Fig. 6.1
T cell activation in fungal infection. Dendritic cells (DCs) are at the crossroads of antifungal immunity and tolerance because both inflammatory and tolerogenic DCs may arise from their common immature progenitors. Following fungal recognition by specific pattern recognition receptors, inflammatory DCs may trigger the expression of subset-associated transcription factors and differentiation of naïve CD4+ T cells into T helper 1 (Th1), Th2, or Th17 cells, which may induce cytokine secretion and activation of specific antifungal immunity programs. On the other hand, plasmacytoid DCs may activate CD8+ cytotoxic lymphocytes (CTLs) that, by displaying direct fungicidal activity, also contribute to antifungal immunity. In addition, indoleamine 2,3-dioxygenase 1 (IDO1)-competent tolerogenic DCs produce kynurenines (Kyn) and cytokines that contribute to the expansion of interleukin (IL)-10-secreting T regulatory (Treg) cells, crucial for the maintenance of tolerance to the fungus. MR mannose receptor, IFN interferon, TLR Toll-like receptor, T-bet T-box expressed in T cells, Gata3 GATA-binding protein 3, Rorc retinoic acid receptor (RAR)-related orphan receptor C
1. Th1 Cells
Generation of a dominant Th1 response driven by IL-12 is essentially required for the expression of protective immunity to fungi and is compulsory for the design of effective antifungal vaccines (Spellberg et al. 2008). Through the release of the signature cytokine IFN-γ and by helping the production of opsonizing antibodies, induction of Th1 cells is instrumental for the optimal activation of phagocytes at sites of infection. Of interest, Th1 cell-based cross-protection for different fungi has been recently demonstrated to be achievable using a single immunogenic epitope from the A. fumigatus cell wall, thus providing attractive opportunities for immunotherapeutic strategies (Stuehler et al. 2011). The failure to deliver activating signals to effector phagocytes may predispose patients to overwhelming infections, limit the therapeutic efficacy of antifungals and antibodies, and favor persistency and/or commensalism (Romani 2011).
As noted above, Th cell skewing is determined by the way that DCs respond to the combination of fungal-derived TLR and C-type lectin receptor (CLR) signaling. Interestingly, dectin-1-mediated signals can alter the Th1 profile of CD4+ T cell responses to fungal infection by decreasing the production of IL-12 and IFN-γ in innate cells and consequent expression of T-box expressed in T cells (T-bet) in A. fumigatus-specific CD4+ T cells, thus enabling Th17 differentiation (Rivera et al. 2011).
Further supporting the pivotal role of Th1 cell activation, immunological studies on patients with polar forms of paracoccidioidomycosis demonstrate an association between Th1-biased reactivity and asymptomatic and mild forms of infection, as opposed to the correlation of Th2 cell responses with severe disease and poor prognosis. Thus, the finding that estradiol favors Th1-type immunity may explain why paracoccidioidomycosis is much more frequent in men than in women (Pinzan et al. 2010).
2. Th2 Cells
Progressive fungal infections, especially those acquired through the respiratory tract, are eventually associated with a shift in dominance from Th1 to Th2. IL-4 and IL-13 provide the most potent proximal signals for commitment of naïve T cells to the Th2 cell lineage. Despite often occurring coincidentally with vigorous Th1 responses, Th2 reactivity results in a net effect of poor control of the fungal burden because it induces alternatively activated macrophages that are permissive for intracellular fungal growth and may be impervious to signaling by IFN-γ (Voelz et al. 2009). In addition, Th2 reactivity alters pulmonary physiology such that airway resistance increases, thus compounding the severity of infection and contributing to fungus-associated allergic responses (Jain et al. 2009). Accordingly, attenuating Th2 responses to A. fumigatus in cystic fibrosis patients with allergic bronchopulmonary aspergillosis (Kreindler et al. 2010) or limiting IL-4 production in experimental models of histoplasmosis (Szymczak and Deepe 2009) restored antifungal resistance.
For the vast majority of fungi, Th2 responses are associated with pathogenic allergic responses. The contradiction is pneumocystosis, in which a Th2 cell-dependent humoral immunity affords some degree of protection (Rapaka et al. 2010). In addition, alternatively activated macrophages driven by IL-13 and amplified by IL-33 displayed enhanced fungicidal activity regardless of the antibody-dependent effect, a finding that further supports a contribution of Th2 cells to fungal clearance (Bhatia et al. 2011).
