Stress Echocardiography: Introduction
- Patricia A. Pellikka, MD
In 1979 the use of cross-sectional echocardiography to detect reversible segmental dyssynergy during bicycle exercise was first described. Although the feasibility was limited, stress echocardiography was born: two-dimensional echocardiography was used to recognize exercise-induced regional myocardial ischemia in areas subtended by stenosed coronary arteries. Subsequent developments have resulted in the widespread acceptance of stress echocardiography and appreciation of its many advantages for detecting myocardial ischemia and viability and predicting prognosis, as well as for assessing other forms of heart disease.
Important technologic advances in the 1980s set the stage for further development of stress echocardiography. Digital echocardiography allowed the acquisition of cine-loop images, so that the cardiac cycles in which the endocardium was best visualized at rest and with stress could be acquired, saved, and replayed side by side. This facilitated storage, display, and interpretation of stress echocardiograms. The development of tissue harmonic imaging and other technologic advances of ultrasound systems and the approval by the U.S. Food and Drug Administration of contrast agents for left ventricular endocardial border detection in patients in whom one or more segments could not be adequately visualized greatly increased the feasibility of stress echocardiographic imaging. Various stress testing protocols have been developed and combined with echocardiography. These include treadmill exercise, upright or supine bicycle exercise, and various other methods for the patient unable to exercise. These nonexercise methods of stress testing include pharmacologic agents such as dobutamine, dipyridamole, and adenosine, with or without atropine or handgrip. The heart rate may also be increased by inserting a transesophageal pacing catheter, or if the patient has a permanent pacemaker, its rate can be increased. In the patient with resting regional wall motion abnormalities or global left ventricular systolic dysfunction, myocardial viability can be demonstrated by multistage stress echocardiography using dobutamine or exercise, in which images are obtained at each stage.
Validation studies of stress echocardiography were performed in the early 1990s. These studies documented the sensitivity and specificity of treadmill and bicycle exercise and pharmacologic stress echocardiography for detection of angiographic stenosis of a major coronary artery or significant branch vessel. Although not perfect, the results were superior to those obtained with exercise electrocardiography and had a higher specificity compared with the more widely used nuclear medicine technique of stress single-photon emission computed tomography (SPECT). At this point, the evidence supporting stress echocardiography as a valid test for evaluating patients with known or suspected coronary artery disease was regarded by the American Society of Echocardiography (ASE) as sufficient to merit a guidelines document. Hence in 1998, such a document was published. It reviewed the validation studies and the methodology for performing the stress echocardiography, including the necessary equipment and personnel, stress protocols, and analysis techniques. It also provided recommendations regarding the necessary training to perform and interpret studies; when such practices are followed, good reproducibility for segmental wall motion assessment has been demonstrated.
The next necessary step towards acceptance of stress echocardiography was to document its prognostic value. The utility of stress echocardiography in distinguishing patients at low risk versus those at high risk for cardiovascular events and mortality, based on stress echocardiography results and specific stress test characteristics, , was accomplished in large studies of consecutive patients from multiple institutions that had experience with the technique. The prognostic utility of stress echocardiography was thus shown with exercise stress , and with pharmacologic stress , , in patients with known or suspected coronary artery disease, including those with various symptom presentations, left ventricular dysfunction, left bundle branch block, and prior coronary artery revascularization. Stress echocardiography was shown to have a prognostic value similar to that of stress nuclear perfusion imaging. This large body of evidence prompted the ASE to issue new guidelines for stress echocardiography in 2007.
Although stress echocardiography had already become the stress test of choice at many large academic centers, its usefulness gained broader attention during 2009, when an unexpected shortage of radioisotopes for SPECT imaging prompted the more widespread application of stress echocardiography. Increasing requirements for preauthorization for the more expensive SPECT studies also contributed to increased use of stress echocardiography. More recently, greater physician and public awareness of the radiation exposure required during myocardial perfusion testing has contributed to the interest in stress echocardiography as an excellent alternative.
Compared with other forms of stress testing with imaging, stress echocardiography has several important advantages. The versatility of stress echocardiography is unparalleled. The rest images obtained at the start of stress allow rapid recognition of many conditions that can contribute to cardiac symptoms, such as valvular heart disease, hypertrophic cardiomyopathy, aortic dissection, diastolic dysfunction, pulmonary hypertension, and pericardial effusion. Incidental findings readily appreciated at the time of baseline image acquisition, such as left ventricular hypertrophy in a patient with hypertension, may influence prognosis and treatment decisions. Moreover, in the patient with cardiac symptoms, assessment is not limited to detection of ischemia; diastolic function, valvular heart disease, intraventricular gradients, and pulmonary artery pressures may be evaluated at rest and during stress to assess the contributions of these conditions to the patient’s symptoms.
Another advantage of stress echocardiography is its safety. Exercise and dobutamine stress echocardiography may be supervised by registered nurses, and the safety of this practice has been well documented. Administration of radioactive isotopes and iodinated contrast agents are not required, and contraindications to testing, such as acute aortic dissection, cardiac tamponade, and severe valvular heart disease, may be rapidly recognized at the time of baseline imaging. Together these attributes confer a safety profile superior to other tests. Moreover, for patients undergoing pharmacologic stress testing or supine bicycle stress testing, imaging may be performed at each stage of stress. This allows recognition of the heart rate at which ischemia first develops, a unique and useful feature of this test.
New modalities and enhancements of stress echocardiography will further augment its utility, feasibility, and accuracy. These include the use of myocardial contrast for perfusion imaging, three-dimensional stress echocardiography, and strain rate imaging.
From its beginnings more than 30 years ago as a test to detect ischemic heart disease during bicycle exercise, stress echocardiography has blossomed into a test that is applicable to many clinical conditions. It has been well validated and widely accepted as an extremely useful test for detecting myocardial ischemia and viability, developing prognosis, and evaluating therapy. Thirty familiar clinical scenarios in which stress echocardiography is appropriate are described in “2011 Appropriate Use Criteria for Echocardiography.” The value and utility of stress echocardiography in assessing other forms of heart disease, including pulmonary hypertension, cardiomyopathy, diastolic dysfunction, and valvular heart disease, will continue to develop. It costs less than other forms of stress imaging and does not require the use of radioactive isotopes, both of which make it a particularly attractive alternative. In the current era, it is more important than ever to reduce costs and prevent redundant testing, the versatility and comprehensiveness of this effective, widely available, and safe test distinguish it. The subsequent chapters in this section will highlight the current state of the art for stress echocardiography. Written by accomplished experts in the field, these chapters provide the reader with an excellent understanding of the utility and applications of this highly versatile test.
Effects of Exercise, Pharmacological Stress, and Pacing on the Cardiovascular System
- Wojciech Kosmala, MD, PhD
- Thomas H. Marwick, MD, PhD, MPH
The responses of the cardiovascular system to different stressors are not homogeneous and vary with the nature of the approach. Although laboratories typically have expertise with one or two approaches, it is desirable to have experience with alternatives, because no one test suits all patients and clinical scenarios.
Hemodynamic effects
Hemodynamic effects of treadmill or bicycle exercise include an increase in heart rate (due chiefly to sympathetic activation and partly to parasympathetic withdrawal); increase in inotropic state; increase in systolic blood pressure; decrease in systemic vascular resistance; and increase in venous return (due to sympathetic vasoconstriction of the large capacitance veins and the pumping effect of skeletal muscles), contributing via the Frank-Starling mechanism to the increase in stroke volume. , The increment of blood pressure with exercise is mainly due to an increase in cardiac output, which outweighs the decline in peripheral resistance. The opposite changes in afterload and preload are seen with isometric (handgrip) exercise ( Table 56.1 ). ,
Inotropic State | Heart Rate | Blood Pressure | Systemic Vascular Resistance | Venous Return | |
---|---|---|---|---|---|
Isometric exercise (handgrip) | ↑ – ↑↑ | ↑ – ↑↑ | ↑↑↑ | ↑ | ↓ * |
Supine bicycle exercise | ↑↑ | ↑↑↑ | ↑↑↑ | ↓ | ↑↑↑ |
Upright exercise | ↑↑ | ↑↑↑ | ↑↑↑ | ↓ | ↑ |
Dobutamine | ↑↑↑ | ↑↑ | ↑ – ↑↑ | ↓↓ | ↓ |
Vasodilators | ↑ | ↑ | ↓ | ↓↓↓ | ↓↓ |
Pacing (atrial) | 0 | ↑↑↑ | 0 | 0 | 0 |
The major effects of dobutamine are mediated by β 1 -adrenergic receptors. At low doses, an enhancement in myocardial contractility without tachycardia is a predominant response. At doses higher than 20 μg/kg/min, there is an increase in systolic blood pressure to about 170 mm Hg (i.e., by 30 to 40 mm Hg) and in heart rate to about 120/min (i.e., by 40/min to 50/min). , A decrease in blood pressure at higher doses is common, most commonly due to the vasodilating effect of dobutamine reflecting the stimulation of β 2 -adrenergic receptors. Bradycardia is less common and reflects a reflex response to blood pressure elevation or the activation of mechanoreceptors due to myocardial hypercontractility, or both.
The most commonly used coronary vasodilators—dipyridamole, adenosine, and regadenoson—involve the same metabolic pathway, either increasing endogenous adenosine levels (dipyridamole) or directly acting on the vasculature (exogenous adenosine or regadenoson, which is an A 2A adenosine receptor agonist). All these agents produce a small decrease in blood pressure and modest tachycardia with a minor increase in myocardial function.
