Stereotactic Techniques for Lung Cancer Treatment
Robert D. Timmerman
Timothy D. Solberg
Brian D. Kavanagh
Simon S. Lo
Historically, the term stereotactic is related to the correlation of tumor target position to reliable fiducials position that could be readily identified from various imaging platforms.1 Radiation treatments guided by such fiducials were referred to as stereotactic radiation therapy. Fiducials define a coordinate system that can be used to target the tumor, orient the treatment planning process, and ultimately guide the therapy toward the intended location in the body. These fiducials were typically placed in a rigid frame outside the patient.2 Today, with sophisticated real-time image guidance, the tumor itself can serve as the fiducial negating the need for external markers that have classically characterized stereotactic treatments.3,4,5,6 In the end, modern stereotactic radiotherapy is a little about “stereotaxy” and a lot about dose fractionation, target definition, motion control (four-dimensional [4D] therapy), image guidance, conformal and compact dose distributions, and high levels of quality assurance in treatment conduct.7 Irrespective of the current significance of the term “stereotaxy,” modern treatments to lung tumors using such technology intensive techniques and giving less than five potent treatments are referred to as stereotactic body radiation therapy (SBRT).8,9
SBRT, or synonomously stereotactic ablative radiotherapy (SABR), is biologically unique from conventional fractionated radiation therapy (CFRT). Whereas CFRT is typically administered in small daily doses in the range of 1.8 to 2.0 Gy to total doses of 60 to 70 Gy, SBRT is intended to be ablative. These ablative treatments are very potent because considerably higher doses per fraction are applied, generally in the range of 10 to 20 Gy per fraction. Biologically, affected cells are both unable to divide and unable to perform intended cellular functions (e.g., secretion, transport oxygen, etc.). Such high doses per treatment were historically out of bounds because limitations in treatment delivery technology did not allow the radiation oncologist to avoid catastrophic toxicity when large volumes of normal tissues were exposed to so much radiation each treatment. With improvements in tumor imaging, image guidance, computerized dosimetry, and treatment delivery devices, such very large dose-per-fraction treatments are no longer out of bounds. Indeed, they are showing dramatic utility for their ability to safety eradicate cancer.
SBRT has been shown to be particularly effective in eradicating the primary tumor in early stage lung cancer.10,11,12,13,14,15,16,17 SBRT offers an elegant, noninvasive, and highly efficient treatment option that is preferred by patients because of its convenience. SBRT has not been generally used in patients with lymph node metastases, however, because of difficulty in targeting the entire extent of tumor as well as toxicity concerns relating to the central chest. In metastatic lung cancer, SBRT may be used as an ablative or cytoreductive agent in selected patients.
Because of its normal-tissue dose sparing facilitated by the unique dose distribution and imaging technology, frail patients can tolerate the treatment surprisingly well. It is considered a standard treatment in such patients with stage I non-small cell lung cancer (NSCLC).18 Despite its ability to treat the frailest patients, SBRT should still be viewed as the most potent radiation treatment with clinical experience in fighting gross tumor deposits. Local control with SBRT has been shown to be dramatically superior to historical controls using CFRT for early stage lung cancer. Indeed, local control with SBRT rivals surgical resection for most indications. Limitations definitely exist as will be discussed in this chapter. With careful clinical testing and implementation, SBRT is finding a prominent place within the cancer treatment arsenal.