3. Th17 Cells
Over the past several years, the Th1/Th2 dichotomy has been replaced by the belief that the “fates” for developing CD4+ T cells and associated cytokines are more flexible than formerly anticipated (Zhou et al. 2009). Th17 cells are a separate lineage of effector Th cells contributing to immune pathogenesis previously attributed to the Th1 lineage (Kaufmann and Kuchroo 2009). Th17 cells have an important function in the host response against extracellular pathogens, but they are also associated with the pathogenesis of many autoimmune and allergic disorders. It is now well accepted that Th17 cell activation occurs in fungal infections (Hernandez-Santos and Gaffen 2012), mainly through the spleen tyrosine kinase/caspase recruitment domain-containing protein 9 (Syk/CARD9), myeloid differentiation primary response protein 88 (MyD88), and mannose receptor (MR), signaling pathways in DCs and macrophages leading to the production of unique cytokines such as IL-17, IL-17F, and IL-22. This signaling is inhibited by Raf-1 kinase and TIR domain–containing adapter–inducing interferon-beta (TRIF)/type I IFN pathways, indicating that the molecular pathways defining activation or inhibition of Th17 cells are present downstream of both CLRs and TLRs. Indeed, the central role of Th17 cells in antifungal immunity is supported by studies reporting Mendelian susceptibility to fungal infections of individuals with inborn errors of dectin-1, CARD9, STAT1, STAT3 and, specifically, IL-17 immunity (Ferwerda et al. 2009; Glocker et al. 2009; Puel et al. 2011; van de Veerdonk et al. 2011) (Table 6.1). In experimental fungal infections, however, IL-17-dependent immunity has been reported to be either essential (Huang et al. 2004; Saijo et al. 2007; Conti et al. 2009; Wuthrich et al. 2011) or not (Lin et al. 2009; De Luca et al. 2010b; Hardison et al. 2010). This suggests that the protective or detrimental effects of this pathway may depend on the stage and site of infection, probably influenced by environmental stimuli that induce cells to produce cytokines of the IL-17 family, including IL-22 (see below). In this regard, recent evidence has demonstrated that Th17 cells can be segregated into pathogenic or nonpathogenic cells, depending on whether they produce granulocyte macrophage colony stimulating factor (GM-CSF) or IL-10, respectively (Codarri et al. 2011; El-Behi et al. 2011). These Th17 phenotypes are still to be described in the context of fungal diseases (Wuthrich et al. 2012).
Table 6.1.
Mendelian susceptibility to fungal diseases
Primary immunodeficiency | Molecular/cellular defect | Reported fungal disease(s) | Associated gene | Mode of Mendelian inheritance |
---|---|---|---|---|
AD-HIES | Defective STAT3–dependent signaling (e.g., impaired generation of Th17 cells and signaling by IL-17R, IL-22R, and IL-23R) | CMC, histoplasmosis, cryptococcosis, and coccidioidomycosis | STAT3 | Autosomal dominant |
APECED | Loss of central tolerance, with persisting auto-reactive T cells and autoantibodies to cytokines (e.g., IL-17 and IL-22) | CMC | AIRE | Autosomal recessive |
CARD9 deficiency | Defective function of signalosomes for dectin-1, dectin-2 and other PRRs | CMC | CARD9 | Autosomal recessive |
DOCK8 deficiency | Impaired T cell activation and survival | CMC | DOCK8 | Autosomal recessive |
Hyper-IgM syndrome (HIGM) | Impaired co-stimulation of T cells and monocytes | Histoplasmosis and cryptococcosis | CD40L | X-linked recessive |
IL-12/IL-23 deficiency | Impaired development of Th17 cells | CMC | IL12B | Autosomal recessive |
IL12RB1 | Autosomal recessive | |||
IL-12/IFNG deficiency (also termed MSMD) | Impaired activation of macrophage intracellular killing | Paracoccidioidomycosis and coccidioidomycosis | IL12RB1 | Autosomal recessive |
IFNGR1 | Autosomal dominant | |||
STAT1 | Autosomal dominant | |||
IL-17 deficiency | Impaired development of Th17 cells | CMC | IL17F | Autosomal dominant |
IL17RA | Autosomal recessive | |||
SCID | Impaired generation of T cells, with or without concomitant B and NK lymphocytopenia | CMC | IL2RG | X-linked |
JAK3 | Autosomal recessive | |||
IL7R | Autosomal recessive | |||
CD3D | Autosomal recessive | |||
CD3E | Autosomal recessive | |||
RAG1 | Autosomal recessive | |||
RAG2 | Autosomal recessive | |||
DCLRE1C | Autosomal recessive | |||
CD45 | Autosomal recessive | |||
STAT1 deficiency | Defective STAT1-dependent signaling (e.g., impaired generation of Th1 and Th17 cells and signaling by IL-12R and IL-23R) | CMC | STAT1 | Autosomal dominant |
STAT1 gain-of-function | Increased STAT1-dependent cellular responses and impaired IL-17 immunity | CMC | STAT1 | Autosomal dominant |
TYK2 deficiency | Impaired receptor signaling (e.g. IL-23R) | CMC | TYK2 | Autosomal recessive |