Pacing represents an alternative method to increase cardiac work by the induction of tachycardia, usually of 160 beats/min. Atrial stimulation (either by transvenous or transesophageal approach) is a preferable technique, because asynchronous regional contractility from ventricular pacing provides interpretive challenges. Tachycardia attained with pacing is accompanied by stable blood pressure and unchanged loading, and a major advantage of this modality is a better hemodynamic control than with other stressors. , The disadvantage is that the lack of a suitable blood pressure response leads to a modest increment of rate-pressure product.
Ergonovine stress testing is thought to be the gold standard for diagnosis of coronary artery spasm. This drug is an agonist for β-adrenergic, dopamine, and serotonin receptors, through which it induces coronary vasoconstriction. The high sensitivity of this modality, even in patients with single-vessel spasm, can be explained by the transmural nature of vasospastic ischemia leading to severe wall motion abnormalities.
Mechanisms of ischemia
Exercise, dobutamine, and pacing can induce myocardial ischemia by increasing cardiac work and oxygen demand beyond what can be maintained when the blood supply is limited by significant coronary stenosis. Additional mechanisms responsible for ischemia include coronary flow maldistribution, with the reduction of subendocardial perfusion caused by adenosine accumulation in the myocardial tissue during stress, , , and the oxygen-wasting effect of dobutamine.
The use of vasoactive stressors is based in part on the development of perfusion heterogeneity. The generation of maximal vasodilation causes flow heterogeneity because the hyperemic response is limited in the territory supplied by a coronary stenosis. More controversially, it has been thought to provoke ischemia through coronary steal, implying that overperfusion of myocardial regions supplied by normal coronary arteries is at the cost of the heart muscle fed by the stenotic vessel (the so-called reverse Robin Hood effect). , In horizontal steal, vasodilation of distal arteriolar beds of nonstenosed coronary artery causes a decrease in perfusion pressure in the collateral circulation to the stenosed territory. Vertical steal is characterized by vasodilator-induced depressurization of the microcirculation in the territory of stenotic artery. The decrease in pressure collapses the subendocardial vessels because extravascular pressure in this layer is higher, and the subsequent flow maldistribution favors the subepicardium. Another contribution to the ischemic effect of vasodilators is increased oxygen demand from a reflex tachycardia in response to the decrease in blood pressure, or administration of atropine.
Left ventricular response to stress
The normal response elicited by inotropic stress includes an increase in endocardial systolic movement, systolic wall thickening, and velocity of contraction. The typical effect of dobutamine infusion is a reduction in end-diastolic and end-systolic volumes and a rise in cardiac output, which results more from elevated heart rate than stroke volume. An increased inotropic state together with the substantial decrease in loading may cause left ventricular (LV) cavity obliteration, which is a potential cause of false-negative responses, due to reduced transmural wall stress and smaller areas in which wall motion abnormalities can be identified.
A key marker of ischemia is a decrease in regional myocardial function from the resting level. The functional changes noted include both systolic excursion and wall thickening, which may also occur after an initial augmentation of contractility. The phase of transient enhancement of function is particularly important for recognition of ischemia in akinetic or dyskinetic segments. Despite their poor functional status, these segments may still have some ischemic or viable tissue. A deterioration of performance without antecedent improvement may reflect increased loading rather than ischemia.
Both left ventricular cavity dilation and a decrease in ejection fraction during stress are strong indicators of multivessel or left main coronary artery disease. However, these findings are much less common with pharmacological than exercise testing, possibly because of the reduction of loading. According to some observations, an abnormal end-diastolic volume response during dobutamine infusion implying severe coronary artery disease should be defined as a decrease of less than 15%.
Myocardium that is dysfunctional at rest but improves in response to low-dose dobutamine (less than 20 μg/kg/min) or dipyridamole (0.28 to 0.56 mg/kg) is considered to be viable. With dobutamine stress, the mechanism is clearly associated with adrenergic effects on contractility. However, the mechanisms behind the contractile response to dipyridamole are less obvious and possibly include sympathetic activation or systemic vasodilation causing a “local Frank-Starling effect,” whereby the expansion of myocardial blood volume leads to increased tension of the myofibrils.
In the presence of a patent infarct-related artery, the augmentation of function is sustained even if workload and oxygen demand increase. Generally this is understood to signify the presence of stunned myocardium, although nontransmural infarction can also provide this “uniphasic” response. As a consequence, this is not specific for functional recovery. Initial improvement at low heart rates followed by a deterioration of regional function, as tachycardia becomes more evident and induces ischemia, is typical for viable tissue supplied by a stenosed infarct-related artery, representing hibernating myocardium. , However, if the region is nourished by a critically narrowed artery, the stage of conspicuous improvement may not appear because of a very early advent of ischemia. The biphasic response is strongly predictive of functional recovery after revascularization, which is an argument for proceeding to maximal stress whenever possible ( Table 56.2 ). , However, it should be emphasized that the presence of severe LV dysfunction and extensive coronary artery disease necessitates a more cautious approach and early termination of the test.
Diagnosis | Function at Rest | Low Dose | High Dose |
---|---|---|---|
Normal | Normal | Normal | Hyperkinetic (mildly with vasodilators) |
Ischemic | Normal | Normal (unless severe CAD) | Reduction vs. rest Reduction vs. other segments Delayed contraction |
Viable, patent IRA (stunning) | Hypokinetic/akinetic | Improvement | Sustained improvement |
Viable, stenosed IRA (hibernation) | Hypokinetic/akinetic | Improvement | Reduction (vs. low dose) |
Infarction | Akinetic/dyskinetic | No change | No change |
Comparisons of stressors
Dissimilarities in hemodynamic effects of inotropic stressors account for the greater workload and consequently more extensive ischemia imposed by exercise than dobutamine. The rate-pressure product is higher with exercise (greater than 25,000) than with dobutamine (about 20,000) or pacing (less than 20,000). , , The potency of exercise or dobutamine in inducing myocardial ischemia (and as a result, their diagnostic sensitivity) is dependent on the target heart rate, with a maximal stress providing better reliability than submaximal testing. , β-Blockade may diminish the chronotropic response to dobutamine and decrease the double product to approximately 14,000, but this may be circumvented by the administration of atropine. The combined use of dobutamine and atropine also prevents vagal activation and the subsequent depression of heart rate that occurs in response to a hypertensive reaction or cardiac mechanoreceptor stimulation. The addition of atropine to vasodilator stress increases heart rate and myocardial oxygen demand, thereby improving diagnostic sensitivity.
Hypertensive response to stress
An exaggerated systolic blood pressure increase to greater than 200 mm Hg is not infrequent, particularly in hypertensive patients. The higher workload and greater ischemic burden caused by increased wall stress may facilitate the identification of coronary artery disease. However, the scenario poses problems for specificity because global or regional (especially apical) disturbances may be caused from increased afterload.
Parameters to assess for noncoronary indications
Mitral Regurgitation
Exercise stress echocardiography in mitral regurgitation (MR) may be helpful in the identification of patients suitable for surgical treatment. Echocardiographic findings, including increase in the extent of regurgitant jet, prominent elevation of pulmonary artery pressure, and the absence of contractile reserve, may indicate a need for the intervention. This is especially relevant in cases of ischemic MR, which changes dynamically according to alterations in loading conditions and inotropic state.
Supine bicycle exercise appears to be the most appropriate method to evaluate MR during stress. The hemodynamic profile of this approach, characterized by a large venous return and a moderate fall in systemic vascular resistance, contributes to unmasking the true severity of MR. In comparison with exercise, stress with dobutamine is not associated with an exacerbation of MR because it has a more pronounced inotropic effect that reduces the regurgitant orifice, a greater increase in afterload, and a decrease in preload produced by dobutamine and therefore it is not recommended for MR assessment. , Isometric exercise increases systemic resistance but also decreases preload because of the Valsalva effect. Upright exercise moderately decreases venous return and is believed to have an intermediate usefulness in the verification of MR severity. ,
Aortic Stenosis
Low-dose dobutamine echocardiography may help distinguish truly severe (low flow and low gradient) aortic stenosis (AS) from pseudosevere stenosis in patients with a decreased LV systolic function (low mean gradient less than 40 mm Hg and small valve area less than 1 cm 2 ). This setting may indicate either severe AS with a “poor” left ventricle incapable of generating adequate force to develop higher gradients or nonsevere AS with a small valve area because the cusps are unable to fully open at insufficiently elevated pressure inside the left ventricle. Dobutamine-induced increase in transvalvular pressure gradient with a relatively unchanged aortic valve area indicates severe disease, whereas improvement in valve area without a significant increase in the aortic gradient indicates pseudosevere AS. Another diagnostic benefit of stress with dobutamine is the evaluation of contractility reserve, defined as an increase in stroke volume greater than 20%, which provides prognostically relevant information.
Diastolic Dysfunction
Myocardial relaxation abnormalities provoked by ischemia may be demonstrated during each kind of stress, but this is more feasible using modalities with low heart rates, like dipyridamole or pacing (after termination of stress). Alterations in diastolic function in response to stress are not specific for ischemia and may appear because of left ventricular hypertrophy or myocardial fibrosis, or both. An increase in left ventricular filling pressure with exercise as an important cause of dyspnea may be identified by a postexercise E/e′ ratio higher than 13, but the utility of analogues assessment during pharmacological stress remains to be established.