DEVELOPMENT OF STEREOTACTIC BODY RADIATION THERAPY
The success of brain radiosurgery pioneered by Swedish neurosurgeon Lars Leksell 1 forms the basis of SBRT. Leksell not only developed what would become the modern day Gamma Knife, he departed from the current practice of the time to deliver radiation in a protracted fractionation schedule. With the newly developed technology, large dose-per-fraction treatments given in a single treatment at dose levels that would have historically resulted in considerable calamity were able to be routinely
delivered in the dose intolerant brain. The new treatment conduct went to great lengths to avoid prescription level dose to normal tissues. Whatever normal tissue was included, either by being adjacent to the target or by inferior dosimetry, was likely damaged. However, if this damaged tissue was small in volume or noneloquent, the patient did not suffer clinically apparent toxicity, even as a late event. On the other hand, it is undeniable that the large dose-per-fraction treatments are biologically extremely potent by overwhelming repair mechanisms.19 Targeted tumors are disabled and toxicity is avoided by simply missing most normal tissue.
delivered in the dose intolerant brain. The new treatment conduct went to great lengths to avoid prescription level dose to normal tissues. Whatever normal tissue was included, either by being adjacent to the target or by inferior dosimetry, was likely damaged. However, if this damaged tissue was small in volume or noneloquent, the patient did not suffer clinically apparent toxicity, even as a late event. On the other hand, it is undeniable that the large dose-per-fraction treatments are biologically extremely potent by overwhelming repair mechanisms.19 Targeted tumors are disabled and toxicity is avoided by simply missing most normal tissue.
Hamilton et al.20 performed the earliest examples of treatments mimicking the stereotactic radiosurgery (SRS) treatments outside of the brain. These treatments employed the same rigid immobilization principles of SRS in the spine by screwing a frame to the spinous processes. Although reports were encouraging, the conduct of the treatment was not as gratifying as natural and inherent motion confounded accuracy. The brain can be practically immobilized by immobilizing the skull. Once the skull is immobilized, targets within the brain have very little additional movement. Such is not the case outside of the skull. Tumors in the body may be displaced as a function of time by forces exerted by muscle contraction, breathing, gastrointestinal peristalsis, cardiac activity, and many other important physiological processes. It is not possible to eliminate or account for all of these forces. As a result, SBRT is inherently less accurate than SRS.
As with the Gamma Knife, researchers again from Sweden, Ingmar Lax and Henric Blomgren, set out to overcome these problems by constructing a body frame that would both comfortably immobilize the patients torso as well as dampen the internal motion relating to respiration.21,22 Subsequently, they treated patients with localized tumors using dosimetry plans that mimicked SRS.23 The dosimetry was constructed using multiple noncoplanar beams with aperture dimensions on the order of the target dimensions. Each of the many beams carried relatively lower weight than with CFRT such that the target dose at the convergence could be dramatically escalated. Blomgren and Lax treated patients with mostly metastases initially. Local tumor control was better than expected, leading them to treat more limited stage cancer patients. Blomgren et al.24 shared their results via publications and eventually trained others in this new technique.
Meanwhile, investigators from Japan were exploring radiosurgery-like treatments in the chest. Shirato et al.25 pioneered investigation into characterization and accounting of respiratory motion. Initially, they used CFRT dose schedules, but the understanding of target motion control was very important for the ultimate feasibility of SBRT, as it currently exists. Uematsu et al.26,27,28 again from Japan worked in the early 1990s on developing technologies for delivering multiple focused beams of radiation for extracranial targets. In addition, Uematsu’s group treated patients with lung tumors primarily from primary non—small cell cancer.
Prospective testing of SBRT began with acquisition of the technology by the groups from the University of Heidelberg, University of Wurzburg, Kyoto University, and Indiana University.11,14,29,30 Initially, dose-escalation toxicity studies were carried out in the liver and lung trying to find the most potent dose schedules for typically radioresistant primary and metastatic tumors. These prospective trials have been reported but are still maturing and will add a wealth of understanding for the use of SBRT. It is already clear that local tumor control will be higher with SBRT than has been observed with CFRT. SBRT is now a standard treatment for patients with peripheral tumors from early stage lung cancer with simultaneous medical problems precluding surgery. However, toxicity from large dose-per-fraction radiation schedules often appears quite “late” from time of treatment. Therefore, the total impact of treatment, good and bad, will require many years of follow-up to fully appreciate.