Th17 cells are present in the fungus-specific T cell memory repertoire in humans (Acosta-Rodriguez et al. 2007; Bozza et al. 2009) and mediate some (Wuthrich et al. 2011), but not all (De Luca et al. 2012), vaccine-induced protection in mice. Interestingly, as in mice (De Luca et al. 2012), human host defense against A. fumigatus relies on Th1 rather than Th17 cell responses (Chai et al. 2010), and CMC patients (with or without autosomal dominant hyper-IgE syndrome) have defective Th17 and Th1 cell responses (Ryan et al. 2008; van de Veerdonk et al. 2011). This could be explained by the notion that Th17 cells, although found early during the initiation of an immune response, are involved in a broad range of both Th1- and Th2-type responses.
Indeed, a role for Th17 cells in supporting Th1 cell responses has been shown in experimental mucosal candidiasis (Conti et al. 2009; De Luca et al. 2010b). In addition, in experimental aspergillosis, enhanced Th2 cell responses and fungal allergy are observed in the absence of IL-17A receptor signaling (our unpublished observations). Thus, these findings point to an important regulatory function of the Th17 cell pathway in promoting Th1-type and restraining Th2-type immunity.
It is intriguing that Th17 cell responses are dampened by C. albicans (Cheng et al. 2010) and that failure to do so eventually results in chronic inflammation and failure to resolve the infection (Zelante et al. 2007; Loures et al. 2009). In this regard, it is fascinating that fungi are able to sense mammalian IL-17 in their surrounding environment and turn on molecular programs that result in enhanced virulence and survival aptitude (Zelante et al. 2012).
The mechanisms that link inflammation to chronic infection may lie in an inability to control inflammation following IL-17A-dependent neutrophil mobilization, thus preventing optimal protection and favoring fungal persistence. Thus, the Th17 cell pathway could be involved in the immunopathogenesis of chronic fungal disease, in which persistent fungal antigens may promote immune deregulation, as demonstrated in patients with autoimmune polyendocrine syndrome type 1 and in the absence of autoimmune regulator (AIRE), in which excessive Th17-type responses to fungi have been observed (Ahlgren et al. 2011).
As noted above, Th17 cells can concomitantly synthesize IL-22, a member of the IL-10 family of cytokines, which has been shown to play a more important role than IL-17 in host defense in the lung and gut (Zenewicz and Flavell 2008). Our recent findings suggest that the IL-23/IL-22/defensins pathway is crucially involved in the control of fungal growth at mucosal and nonmucosal sites in both candidiasis and aspergillosis, particularly in conditions of Th1 deficiency. Interestingly, memory IL-22+ CD4+ cells specific for C. albicans are present in humans (Liu et al. 2009) and are defective in patients with CMC (Eyerich et al. 2008). Thus, further tweaking the Th17 model, Th17 cells may exert their protective role in fungal infections through IL-22. Indeed, IL-22 has recently been demonstrated to be required for the control of C. albicans growth at mucosal sites in the absence of Th1 and Th17 cells (De Luca et al. 2010b). Specifically, IL-22 produced by NKp46+ innate lymphoid cells expressing the aryl hydrocarbon receptor directly targeted intestinal ECs and induced STAT3 phosphorylation and release of S100 calcium binding protein A8 (S100A8) and S100A9, peptides known to have antifungal activity and anti-inflammatory effects. Thus, in the relative absence of protective Th1/Treg, IL-22 + Th17 cells may fulfill the role of a protective response that exploits primitive effector defense mechanisms of antifungal resistance, as demonstrated also for experimental bacterial diseases (Aujla et al. 2008). Consistent with this role for IL-22, patients with autosomal dominant hyper-IgE syndrome, owing to dominant-negative mutations of STAT3, have a defective Th17 cell response to C. albicans (Milner et al. 2008). Accordingly, C. albicans-specific IL-22+ CD4+ memory T cells are present in healthy individuals (Liu et al. 2009) but are lacking in CMC patients (Eyerich et al. 2010), an observation pointing to IL-22 production in the mucosa as a primitive mechanism of resistance against fungi under conditions of limited inflammation. Of interest, dectin-1-mediated production of IL-22 in the lung has also been demonstrated to contribute to early innate immune resistance to A. fumigatus (Gessner et al. 2012), although it paradoxically promoted lung inflammation and immunopathology during persistent fungal exposure in an allergy model (Lilly et al. 2012). These seemingly discrepant findings further add to the complexity of IL-22 function in antifungal mucosal immunity and point to the existence of regulatory events leading to its production that depend on the stage and site of infection.