Conclusions
The agents used for stress echocardiography provide a spectrum of physiologic effects. Careful selection permits the stressor to be tailored to the clinical situation, and various imaging tools can be used to examine not only wall motion but also hemodynamics, myocardial function, coronary flow, and perfusion to further extend stress echocardiography to new fields.
Diagnostic Criteria and Accuracy
- Kavit A. Desouza, MD
- Farooq A. Chaudhry, MD
Normal coronary arteries adapt to stress by coronary vasodilatation (coronary flow reserve), which increases blood flow to meet the increased oxygen demand. In the presence of a flow-limiting coronary stenosis, coronary flow reserve is impaired, resulting in a sequence of events called the ischemic cascade ( Fig. 57.1 ). Stress echocardiography identifies new reversible wall motion abnormalities precipitated by stress-induced ischemia using either exercise (treadmill or bicycle) or pharmacologic stress (dobutamine, dipyridamole, or adenosine). Wall motion abnormalities precede electrocardiographic (ECG) and hemodynamic changes in the ischemic cascade. Thus identification of new wall motion abnormalities by stress echocardiography is more sensitive and specific than hemodynamic changes, ECG changes, and symptoms at identifying flow-limiting stenosis.

Diagnostic criteria
The left ventricle (LV) is divided into 17 segments as recommended by the American Society of Echocardiography, and each segment is scored on a scale of 1 to 5 ( Table 57.1 ) during rest, at peak stress, and during the recovery phase. A normal response to stress is an increase in wall thickening and endocardial excursion in each of 17 segments and a decrease in left ventricular end-systolic volume.
Score | Wall Motion | Endocardial Motion * | Wall Thickening * |
---|---|---|---|
1 | Normal | Normal inward | Normal (> 30%) |
2 | Hypokinesis | Reduced inward (< 5 mm) | Reduced (< 30%) |
3 | Akinesis | Absent (< 2 mm) or reduced if dragged by adjacent walls | Absent (< 10%) |
4 | Dyskinesis | Outward | Thinning |
5 | Aneurysmal | Outward, with diastolic deformity | Absent and thinning |
LV segment responses can be classified as normal, ischemic, viable (stunned or hibernating), and infarcted and scarred myocardium as shown in Figure 57.2 . The normal response postexercise is myocardial thickening, increased excursion of the endocardium, and almost complete obliteration of the left ventricular cavity (Video 57.1). An abnormal (ischemic) response to stress is defined as deterioration in LV wall segment thickening and excursion during stress (increase in wall motion score of at least one grade) (Video 57.2, A-C ). In patients with wall motion abnormality at rest, ischemia is defined as improvement of wall motion with a low dose of dobutamine (viable myocardium) and deterioration of wall motion at the peak dose (biphasic response).

Semiquantification of Left Ventricular Wall Motion Using Wall Motion Score Index
Wall motion score index (WMSI) is calculated by dividing the cumulative sum score of 17 left ventricular wall segments by the number of visualized segments (scoring: normal segment = 1; hypokinetic = 2; akinetic = 3; dyskinetic = 4; and aneurysmal = 5). Patients with a normal stress echocardiogram (peak WMSI = 1.0) have a low risk of cardiac events (i.e., nonfatal myocardial infarction or cardiac death rate less than 1%/ year), and those with mild to moderate ischemia at peak stress (peak WMSI = 1.1-1.7) have an intermediate risk of cardiac events (up to 2.6%/year). However, patients with significant ischemia (peak WMSI greater than 1.7) have high risk of cardiac events (5.5%/year).
Wall Motion Abnormalities at Low Heart Rates
If new wall motion abnormalities occur at low or submaximal (less than 85%) age-predicted heart rates, multivessel coronary artery disease (CAD) is more likely and thus also carries a poor prognosis.
Left Atrial Size
Left atrial (LA) size is a marker of the severity and duration of left ventricular diastolic dysfunction in patients without significant mitral valve disease, systolic heart failure, or arrhythmias, and it further facilitates risk stratification of patients. A normal stress echocardiogram in the setting of a normal LA size confers a benign prognosis (less than 1%/year), whereas an increased LA size portends a worse prognosis regardless of whether the patient’s stress echocardiogram is normal. Hence LA size provides independent and incremental prognostic value, independent of traditional risk factors, LV ejection fraction, and echocardiographic myocardial ischemia.
Right Ventricular Function
The right ventricle (RV) can be analyzed by visual assessment of parasternal long-axis, parasternal short-axis, apical four-chamber, and subcostal images acquired at rest and peak stress. The RV wall motion is scored in three segments: base, mid, and apex on a scale of 1 to 5, similar to the LV scale for grading wall motion. The peak RV WMSI is calculated as the cumulative sum score of the three segments divided by the number of visualized RV segments (normal RV WMSI = 1.0). Abnormal RV function (wall motion abnormalities, either new [ischemia] or fixed [infarction]) is seen in up to 4% of patients referred for stress echocardiography and can further stratify risk and add incremental prognostic value. The risk of cardiac events increases exponentially from 1.3%/year to 11.4%/year with an increase in peak RV WMSI from 1.0 to higher than 2.0, respectively, implying a greater risk with RV involvement.
Transient Ischemic Dilatation
Transient ischemic dilatation (TID) is an abnormal increase in end-systolic LV volume with stress (stress/rest LV volume ratio greater than 1.17), which along with a decrease in global systolic function is usually associated with multivessel CAD and a poor prognosis, with a very high cardiac event rate (19.7%/year). Furthermore, the longer it takes for stress-induced wall motion abnormalities to revert back to baseline upon cessation of stress, the more severe the degree of stenosis in the culprit vessel.
Diagnostic accuracy
Coronary angiography has been considered the gold standard to compare the diagnostic accuracy of noninvasive stress testing modalities in detecting flow-limiting CAD. However, coronary angiography yields “luminograms,” so this approach has several limitations. It can yield false-positive and false-negative results because it evaluates coronary anatomy but not the physiologic effects of flow-limiting CAD or microvascular hemodynamics. Studies have shown marked disparity between the apparent severity of coronary artery lesions and their effects on blood flow.
Typically using angiography as the gold standard, greater than 50% stenosis by quantitative coronary angiography and 70% stenosis by visual estimation is considered to be significant. Causes of false-positive or false-negative results are listed in Table 57.2 . The overall sensitivity for stress echocardiography is 75% to 85%, and specificity is 80% to 90%. Table 57.3 outlines the sensitivity, specificity, and diagnostic accuracy of different stress echocardiographic modalities in various cohorts. The sensitivity and specificity are comparable in men and women and across different stress modalities (e.g., exercise, dobutamine, and vasodilator). The sensitivity of stress testing increases when the achieved heart rate is greater than 85% of the age-predicted maximal heart rate.
False Positive (Lowered Specificity) | False Negative (Lowered Sensitivity) |
---|---|
Microvascular disease (e.g., diabetes, LVH, HCM, syndrome X) | Submaximal stress (< 85% maximal predicted heart rate) |
Hypertensive response during stress | Delayed post-stress image acquisition |
Cardiomyopathies: focal myocarditis, takotsubo, and idiopathic | Poor image quality |
Paradoxical septal motion (e.g., post cardiac surgery, left bundle branch block, RV volume overload, RV pacing) | Very mild ischemia, circumflex coronary stenosis, branch or distal stenosis |
Coronary spasm, endothelial dysfunction | Good coronary reserve (collateral circulation), potent endothelial function |
Localized basal inferior wall motion abnormalities, tethered wall segments | Antianginal drug therapy during testing (calcium channel blockers, beta-blockers, nitrates) |
Inexperienced personnel, interpreter bias | Inexperienced personnel |
Studies | Study Size | Sensitivity (%) | Specificity (%) | Accuracy (%) |
---|---|---|---|---|
Jang et al. (2011) ,† | 1287 patients with treadmill exercise SE (Korean population) | 68 | 83 | 72 |
Peteiro et al. (2012) | 116 patients | |||
Peak supine bicycle SE | 84 | 63 | 77 | |
Peak treadmill exercise SE | 75 | 80 | 77 | |
Post treadmill exercise SE | 60 | 78 | 66 | |
Aggeli et al. (2011) | 60 patients with dobutamine SE | |||
Two-dimensional | 80 | 82 | ||
Real-time, three-dimensional with perfusion | 82 | 64 | ||
Meta-analyses | ||||
Geleijnse et al. (2009) | 62 studies (6881 patients) | |||
With prior MI | 83 | 81 | ||
Without prior MI | 74 | 85 | ||
With RWMA | 82 | 81 | ||
Without RWMA | 76 | 88 | ||
Fleischmann et al. (1998) | 24 studies (2637 patients), exercise SE | 85 | 77 | |
Gargiulo et al. (2011) | 11 studies (701 hypertensive patients) | 77 | 89 | |
De Albuquerque Fonseca et al. (2001) | 8 studies (533 patients) | |||
Dipyridamole | 72 | 82 | 77 | |
Treadmill | 79 | 92 | 80 | |
Picano et al. (2008) | 5 studies (435 patients) | |||
Dipyridamole | 85 | 89 | 87 | |
Dobutamine | 86 | 86 | 84 |
* Obstructive disease considered > 50% stenosis on coronary angiography.
† Negative predictive value, 61%; positive predictive value, 83%.