DEFINITIONS AND NOMENCLATURE OF STEREOTACTIC BODY RADIATION THERAPY
SBRT is best defined by its conduct. The proper performance of SBRT requires a team approach to carry out the following tasks31:
Secure yet comfortable immobilization avoiding patient movement for the typical long treatment sessions.
Accurate repositioning of the patient from planning sessions to each of the treatment sessions.
Proper accounting of inherent internal organ motion, including breathing motion consistently between planning and treatment.
Construction of dose distributions confidently covering tumor and yet falling off very rapidly to surrounding normal tissues. The dosimetry must be extremely conformal in relation to the prescription isodose line compared to the target outline but may allow very heterogeneous target dose ranges.
Registration of the patient’s anatomy, constructed dosimetry, and treatment delivery to a three-dimensional coordinate system as referenced to fiducials. Fiducials are “markers” whose position can be confidently correlated to both the tumor target and the treatment delivery device. A “stereotactic” treatment is one directed by such fiducial references.
Biologically, potent dose prescriptions using a few (i.e., one to five) fractions of very high dose (e.g., generally a minimum of 6 Gy per fraction but often as high as 20 to 30 Gy per fraction).
A working group from the American College of Radiology and American Society for Therapeutic Radiology and Oncology had formulated guidelines for the conduct of SBRT.8 In general, SBRT is used to treat well-demarcated visible gross disease up to 5 to 7 cm in dimension. It is not used for prophylactic (adjuvant) treatment, as the intent is to totally disrupt clonogenicity and likely disrupt all cellular functioning of the target tissues (i.e., the definition of an ablative therapy).32,33,34,35,36
Tissues exposed to the prescription dose level or nearby are likely to be ablated. Such a treatment, properly directed would constitute a most potent form of cancer therapy. In turn, if misdirected or used too liberally, SBRT could lead to debilitating toxicity. At any rate, SBRT is very different from conventionally fractionated radiation therapy (CFRT) in its conduct, toxicity, and ability to control cancer. To this end, it is clear that all forms of radiotherapy do not constitute a black box.
MOTION ISSUES FOR LUNG STEREOTACTIC BODY RADIATION THERAPY
Treatment plans are generally static with no specific accounting of motion that is typical in the treated patients. Still, the geometry and dose distribution from the radiation therapy treatment plan should be a reasonably true characterization of what is actually delivered to the patient. With the typical large volume of treatment and homogeneous dose-distributions characteristic of CFRT, such an emphasis on the proper correlation of the treatment plan and actual treatment is probably not so critical. However, for SBRT, claims regarding accuracy of equipment, quality of dose distributions, and dose tolerance should not be made based on the virtual computer simulation of the treatment plan; rather, on actual delivery of dose to treated patients. This is particularly true for predicting normal-tissue toxicity from SBRT where both heterogeneous dose and differential volume effects may equally affect outcome.
Although there is a general fascination within the radiotherapy community for electronic or sophisticated mechanical solutions to motion, passive immobilization can be extremely effective. Body frames, vacuum pillows, thermal plastic restraints, and other equipment have been used to try to achieve relocalization similar to the position of simulation,22,37,38,39,40,41,42,43,44,45,46,47,48,49 Other systems will effectively relocate a reference position within the patient prior to each treatment, without the aid of frames or other immobilization devices (i.e., “frameless” systems).27,50,51,52 Both approaches have advantages and disadvantages, and no clearly superior method has been identified in clinical practice. In the end, it is most critical to be practical. SBRT treatment sessions are longer than CFRT sessions. Hence, it is important that the positioning system be comfortable and avoids awkward positions or positions fighting against gravity. In addition, the system employed must be properly utilized. As such, staff training and properly administered quality assurance programs are more essential than using a particular brand of equipment.