4. Treg Cells
During infection, the immune response must eliminate the fungus while limiting infection-associated costs to host fitness and restoring a homeostatic environment. Treg cells, by means of their anti-inflammatory activity, play a central role in this process. In experimental fungal infections, inflammatory immunity and immune tolerance in the respiratory or gastrointestinal mucosa have been shown to be controlled by the coordinated activation of different Treg cell subsets. Because Treg cell responses may handicap the efficacy of protective immunity, Treg cell activity decreases host tissue damage but may conversely promote fungal persistence (Romani and Puccetti 2006) and, eventually, immunosuppression (Ferreira et al. 2010). Some cells with this function, such as CD4+ Foxp3+ natural Tregs (nTregs), exist regardless of the presence of infectious stimuli, whereas others may be induced as a consequence of infection (iTregs) or in conditions of impaired co-stimulatory signaling and in the presence of deactivating cytokines and drugs. This scenario is crucially exemplified in experimental aspergillosis, in which inflammation was controlled at an early stage by nTregs suppressing neutrophils whereas, later tolerogenic iTregs inhibited Th2 cells and prevented fungal allergy (Montagnoli et al. 2006).
As already discussed, a reciprocal relationship has been described between the development of Foxp3+ Treg and effector Th17 cells, so that naïve T cell activation in the presence of innate stimuli redirects iTreg generation to Th17 generation. In this regard, CD4+ CD25+ Foxp3+ Treg cells have been recently found to promote IL-17 upregulation and contribute to suppression of mucosal candidiasis in vivo (Pandiyan et al. 2011). Thus, by controlling the quality and magnitude of effector innate and adaptive responses, the spectrum of Treg cell skills may go from protective tolerance and immune homeostasis to dominant effector activities. Furthermore, this suggests that this degree of interaction between fungi and the host immune system determines whether a fungus is perceived as commensal or pathogen, and that this definition may evolve constantly.
5. Indoleamine 2,3-Dioxygenase 1 is a Critical Regulator of Tolerance to Fungi
IDO1 is an IFN-γ-inducible intracellular enzyme that catalyzes the catabolism of tryptophan (Puccetti and Grohmann 2007; Mellor and Munn 2008). The effects of IDO1 activity are tryptophan deficiency, excess tryptophan breakdown products (kynurenines), and consumption of reactive oxygen species. IDO1 and kynurenines serve many roles in fungal infections. A number of studies have established that the proper control of infection and associated inflammatory reactions require IDO1 induction and consequent production of tryptophan metabolites with immunoregulatory activities, contributing to the maintenance of the Treg/Th17 balance (Romani et al. 2008b). As already mentioned, IDO1-expressing DCs are regarded as regulatory DCs specialized in antigen-specific deletional tolerance or induction of CD4+ CD25+ Treg cells. These findings disclose a mutual interaction between DCs and Treg cells for the preservation of immunological tolerance. Indeed, IDO1 blockade greatly exacerbated experimental fungal infections and the associated inflammatory pathology, and swept away resistance to re-infection, as a result of deregulated innate and adaptive immune responses caused by the impaired activation and functioning of suppressor CD4+ CD25+ Treg cells producing IL-10 (Romani and Puccetti 2006). IDO1 expression is paradoxically upregulated in patients with allergy or autoimmune inflammation, a finding pointing to the occurrence of a homeostatic mechanism to halt ongoing inflammation (Grohmann et al. 2007). During experimental fungal allergy, modulation of tryptophan catabolism via the glucocorticoid-induced tumor necrosis factor receptor (GITR) and its ligand, GITRL, inhibited Th2 cell responses and allergy and induced the expression of Foxp3+ Treg cells through IDO1-dependent mechanisms (Grohmann et al. 2007), a finding pointing to the potential relevance of IDO1 in the anti-inflammatory action of corticosteroids. As already mentioned, a reciprocal antagonistic relationship exists between IDO1 and the Th17 pathway, with IDO1 restraining Th17 responses and IL-17A inhibiting IDO1 (Zelante et al. 2007).