In patients with greater than 50% angiographic stenosis, the sensitivity of stress echocardiography to detect one-, two-, or three-vessel CAD increases from 58% to 86% and 95%, respectively. In patients with poor image quality, the use of echocardiographic enhancing contrast agents improves endocardial border definition and thus the wall motion analysis, especially in the problematic anterior and lateral walls. This also increases the interobserver and intraobserver agreement and improves sensitivity of the test without affecting specificity, hence converting a suboptimal to an optimal study. Among expert readers with good interpretation skills, stress echocardiography is a safe, cost-effective, reliable, and reproducible test in the diagnosis, risk stratification, and prognostication of coronary artery disease.
Stress Echocardiography Methodology
- R. Raina Roy, MD
- Robert McCully, MD, MBChB
- Steven J. Lester, MD
- Robert McCully, MD, MBChB
Stress echocardiography, with its unique ability to give insight into both the morphologic and physiologic cardiovascular adaptations to exercise or stress, has evolved into a principle tool for the evaluation of individuals with known or suspected cardiovascular disease. For patients with either known or suspected obstructive coronary artery disease, stress echocardiography relies primarily on being able to characterize the functional consequences of significant coronary stenosis, which is the development of new left ventricular wall motion abnormalities. This chapter will outline commonly used stress echocardiographic clinical protocols employed for both diagnosis and risk assessment of patients with either suspected or known coronary artery disease.
Exercise echocardiography
The ability to gain insight into patients’ functional capacity along with heart rate and blood pressure response makes exercise preferred over pharmacologic stress testing. Patients who are able to perform routine activities of daily living without much difficulty should be considered for exercise testing. Exercise stress echocardiography is performed with either a treadmill or a bicycle based on the individual patient and the information desired.
Baseline imaging includes the acquisition of an apical four-, three-. and two-chamber views along with a parasternal long- and short-axis views. In addition to standard views, an apical short-axis view can be acquired to ensure proper visualization of the apex, which can sometimes be foreshortened or inadequately visualized on the apical views. The subcostal view may be used in patients who have limited parasternal windows. Intravenous contrast agents should be administered when two or more left ventricular wall segments are inadequately visualized. Patients abstain from food or drink 3 hours before testing.
Versatility and comprehensiveness are among the strengths of stress echocardiography compared with other modalities (e.g., nuclear stress testing). To fully exploit the strengths of echocardiography, the baseline echocardiogram should include the following:
- 1.
An evaluation of valve morphology and function
- 2.
An anatomic evaluation of the aortic root and ascending aorta
- 3.
A volume-based measure of left atrial size
- 4.
An estimation of right ventricular systolic pressure
- 5.
A mitral inflow velocity profile using pulsed wave Doppler
- 6.
A medial and/or lateral mitral annular early diastolic velocity (e′ velocity) using pulsed wave Doppler
Exercise protocols
Treadmill
Treadmill exercise testing is the most widely used form of exercise testing in the United States. It confers the benefit of ease of use with less dependence on patient cooperation compared with bicycle ergometry. Treadmill testing should be supervised by a health-care professional trained to monitor exercise tests. A registered nurse can effectively fulfill these needs, which include continuously monitoring the patient’s electrocardiogram (ECG) and frequently assessing blood pressure.
The most commonly used treadmill protocol is the multistage Bruce protocol, and it has been validated in the literature. Stage 1 of the Bruce protocol starts at a speed of 1.7 miles per hour (mph) with a 10% incline. With each 3-minute stage, speed and incline are progressively increased ( Fig. 58.1 ). The fourth stage and beyond may involve light jogging or running. The modified Bruce and Naughton protocols are lower intensity exercise protocols that may be used in older patients or those with limited exercise capacity. The Cornell protocol provides a more gradual increase in speed and incline than the Bruce protocol. The patient is periodically asked to rate their perceived exertion using the Borg exertion scale from 6 to 20 ( Table 58.1 ). Maximal exercise is thought to be achieved when the patient reaches a perceived exertion score of 18 on the Borg scale. Exercise testing should be limited by symptoms but may be terminated earlier in specific situations ( Table 58.2 ). ,

Graded Scale | Perceived Exertion |
---|---|
6 | |
7 | Very, very light |
8 | |
9 | Very light |
10 | |
11 | Fairly light |
12 | |
13 | Somewhat hard |
14 | |
15 | Hard |
16 | |
17 | Very hard |
18 | |
19 | Very, very hard |
20 |
Absolute Indications | Relative Indications |
---|---|
|
|
|
|
|
|
|
|
|
|
|
|
|
|
|
Immediately upon cessation of exercise, the patient is instructed to lie in the left lateral decubitus position such that the sonographer is able to acquire postexercise images as soon as possible after peak heart rate has been achieved. This may be challenging and requires the patient’s cooperation with breath-holding instructions from the sonographer to prevent lung and motion artifacts. The postexercise images of the left ventricle mirror those of the images obtained at rest, as discussed earlier (apical four-, three-, and two-chamber views along with parasternal images). Images should be acquired within 1 minute after exercise to ensure diagnostic accuracy, because regional wall motion abnormalities can resolve rapidly upon cessation of exercise. , Failure to acquire images within 1 minute is an important cause of false-negative results in stress echocardiography. The images are displayed in a quad format, which includes rest and stress images from each view. It has been our practice to display the rest image and then three separate single-beat clips of each view post exercise. Therefore we will review at least five separate sets of rest-stress images for each study. This allows the reviewer the opportunity to review more than one poststress image for each view obtained.
Bicycle Ergometry
Bicycle exercise echocardiography may be done with an upright, semirecumbent, or recumbent stationary bicycle. The cycles are calibrated in kiloponds or watts, which can then be converted to metabolic equivalents (METs). One benefit of cycle ergometry is that it may allow image acquisition during exercise and is therefore of benefit when Doppler information is requested, such as when valvular heart disease and pulmonary hypertension are assessed. In addition, bicycles require less space and are less expensive than treadmills. Patients tend to pedal slower as they fatigue, and thus the ability to vary the resistance with the pedaling speed improves power output control. A common protocol for cycle ergometry is to begin at a workload of 25 W and increase by 25 W every 2 to 3 minutes. As with treadmill exercise, cycle ergometry should also be limited by symptoms. Image acquisition is performed at baseline, 25 W, peak exercise, and in recovery. A major limitation of cycle ergometry is patient discomfort with the supine bicycle and fatigue of the quadriceps muscles, which can lead to termination of the test before reaching maximal oxygen uptake. In patients who are unaccustomed to cycling, their maximal oxygen uptake can be 10% to 20% less than that achieved with a treadmill. ,
Pharmacologic stress protocols
Dobutamine
For patients who are unable to exercise, pharmacologic stress echocardiography is a practical alternative. , The most widely used pharmacologic agent is dobutamine. Dobutamine is a synthetic catecholamine that has predominant inotropic effects when used at lower doses (less than 10 μg/kg/min) via its effect on β 1 -adrenergic receptors in the heart. Thus at low doses of dobutamine, there is increased contractility. Dobutamine has a very short plasma half-life of less than 2 minutes, so it must be administered as a continuous infusion. Dobutamine also has effects on β 2 -adrenergic and α 1 -adrenergic receptors, leading to a balanced effect on the peripheral vasculature at lower doses. However, as the dose of dobutamine increases, there is a greater response through β 2 -adrenergic receptors, resulting in peripheral vasodilatation, which may lead to a decrease in blood pressure. The standard protocol for pharmacologic echocardiography is to initiate a dobutamine infusion at 5 μg/kg/min for 3 minutes, and then increase the dose to a maximum of 40 μg/kg/min. Our laboratory starts at 5 μg/kg/min, followed by 10 μg/kg/min, 20 μg/kg/min, 30 μg/kg/min, and 40 μg/kg/min every 3 minutes. The patient’s ECG is continuously monitored, and blood pressure is assessed at each stage of infusion. When an adequate increase in heart rate is not achieved, atropine may be used in doses of 0.25 mg IV every minute up to a maximum of 2 mg. In our lab, atropine is administered under the following conditions: if the heart rate is less than 90 bpm at the end of the 20 μg/kg/min stage, if the heart rate is less than 70% of the maximum predicted heart rate at the end of the 30 μg/kg/min stage, or if the heart rate is less than 85% of the maximum predicted heart rate at the end of the 40 μg/kg/min stage. Atropine increases heart rate by exerting its vagolytic effect and has a serum half-life of 3.5 hours. The handgrip maneuver may also be used to augment the heart rate response (see later).
During dobutamine stress echocardiography, the test should be terminated when the patient reaches 85% of the maximal age-predicted heart rate; if new or worsening wall motion abnormalities develop; or significant arrhythmias, hypotension (more than 20 mm Hg from baseline blood pressure), severe hypertension (greater than 240 mm Hg systolic blood pressure or greater than 120 mm Hg diastolic blood pressure), or intolerable symptoms occur. , Common side effects of dobutamine include headache, chills, nausea, palpitations, arrhythmias, angina, hypotension, and urinary urgency. Contraindications to dobutamine stress echocardiography are severe symptomatic aortic stenosis, uncontrolled hypertension, decompensated heart failure, uncontrolled arrhythmias, acute pulmonary embolism, acute aortic dissection, acute myocardial infarction within past 2 days, and acute myocarditis. A beta-blocker such as intravenous esmolol may be administered to help reverse the side effects of dobutamine. Esmolol is a short-acting intravenous beta-blocker that is administered in a dose of up to 0.5 mg/kg. Sublingual nitroglycerin can also be given if the patient develops angina. Transthoracic images of the left ventricle include the apical four-, three-, and two-chamber views along with parasternal long- and short-axis views. Images of the left ventricle are acquired at baseline, low dose, prepeak, peak stress, and recovery. The images are displayed in a quad format, which includes the rest, low-dose, prepeak, and peak images. The recovery images are displayed separately. In our lab, the patient is monitored during the recovery period until the heart rate returns to within 20 beats of the baseline heart rate, wall motion abnormalities return to baseline, and the patient is asymptomatic.