To minimize treatment margins for coverage, tumor (and normal tissue) motion must be characterized, controlled, or compensated. Motion control accounting approaches fall into three general categories: (a) dampening, (b) gating, and (c) chasing. Within the category of dampening includes the systems of abdominal compression aimed at decreasing one of the largest contributors to respiratory motion related to the diaphragm.22,41,44,45,46,49 Also included in this category are the systems employing breath-hold maneuvers to “freeze” the tumor in a reproducible stage of the respiratory cycle (e.g., deep inspiration).53,54,55,56 Gating systems follow the respiratory cycle using a surrogate and employ an electronic beam activation trigger, allowing irradiation to only occur during a specific segment (e.g., end expiration).50,57,58,59 Tracking systems literally move the radiation beam along the same path as the tumor from the beam’s eye view.25,60,61,62,63 Tracking may be accomplished by moving the entire accelerator, the aperture (e.g., with the multileaf collimator), or moving the patient on the couch counter to the motion of the tumor. In the case of gating and breath hold, the beam is triggered on and off, constituting a duty cycle avoided by the other systems. In any case, the acquisition of planning information must include the same consideration for motion accounting as the treatment to achieve accuracy. Despite available motion control equipment, some uncertainty continues to require that planning treatment volume (PTV) is larger than gross tumor volume (GTV). In general, for typical dose prescriptions, this enlargement should not be greater than 1.0 cm in the cranial caudal plane and 0.5 cm in the axial plane.
BIOLOGICAL ASPECTS OF STEREOTACTIC BODY RADIATION THERAPY
