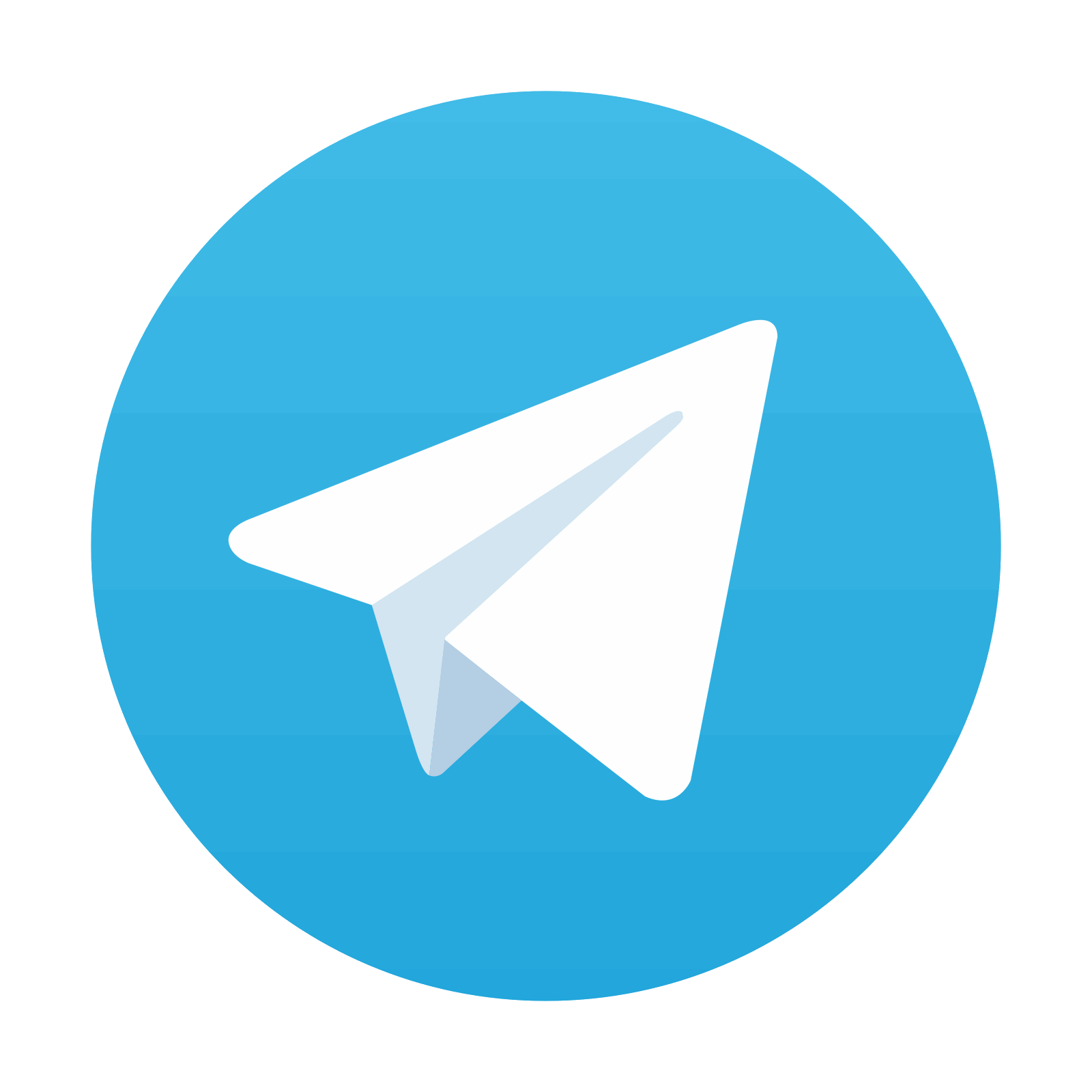
Stay updated, free articles. Join our Telegram channel

Full access? Get Clinical Tree
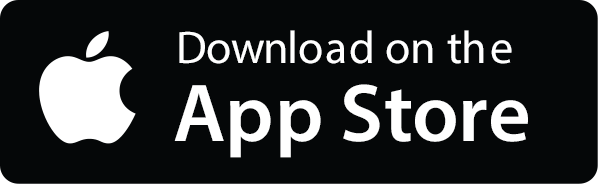
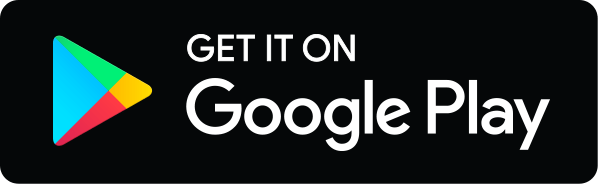