In patients with permanent pacemakers, dobutamine can be administered using the following protocol. The infusion begins at 5 μg/kg/min for 3 minutes, followed by 10 μg/kg/min for 3 minutes, and 20 μg/kg/min for 3 minutes. If the patient’s heart is paced, dobutamine is continued at 20 μg/kg/min for 3 minutes while pacing at 70% of the maximum predicted heart rate (MPHR). If the patient’s heart is not paced, dobutamine is continued at 20 μg/kg/min and the heart is paced just above the resting heart rate for 3 minutes; then the pacing rate is increased to 70% of the MPHR. The pacing rate is then increased to 85% of the MPHR for 3 minutes with dobutamine administered at 20 μg/kg/min; this is followed by a cooldown period.
Vasodilator Stress Echocardiography
Other pharmacologic agents include vasodilators such as dipyridamole and adenosine. In stenotic vessels, the amount of myocardial blood flow is less than that of nonstenotic vessels, leading to regional ischemia via a “steal” phenomenon. This may be detected by echocardiography as regional wall motion abnormalities. However, when ischemia is used to define an abnormal test result, based on the induction of left ventricular regional wall motion abnormalities, vasodilator stress is less sensitive than dobutamine stress; thus dobutamine stress is preferred. For this reason, the administration of atropine during dipyridamole stress echocardiography is routinely performed by many institutions.
Other modalities
Handgrip
To augment heart rate and blood pressure, the handgrip maneuver has been used alone and as an adjunct to both exercise and pharmacologic stress testing. Patients are asked to grip a tennis ball and exert 30% maximum strength of a sustained handgrip for approximately 30 seconds. This can be done during the last 30 seconds of the pharmacologic or the exercise study. If patients have severe ischemia, the use of handgrip alone may be sufficient to cause wall motion abnormalities. Given the small incremental increase in heart rate and blood pressure, isolated handgrip is not routinely employed in the use of stress echocardiography.
Diastolic Stress Test
The clinical utility of stress echocardiography has expanded as the test has evolved beyond simply evaluating stress-induced left ventricular wall motion abnormalities. For patients who develop dyspnea or declining exercise capacity and in whom the resting echocardiogram suggests normal left ventricular filling pressure, an evaluation of pulmonary artery systolic and left ventricular filling pressures may be included in the postexercise period. Immediately post exercise, as discussed previously, images of the left ventricle are first obtained to evaluate changes in left ventricular ejection fraction, end-systolic volume, or regional wall motion. An estimate of pulmonary artery systolic pressure is then obtained by applying the simplified Bernoulli equation to the peak tricuspid regurgitation velocity, if present. Finally the mitral inflow E-wave and medial mitral annular (e′) velocities are obtained to derive an E/e′ ratio. , Stress-induced changes in the E and e′ velocities may persist for several minutes into recovery when the E and A waves of the mitral inflow signal are no longer fused. This allows for the analysis of regional and global left ventricular function and pulmonary artery systolic pressure and the opportunity to wait until the discrete E and e′ velocities are visible post stress. Diastolic stress testing can be performed with either exercise or dobutamine stress.
Stress Echocardiography: Image Acquisition
- David M. Dudzinski, MD, JD
- Michael H. Picard, MD
The accuracy and reproducibility of stress echocardiography depend on attention to proper image acquisition. Although most stress echocardiograms are performed for assessment of coronary artery disease, the sonographer and physician must tailor the imaging protocol to the specific aims of the test, which may also include assessments of valvular regurgitation, valvular stenosis, pulmonary hypertension, and diastolic parameters.
The American Society of Echocardiography guidelines on stress echocardiography and quality operations offer details on image acquisition. The ultrasound device should employ electrocardiographically triggered image acquisition. The echocardiography reading station should also allow split- and quad-screen displays to facilitate simultaneous comparisons of images at various stages in the stress protocol. This type of display ( Figs. 59.1 and 59.2 ; Videos 59.1, A – D and 59.2, A – D ) is crucial in the assessment of subtle wall motion abnormalities in each segment. Tissue harmonic imaging, which enhances definition of the endocardium and reduces near-field artifacts, should be routinely used in stress echocardiography.


Comprehensive baseline (rest) images must include all segments of the left ventricle for comparison with postphysiologic stress images or pharmacologic stress images; whenever possible, this stage should also include a quick assessment of other structures that might cause the symptoms prompting the test (described later). When assessing for ischemia, the minimum baseline series of images should include parasternal long-axis, parasternal short-axis at mid-papillary muscle level, apical four-chamber, and apical two-chamber views. If parasternal long-axis views are not available, apical long-axis views may be substituted. Ideally, short-axis images should include views at the mitral valve, midventricle, and apical levels. Although stress echocardiography display software programs allow digital acquisition and multistage comparisons for any view, typically only one short-axis view, the midventricular (mid-papillary) level, is included in the stress echocardiography imaging display because myocardium from all three coronary artery territories is shown in this view. However, the other short-axis images (basal and apical) can be saved in the digital archive for more detailed comparison. Subcostal short-axis views may be substituted if the parasternal window is unavailable, but this view is more dependent on patient positioning and there may be limited time at peak stress to reposition the patient to optimize this window. The apical views may offer the only images of the left ventricle apex, and so it is important to prevent foreshortening. In the apical view, it is also important that both diastolic and systolic images depict the four chambers (and visualize both mitral and tricuspid valves), and not the left ventricle outflow tract, which might lead to misinterpretations of the basal midseptum function. When at least two contiguous endocardial segments cannot be delineated, contrast agents should be used for both rest and peak images. Contrast dose at peak can often be lower than the dose at baseline because flow is increased.
For patients who have not had a recent transthoracic echocardiogram, the baseline images should be considered as a screen for other causes of the symptoms prompting the stress echocardiogram. A brief assessment for aortic stenosis, pulmonary hypertension, mitral regurgitation, and pericardial disease can be performed with images of aortic valve leaflet opening, continuous wave Doppler of tricuspid regurgitation, color Doppler of the mitral valve, and assessment of pericardial effusion. If resting images demonstrate asymmetric septal hypertrophy or systolic anterior motion of the mitral valve, then the left ventricular outflow tract should be imaged with continuous wave Doppler to ensure that stress testing is not performed on patient with unrecognized obstructive hypertrophic cardiomyopathy, especially if a resting peak late systolic pressure gradient of at least 50 mm Hg is obtained. Other findings on prestress screening that may prompt cancellation of the test include significant aortic disease, severe left ventricle systolic dysfunction, or left ventricular thrombus.
With treadmill exercise echocardiographic protocols, the patient must move from the upright running position to the bed quickly so that imaging can be performed before wall motion abnormalities resolve: the aim is for these images to be acquired within 1 minute of achieving peak exercise heart rate. During bicycle exercise stress, echocardiographic images can be taken during pedaling at peak exercise. Thus analysis of echocardiographic images acquired during exercise protocols will include three panels: resting baseline, immediate post–peak exercise (treadmill) or peak exercise (bicycle), and recovery (see Fig. 59.1 /Video 59.1, A – D ). For dobutamine protocols, images of each view at four stages will be obtained and compared: baseline, low dose, peak dose, and recovery (see Fig. 59.2 /Video 59.2, A – D ).
To facilitate comparisons to the resting images and promote accurate identification of wall motion abnormalities, the sonographer should obtain the peak images in the same imaging planes and at the same depth as the baseline images. This can be challenging when patients have exaggerated respiratory motion during and after exercise. Given the limited time available at peak heart rate, speed in acquisition is essential. Patients may need to be instructed to enact brief breath holds at midinspiration or midexpiration to maintain the echocardiographic window. For posttreadmill imaging, the apical views should be obtained first. Certain landmarks can help ensure that the same myocardial territory is imaged both during stress and at rest. This includes visualization of a thickened valve chordae tendineae or highly reflective echoes within a specific myocardial segment. Also, if short-axis views are oblique rather than orthogonal to the long axis of the left ventricle, wall motion may be misinterpreted. Whenever possible, wall motion abnormalities should be confirmed on at least one additional view. At the conclusion of image acquisition, a quality check should be conducted to ensure that the best images are used in the comparison displays. The sonographer should check that all images are labeled with the correct window name (e.g., apical 4 chamber [A4C]) and that the electrocardiographic waveform is the same on all images (e.g., the sonographer should exclude images acquired during ventricular ectopic beats).