Recent evidence indicates that the non-hematopoietic compartment also contributes to tolerance to fungi via IDO1 (Cunha et al. 2010; de Luca et al. 2010a). ECs are key players in tolerance to respiratory pathogens via an IFN-γ–IDO1 axis culminating in the inhibition of Th17 cell responses (Desvignes and Ernst 2009; de Luca et al. 2010a). IDO1 overexpression in airway ECs was found to restrain CD4+ T cell activation to A. fumigatus, an activity that was nevertheless dispensable in the presence of IDO1-expressing tolerogenic DCs. However, IDO1 induction in ECs could compensate for the lack of IDO1 on hematopoietic cells (Paveglio et al. 2011). The expression of IDO1 on ECs occurred through the TLR3/TRIF-dependent pathway, a finding consistent with the abundant expression of TLR3 both intracellularly and on the cell surface of ECs. The failure to activate IDO1 probably accounted for the lack of tolerance to the fungus observed in experimental stem cell transplantation in conditions in which either the recipient or the donor (or even more when both) were TRIF- or TLR3-deficient (de Luca et al. 2010a).
Overall, these data shed light on pathways of immune resistance and tolerance to the fungus that probably take place in a hematopoietic stem cell transplantation setting. It appears that protective tolerance to the fungus is achieved through a TLR3/TRIF-dependent pathway activating Th1/Treg cells via IDO1 expressed on both the hematopoietic and non-hematopoietic compartments. In contrast, the MyD88 pathway provides antifungal resistance, i.e., the ability to restrict fungal growth through defensins and, probably, other effector mechanisms (de Luca et al. 2010a). However, the ability of mice to clear the fungus in the relative absence of the MyD88 pathway (Bretz et al. 2008) clearly indicates redundancies and hierarchy in antifungal mechanisms of resistance. Ultimately, the finding that both C. albicans (De Luca et al. 2007) and A. fumigatus (de Luca et al. 2010a), two major human fungal pathogens, exploit the TRIF/IDO1-dependent pathway at the interface with the mammalian hosts indicates that the exploitation of tolerance mechanisms is an advantageous option.
V. Immune Memory and Antifungal Vaccines
A successful vaccination relies on the elicitation of pathogen-specific immune memory that mediates long-term protection from infection or disease. Given the plethora of fungal ligands present at the cell surface, as well as those that become available to immune sensing upon processing of the fungus by phagocytic cells, it is clear that vaccine-induced protection to attenuated fungal strains occurs through distinct PRRs and downstream signaling adapters (Wuthrich et al. 2011; De Luca et al. 2012). For instance, Th17-induced acquisition of vaccine immunity to live attenuated strains of B. dermatitidis, H. capsulatum , and C. posadasii was found to require MyD88 signaling (Wuthrich et al. 2011 ), whereas Th1-induced protection to A. fumigatus relied on TRIF (De Luca et al. 2012 ). Of interest, vaccination with purified A. fumigatus antigens was found to be dependent on the MyD88 pathway in the presence of the appropriate adjuvant (Carvalho et al. 2012; De Luca et al. 2012), a finding pointing to the crucial role of adjuvants in promoting T cell differentiation along specific effector pathways. Thus, fungal innate sensing is one critical step in mounting immune responses, eventually defining appropriate effector responses to maximize protection (Levitz and Golenbock 2012).