Tumor Biology The cell survival curve (logarithm of surviving fraction vs. dose) after exposure to sparsely ionizing radiation, such as photons and gamma rays, follows a characteristic shape starting with a curving portion called the “shoulder” and then becoming linear with increasing dose. With CFRT, daily treatment is given on the shoulder of the survival curve (daily dose range of 1.5 to 3.0 Gy), where cells are able to repair some of the inflicted damage. The survival data in this region can be mathematically “fit” by a second order polynomial to give a simple functional representation. The predictive model related to is called the linear-quadratic (LQ) model.64,65,66 Although this formula had served the field of radiobiology quite well for decades, several authors have pointed out that this formalism is not applicable in the range of high doses applied with SBRT.67,68,69 In effect, the LQ model grossly overestimates the potency of treatment for daily dose delivery beyond the shoulder (i.e., 6 to 8 Gy for most tumor cell lines) caused by erroneous extrapolation outside the area of fit. Guerrero and Li69 have proposed modifying the LQ formula by incorporating features of the so-called lethal-potentially lethal (LPL) model. The LPL model differs from the LQ model primarily insofar as it accounts for ongoing radiation repair processes that occur during the radiation exposure. Marks68 and Park et al.67 have borrowed formalism from the older multitarget model70 to determine a “single fraction equivalent dose” for hypofractionated treatments with daily dose levels greater than 7 to 10 Gy that avoids any use whatsoever of the erroneous LQ model. The net result of more appropriate estimation from tumor cell kill models is a substantial difference in the predicted tumor cell kill at SBRT-level doses. For example, for a dose of approximately 20 Gy, the LQ
model would erroneously predict several orders of magnitude greater cell kill than the LPL model.69
model would erroneously predict several orders of magnitude greater cell kill than the LPL model.69
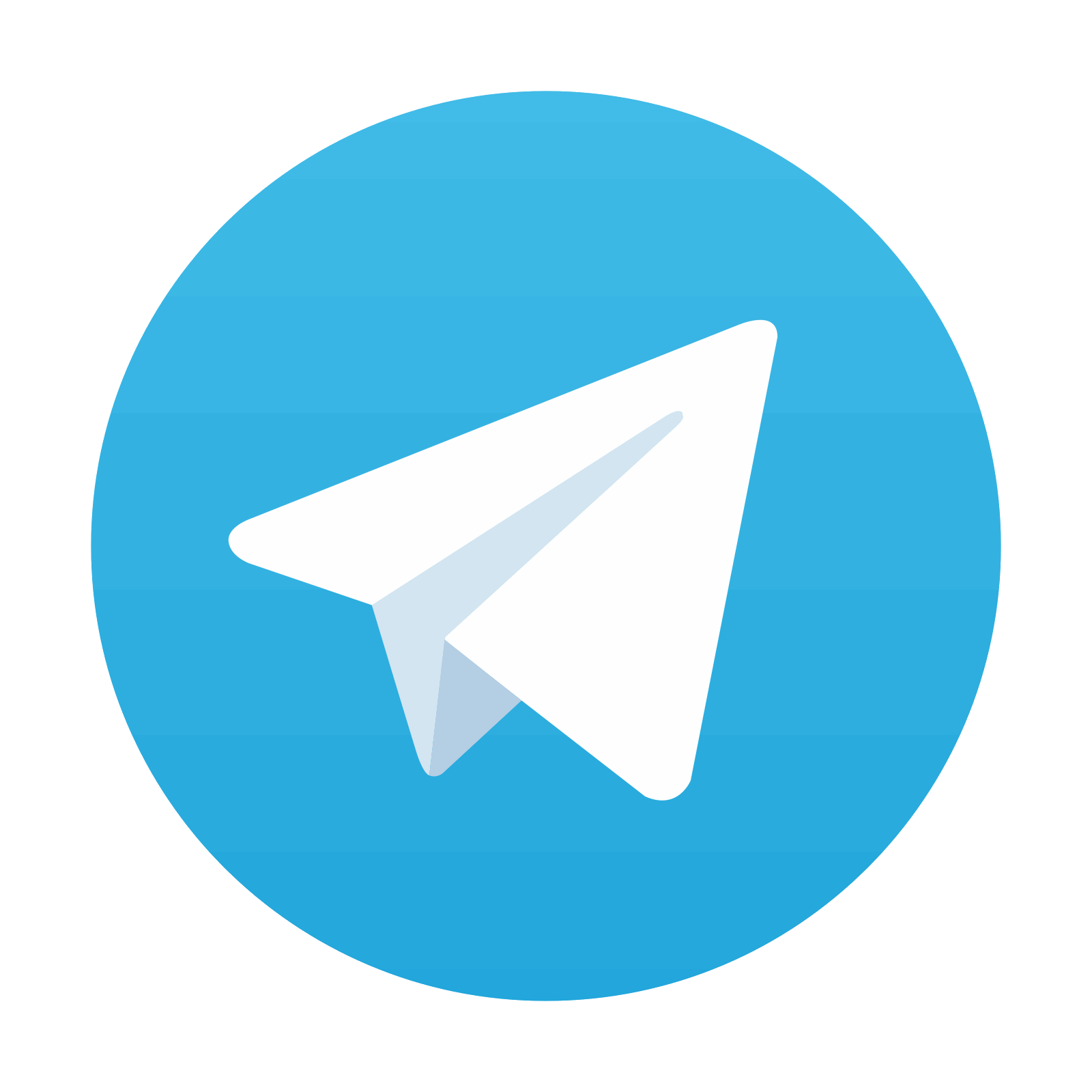
Stay updated, free articles. Join our Telegram channel

Full access? Get Clinical Tree
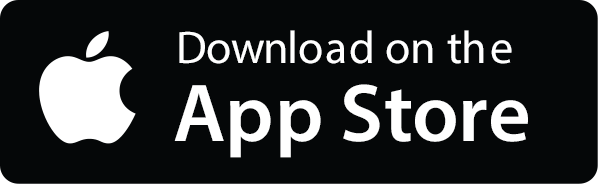
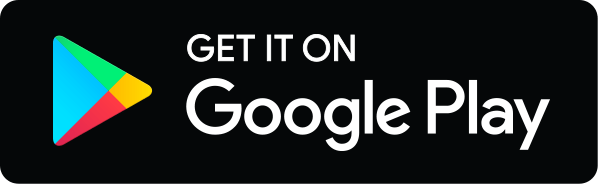