Different imaging protocols are required for other applications of stress echocardiography, such as for the assessment of symptoms thought to be due to subclinical diastolic dysfunction, valvular disease, or pulmonary hypertension. For example, if assessment of diastolic function is required during stress echocardiography, then typically the transmitral pulsed wave Doppler and mitral annular pulsed wave Doppler are acquired at rest and peak stress. Because wall motion abnormalities recover faster than the alterations in the Doppler patterns, the images of the left ventricle myocardium should be acquired first during the peak stress or immediate poststress imaging stage. If the stress echocardiography test is being performed to assess the significance of low gradient aortic stenosis in the setting of reduced left ventricular ejection fraction, then pulsed wave Doppler of the left ventricular outflow tract and continuous wave Doppler across the aortic valve are acquired at rest and typically under stress that is induced with a 20 μg/kg/min dose of dobutamine. For evaluation of valve disease symptoms, the window for peak gradient must be established at rest because imaging through multiple windows may not be possible while at peak exercise stress. As an example, when assessing aortic valve gradients, the right parasternal window is often not available at peak bicycle stress when the patient is in the left lateral position. In cases of mitral stenosis where symptoms are nonspecific or absent, or where exertional symptoms are out of proportion to the degree of mitral stenosis found on the resting echocardiogram, stress echocardiography can also be used to determine if transmitral gradients rise or right ventricular systolic pressure significantly increases ( Figs. 59.3 and 59.4 ; Video 59.4, A – D ). In this setting, the continuous wave Doppler is obtained across the mitral valve and tricuspid valve at both rest and peak stress (or with treadmill exercise, immediately poststress). Stress echocardiography can also be used in cases of asymptomatic significant mitral or aortic regurgitation to determine if exercise ejection fraction decreases, left ventricular cavity size increases, effective regurgitant orifice area changes, or right ventricular systolic pressure increases. In these scenarios, specific Doppler assessments are added to the standard images of the left ventricle at rest and peak stress.


The results of stress echocardiography are critically dependent upon proper image acquisition and display. Each accredited echocardiography laboratory should conduct quality review and improvement initiatives. Specifically in regard to image acquisition, for all sonographers who perform stress echocardiography, a peer review of 5 to 10 studies should be conducted each year to ensure adherence to the protocol for image acquisition.
Prognosis
- Vikram Agarwal, MD, MPH
- Farooq A. Chaudhry, MD
Stress echocardiography, first introduced in 1979, represents the merger of cardiovascular stress testing with two-dimensional echocardiography. Although initially introduced primarily for detection of coronary artery disease (CAD), its applications have expanded and it is now routinely used to provide prognostic information about major subsequent adverse cardiovascular events among various cohorts. The studied patient populations include but are not limited to patients with chronic CAD ; post myocardial infarction ; preoperative risk stratification before noncardiac surgery ; chest pain or dyspnea ; varying pretest probability of CAD ; elderly patients ; both men and women ; conduction abnormalities ; resting left ventricular dysfunction ; prior coronary revascularization ; and underlying diabetes mellitus. Stress echocardiography is now used in prognostic testing so that proper interventions can be performed earlier in patients with highest risk for adverse outcomes. Multiple stress echocardiography parameters have been used for risk stratification and prognosis. Box 60.1 lists some of the variables associated with adverse outcome that are discussed in this chapter.
- •
Reduced baseline LVF (WMSI or EF)
- •
Inducible ischemia
- •
Extensive
- •
Low ischemic threshold
- •
In LAD coronary artery territory
- •
Long duration of wall motion abnormality
- •
- •
Poor EF response
- •
Failure to reduce end-systolic volume or transient LV cavity enlargement
- •
Right ventricular wall motion abnormalities
- •
Left ventricular hypertrophy
- •
Enlarged left atrium at baseline
- •
Reduced HRR
EF, Ejection fraction; HRR, heart rate reserve; LAD, left anterior descending; LV, left ventricular; LVF, left ventricular failure; WMSI, wall motion score index.
Risk stratification and prognosis with extent and severity of wall motion abnormalities
Stress echocardiography yields prognostic information for risk stratification of patients with known or suspected ischemic heart disease. In a study including 1500 patients, a normal stress echocardiography study (peak wall motion score index [pWMSI] = 1) conferred a benign prognosis (cardiac event rate, 0.9% per year), whereas pWMSI (relative risk [RR], 2.1; 95% confidence interval [CI], 1.0-4.4; P = .04) and left ventricular ejection fraction (LVEF) (RR, 1; 95% CI, 0.9-1.0; P = .01) were both predictors of adverse cardiac events. Based on the pWMSI, risk in the cohorts could be further stratified into low-risk (pWMSI, 1; cardiac event rate 0.9%/year), intermediate risk (pWMSI, 1-1.7; cardiac event rate 3.1%/year) and high-risk (pWMSI, 1-1.7; cardiac event rate 5.2%/year) groups. An LVEF threshold of 45% was also used to stratify risk in the overall patient cohort, separating them into low- and high-risk groups, whereas each group could be further stratified into low-, intermediate-, and high-risk groups based on their pWMSI. When pWMSI was factored in, the adverse cardiac event rates in patients with LVEF greater than 45% were 0.8%/year (pWMSI, 1), 2%/year (pWMSI, 1.1-1.7), and 2.3%/year (pWMSI, greater than 1.7). Similarly, in patients with LVEF of 45% or less and an overall higher cardiac event rate, pWMSI could stratify patient risk into low-risk (pWMSI, 1; adverse cardiac event rate = 5.1%/year) and high-risk groups (pWMSI, 1.1-1.7; adverse cardiac event rate, 6.2%/year; and pWMSI greater than 1.7, adverse cardiac event rate, 5.6%/year).
In addition, the extent of ischemia (number of left ventricular wall segments with new wall motion abnormalities) and the severity of ischemia (maximal magnitude of new wall motion abnormality) can predict adverse cardiac event rates. In a study of 1500 patients, ischemic extent (χ 2 = 48.7; P < .0001) and maximal severity (χ 2 = 52; P < .0001) were exponentially correlated with an increase in event rate. When both the extent and severity of ischemia was incorporated into a three-dimensional model, the predicted adverse cardiac event rate ranged from a low of 0.9%/year in patients without any wall motion abnormalities to a high of 6.7%/year in patients with extensive and severe wall motion abnormalities ( Fig. 60.1 ), which represents a sevenfold increase.

Single vessel and multivessel coronary artery disease
In patients with angiographically known coronary artery disease, stress echocardiography provides additional and incremental prognostic value over the coronary angiography data. In a study of 260 patients with angiographically significant coronary artery disease (more than 70% in major epicardial vessels or branches), where 45% had single-vessel disease and 55% had multivessel disease, (hazard ratio [HR], 2.53; 95% CI, 1.16-5.51; P = .02) and the number of segments in which ischemia was present (HR, 4.31; 95% CI, 1.29-14.38; P = .01) were predictors of adverse cardiac events, In addition, in the Cox proportional hazard model, stress echocardiography provided small but significant incremental value over clinical, electrocardiographic (ECG), and coronary angiographic data, with the highest global chi-square value for predicting adverse cardiac events.
Prediction of myocardial infarction versus cardiac death
Stress echocardiogram can be used to separately prognosticate outcome-specific end points of cardiac death and nonfatal myocardial infarction (MI). Although low ejection fraction was the strongest predictor of cardiac death in a study that included 3259 patients (χ 2 = 37.3; P < .0001), the strongest predictor of nonfatal MI was extent of ischemia (χ 2 = 12.3; P < .0001). For each 1% drop in ejection fraction, the risk for cardiac death increased by 5%, whereas for each additional ischemic segment, the risk for nonfatal MI increased by 15%.
Functional parameters, including heart rate reserve, important in prognosis
Although the inability to achieve 85% of the age-based maximum predicted heart rate (MPHR) is extensively used, low chronotropic index and hence heart rate reserve (HRR), which is based on age and resting heart rate, has been shown to be superior to 85% MPHR in patients undergoing exercise stress test. In this study comprising 1323 patients, HRR was defined as [(peak heart rate − heart rate at rest)/(220 − age − heart rate at rest)] × 100, whereas low HRR was defined as HRR less than 70%. The authors found that a low HRR is a predictor of cardiac event independent of ischemia (RR, 2.15; 95% CI, 1.23-4.01; P = .013). In the setting of a low HRR, a normal stress echocardiogram carries a less benign prognosis, whereas an abnormal stress echocardiogram leads to a more malignant prognosis. Even in patients with normal stress echocardiography results, the inability to achieve 85% MPHR conferred a higher, intermediate cardiac event rate of 2.9%/year, and the ability to exercise 9 minutes on the Bruce Protocol to achieve at least 10 metabolic equivalents (METS) confers an overall low cardiac event rate of 0.4%/year.
Heart rate when wall motion abnormality occurs
Patients with submaximal MPHR in the setting of normal stress echocardiography results have a higher risk of cardiovascular events than those who attained maximal stress test. In a meta-analysis comparing patients with submaximal MPHR with patients who achieved age-appropriate MPHR, patients with submaximal MPHR had a higher risk of hard events (cardiac death and myocardial infarction; RR, 1.7; 95% CI, 1.25-2.31; P = .0008) and a higher risk of total cardiac events (RR, 2.27; 95% CI, 1.54-3.31; P < .0001). When evaluating patients only with submaximal MPHR, a positive stress test (i.e., transient worsening wall motion abnormalities or biphasic response on echocardiogram during stress test) was associated with a significantly higher risk of adverse cardiac events, as compared with patients with normal stress echocardiography (RR, 3.78; 95% CI, 2.81-5.08; P < .0001). Thus the results of submaximal MPHR in the setting of normal stress echocardiography should be considered for more accurate risk stratification and prognosis.