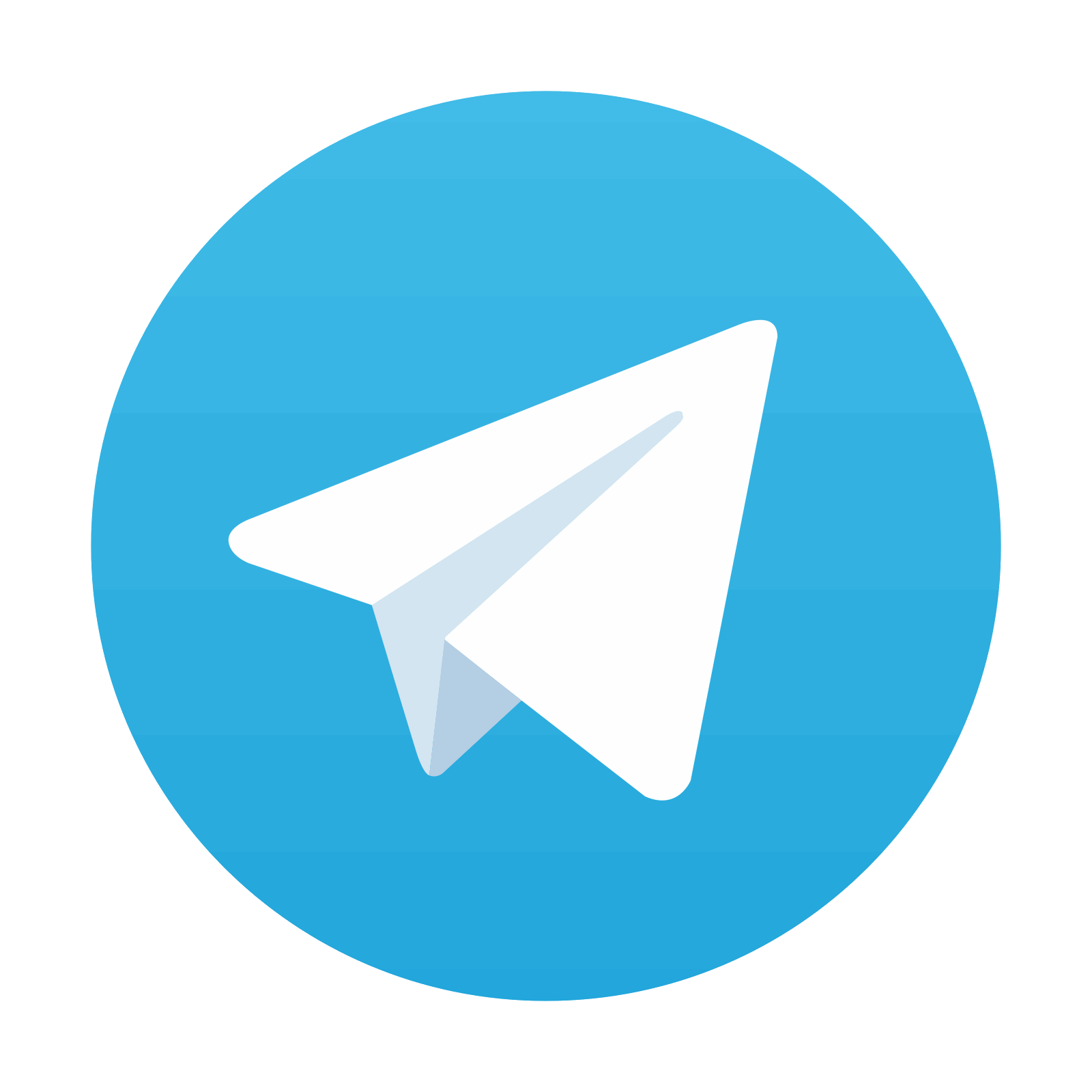
Stay updated, free articles. Join our Telegram channel

Full access? Get Clinical Tree
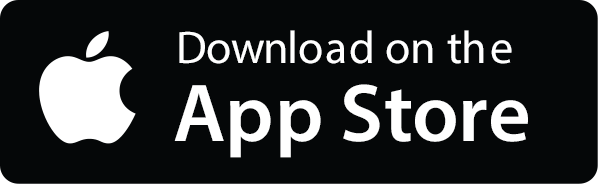
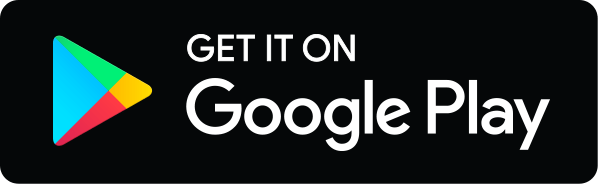