Prognostic value of stress echocardiography versus stress electrocardiography
Although ST depression on stress ECGs is associated with worse cardiovascular outcomes, concurrent echocardiographic imaging is frequently associated with findings that may be concordant or discordant to the ECG findings. Studies suggest that even in the setting of an ischemic stress electrocardiographic response, the presence of a normal stress echocardiogram portends a benign prognosis (cardiac event rate, 1.1% per year). Abnormal stress ECG results provided limited prognostic risk stratification, except in the setting of poor exercise capacity or a concordantly abnormal stress echocardiographic finding. Stress-induced myocardial ischemia provides additional prognostic value over historical, clinical, and stress electrocardiographic variables across all pretest probability subgroups. However, the best incremental value of stress echocardiogram over other variables is in the intermediate pretest probability subgroup.
Duration of regional wall motion abnormalities
With dobutamine stress testing, additional information about the extent of myocardial ischemia can be obtained by assessing the time required for reversible wall motion abnormalities to normalize. In a study of 65 patients, complete resolution of regional wall motion abnormalities occurred within 25 minutes in patients with multivessel CAD, within 20 minutes in those with two-vessel disease, and within 15 minutes in those with single-vessel disease ( P < .001). The greater the wall motion score index at peak stress, the longer the duration of regional wall motion abnormalities into the recovery phase ( P < .01). In addition, regional wall motion abnormalities persisted long after normalization of each patient’s symptoms, electrocardiographic changes, heart rate, and rate-pressure product during recovery.
Role of right ventricular wall motion
During stress echocardiography the presence of an abnormal right ventricle, ischemic or infarcted, has been shown to be an independent predictor of events (HR, 2.69; 95% CI, 1.22-5.92; P = .014). Right ventricular abnormalities can further stratify risk and provide incremental prognostic value over rest and conventional stress echocardiography variables.
Transient ischemic left ventricular cavity dilatation
Transient ischemic dilation (TID) of the left ventricular cavity during stress echocardiography signifies marked ischemia. TID during stress echocardiography is associated with a very high rate of adverse cardiac events (cardiac event rate, 19.7%/year) and a very high relative risk of adverse cardiac events compared with patients with normal stress test (RR, 6.17; 95% CI, 1.1-33.3; P < .0001). TID is not only a sensitive marker of severe and extensive angiographic CAD, but also a marker for possible multivessel CAD (Video 60.1).
Left atrial size
Left atrial size has been shown to provide independent and incremental prognostic value in patients referred for stress echocardiography. In a group of patients without significant mitral valve disease and relatively preserved left ventricular ejection fraction, an enlarged left atrium, defined as a left atrial size indexed to body surface area of at least 2.4 cm/m 2 , was associated with worse hard cardiovascular events compared with patients with normal-sized left atrium (RR, 1.84; 95% CI, 1.19-2.85; P = .006). An enlarged left atrium could also be used to further stratify risk in both the normal and abnormal stress test groups.
Warranty time of a normal stress echocardiogram
Whereas the low event rate among patients with normal stress echocardiogram is well established, the “warranty period” of a normal stress echocardiogram is not well defined. In a patient cohort with a remote history of MI (at least more than 6 weeks previous), stress echocardiography effectively stratified patient risk into normal and abnormal subgroups (event rate 0.8%/year versus 4.2%/year; P = .01; RR, 5.6; 95% CI, 1.3-24.7). In patients with a normal stress echocardiogram, the event rates at the end of 6, 12, and 18 months were less than 1%/year. After 18 months, the event rate in patients with a normal stress echocardiogram increased significantly to more than 1%/year.
In the current economic scenario of reducing health-care resources, stress echocardiogram plays a pivotal role in assessing not only underlying CAD, but also the presence, extent, and severity of inducible ischemia. It provides invaluable information about prognosis across a wide spectrum of patient populations. Stress echocardiograms provide information above and beyond the routine clinical and demographic variables. It provides valuable assistance in risk stratification and prognosis and may also play an important role in the allotment of scarce health-care resources.
Viability
- Sripal Bangalore, MD, MHA
- Farooq A. Chaudhry, MD
Heart failure is a leading cause of morbidity and mortality, with a 5-year mortality rate as high as 50%. It is the leading cause of hospitalization in patients older than 65 years. Coronary artery disease is the leading cause of heart failure, and left ventricular (LV) systolic function is an important prognostic marker. Numerous studies have shown that LV systolic dysfunction is a potentially reversible condition related to myocardial stunning, hibernation, or a combination of the two mechanisms. Segments that lose function as a result of acute ischemia, despite the restoration of normal perfusion, are known as stunned myocardium (transient postischemic dysfunction) ( Fig. 61.1 ). Myocardial stunning results from a mismatch between coronary flow and myocardial function, and these segments are likely to recover function spontaneously over time ( Table 61.1 ). On the other hand, hibernating myocardium is the term used to refer to segments rendered dysfunctional secondary to chronically underperfused myocardium ( Fig. 61.2 ). Hibernating myocardium results from a compensatory decrease in myocardial function as a consequence of chronic ischemia. Both stunned and hibernating myocardium can potentially improve their function and are collectively referred to as viable myocardium . Several observations suggest that the hibernation and stunning may represent different spectrums of the same condition, and that in some cases, hibernation may result from repetitive stunning by multiple episodes of ischemia.

Diagnosis | Coronary Flow | Myocyte Metabolism | Prognosis |
---|---|---|---|
Stunned myocardium | Normal | Decreased | Spontaneous recovery of myocardial function with time |
Hibernating myocardium | Decreased | Decreased | Improvement in function with revascularization |

In patients with ischemic cardiomyopathy, only 40% show improvement in ejection fraction (EF) after revascularization, leaving a substantial subset with no improvement. The perioperative mortality rates from coronary artery bypass grafting in patients with ischemic cardiomyopathy range from 5% to more than 30%. Furthermore, revascularization of nonviable myocardium has not proven to be beneficial for either mortality or improvement in global LV function. In a meta-analysis of 24 viability studies, revascularization of patients without viable myocardium was associated with a trend toward higher annual mortality rate compared with medical management alone (7.7% versus 6.2%, P = .23). On the contrary, revascularization of viable myocardium has been shown to be associated with increased EF, decreased congestive heart failure symptoms, and improved survival compared with those treated medically. However, patients with viable myocardium who did not undergo revascularization had annual mortality rates five times higher than similar patients who were revascularized. The survival benefit of revascularization of a viable myocardium increases with decreasing LV function as well as increasing number of viable segments. These data, however, emerge from a meta-analysis of retrospective studies with varying inclusion and exclusion criteria, different definitions of patients with or without viable myocardium, and inherent biases in the selection of patients for revascularization.
Although the conventional wisdom with data from observational studies suggests a beneficial effect of revascularization on improvement in LV function and outcomes in patients with ischemic cardiomyopathy with viable myocardium, this is not fully supported by data from randomized trials. In the Surgical Treatment for Ischemic Heart Failure (STICH) trial of patients with ischemic cardiomyopathy, assessment of myocardial viability with either single-photon emission computed tomography (SPECT), DSE, or both did not identify patients who had a survival benefit from coronary artery bypass grafting compared with medical therapy alone. Although patients with viable myocardium had significantly lower overall rates of death and cardiovascular hospitalization than those without viable myocardium, this was no longer significant after adjustment for baseline characteristics. Before we throw out the baby with the bath water, it is important to recognize the limitations of the STICH viability substudy. In this study, viability assessment was not randomized, which results in substantial differences in baseline characteristics between the groups with and without viability assessment. In addition, only half of the patients underwent viability assessment, and evaluations were performed using two different imaging modalities. Moreover, there were substantial differences in patients with and without viable myocardium. The patients who underwent viability assessment had greater prevalence of myocardial infarction, heart failure and increase in LV end-diastolic volume, and lower EF. In addition, the majority of patients who were tested had viable myocardium. The small proportion of patients with limited myocardial viability decreases the statistical power for such comparisons. It is also unclear whether nonviable areas were subject to revascularization in the STICH trial. In addition, questions have been raised about the definition of viability used and possible quality control issues of image acquisition and viability protocol, given that it is a multicenter trial. Finally, it is not clear if the lack of mortality difference is due to lack of benefit from coronary artery bypass graft or due to significant improvement in modern optimal medical therapy alone.
Viability of myocardium can be ascertained using different imaging techniques ( Table 61.2 ). Currently the methods used for predicting myocardial viability include assessment of contractile reserve (CR) in dysfunctional regions using low-dose dobutamine stress echocardiography (DSE), assessment of cell membrane integrity using SPECT with thallium-201 (stress-redistribution-reinjection, stress-reinjection 24-hour imaging, or rest-redistribution imaging) and assessment of myocyte metabolic activity using fluorodeoxyglucose F 18 positron emission tomography (FDG-PET). Other techniques that are currently being developed include myocardial contrast echocardiography (MCE) and contrast-enhanced magnetic resonance imaging. ,
Imaging Technique | Definition of Viability | Measure | Disadvantage |
---|---|---|---|
FDG-PET | Myocardial segments with decreased perfusion but with intact metabolic activity | Identifies myocyte metabolic activity Measures viability in the:
|
|
201 Tl-SPECT | Myocardial segments with decreased perfusion with delayed uptake | Identifies cell membrane integrity Measures viability in the:
|
|
DSE | Wall motion abnormality at rest that improves with low-dose dobutamine infusion | Identifies inotropic contractile reserve Measures viability in the:
|
|
MRI | Delayed enhancement (5-20 min) indicates fibrosis or scar | Identifies stunned myocardium |
|
Dobutamine stress echocardiography
Rationale
Dobutamine is a synthetic catecholamine with both positive inotropic and chronotropic effects mediated predominantly through β 1 -adrenergic receptor stimulation. The principle of DSE is based on detection of inotropic contractile reserve in dysfunctional but viable myocardial segments with dobutamine. At low doses (4 to 8 μg/kg/min), dobutamine is a positive inotrope, but at doses higher than 10 μg/kg/min it exhibits a positive chronotropic effect in addition to the inotropic effect. To assess myocardial viability, only low doses of dobutamine are effective, which produce predominantly inotropy with minimal chronotropy. At higher doses, the increased heart rate resulting from a chronotropic effect will increase myocardial oxygen demand with subsequent ischemia, which is undesirable during testing. , Consequently, low-dose dobutamine is the standard protocol for assessing myocardial viability. However, a biphasic response with higher doses of dobutamine has a higher positive predictive value for the assessment of myocardial viability.
Protocol
Protocols used to assess myocardial viability vary widely, but the most commonly used protocol is to administer dobutamine intravenously, beginning at a dose of 2.5 to 5.0 μg/kg/min and then increasing by 5 to 10 μg/kg/min every 3 to 5 minutes up to a maximum of 40 μg/kg/min, or until a study endpoint is achieved. The endpoints for termination of the dobutamine infusion typically include development of new segmental wall motion abnormalities, attainment of 85% of age-related maximum predicted heart rate, or the development of significant adverse effects related to the dobutamine infusion. Five standard echocardiographic views are obtained with each acquisition: parasternal long-axis, parasternal short-axis, apical four-chamber, apical three-chamber, and apical two-chamber views. Echocardiographic images are acquired at baseline, with each stage of stress, and during the recovery phase. Cardiac rhythm is monitored throughout the stress echocardiography protocol, and 12-lead electrocardiograms and blood pressure measurements are obtained at baseline, at each stage of stress, and during the recovery phase.
There are four characteristic responses of dysfunctional myocardial segments with dobutamine infusion (see Chapter 57 , Fig. 57.2 ):
- 1.
Monophasic (sustained) response: Improvement is seen at low dose, and it persists or further improves at high dose. This indicates viable myocardium with no stenosis of the coronary artery subtending the dysfunctional segment/region.
- 2.
Biphasic response: Function is augmented at low dose and is followed by deterioration at high dose. This indicates the presence of viable myocardium, but the coronary artery that supplies the myocardium has flow-limiting stenosis.
- 3.
Ischemic response: Function deteriorates, without CR. This indicates the presence of myocardium with stress-induced ischemia due to flow-limiting stenosis.
- 4.
Nonphasic response: No change is seen. This indicates the presence of scarred myocardium with no viability.
A contractile response to dobutamine requires that at least 50% myocytes in a given segment are viable, and it correlates inversely with the extent of interstitial fibrosis on myocardial biopsy. Atropine may be given with dobutamine to enhance the diagnostic value of the technique in the assessment of ischemia. Diagnostic yield of DSE may be improved by combining it with other recently developed techniques, including myocardial contrast echocardiography and strain rate imaging with tissue Doppler techniques. In addition, administration of nitroglycerin as an adjunct to dobutamine may enhance accuracy in detecting hibernating myocardium.
Prognostic value
The identification of myocardial viability by low-dose DSE has prognostic significance in patients with ischemic cardiomyopathy. In a study by Chaudhry and colleagues, the prognostic implications of myocardial CR were evaluated in patients with coronary artery disease and left ventricular (LV) dysfunction (EF of 40% or less). In patients undergoing medical therapy alone, those with CR (identified by low-dose DSE) had a better initial survival compared with those without CR, but this was not maintained beyond 3 years. In patients undergoing revascularization, those with CR had better survival compared with those without CR ( Fig. 61.3 ). By multivariate analysis, the number of dysfunctional segments demonstrating CR was the strongest predictor of survival. Thus myocardial viability as determined by low-dose DSE is a significant predictor of survival in patients with CAD and LV dysfunction undergoing either medical therapy or revascularization, independent of symptoms, baseline LV function, or coronary anatomy (see Fig. 61.3 ).

Several other studies have evaluated the usefulness of low-dose DSE in predicting improvement in regional contractile function following revascularization. Cusick and co-workers evaluated the utility of DSE in patients with severe LV dysfunction and showed similar accuracy ( Fig. 61.4 ). Pooled analysis of studies show that low-dose DSE has good sensitivity (81%) and specificity (80%) for predicting improvement of regional and global LV function following revascularization. It thus has a good positive predictive value (77%) and an excellent negative predictive value (85%) ( Figs. 61.5 and 61.6 ).


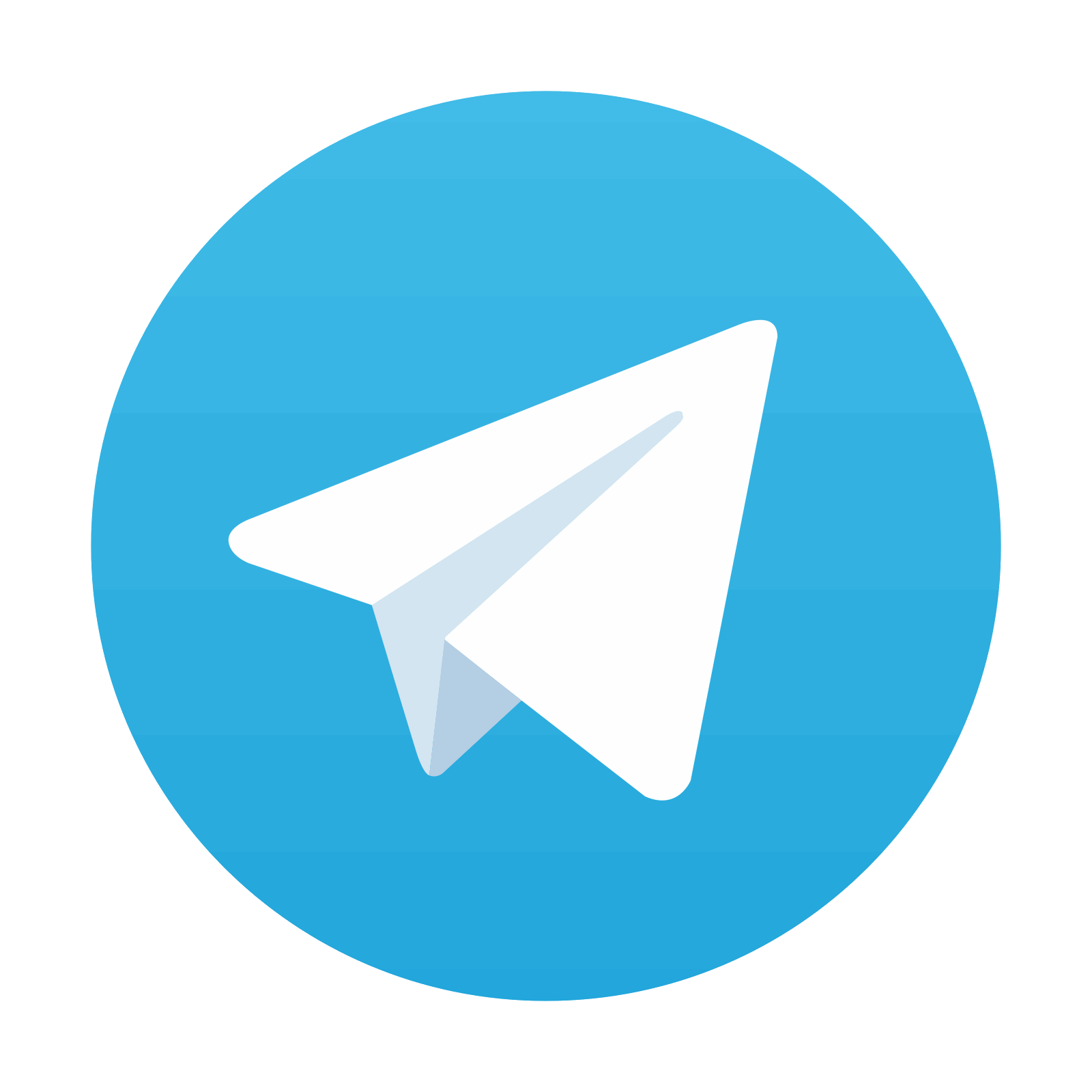
Stay updated, free articles. Join our Telegram channel

Full access? Get Clinical Tree
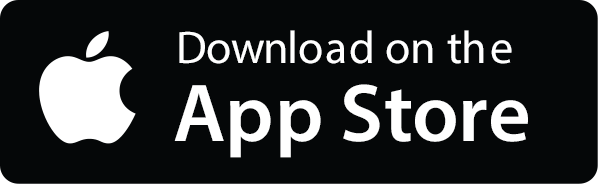
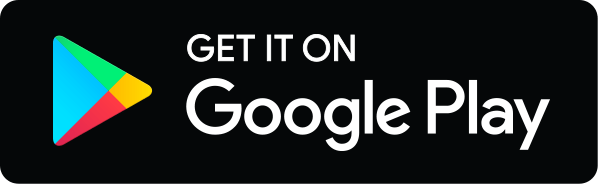
