Fig. 7.1
Stem cells of the distal bronchiolar airways. (a) Murine distal airways are primarily composed of ciliated, secretory (Club), and neuroendocrine cells. Lineage tracing studies using genetically modified mouse models have shown that Club cells function as the predominant stem cell population that maintains normal airway homeostasis (light grey cells). NEB- and BADJ-associated, variant Club stem cells (NASCs and BASCs) additionally exhibit enhanced resistance to airway damage and contribute to lung regeneration following severe injury (dark grey cells). (b) Human distal airways contain abundant secretory (Club and goblet), ciliated, and basal epithelial cell types. In contrast to murine lungs, ex vivo and in vivo evidence supports a likely role for basal cells as human distal bronchiolar stem cells. Here, basal cells are thought to maintain both airway homeostasis and repair /regeneration capacities (light grey cells)
In addition to their contribution to airway homeostasis, previous severe injury, and repair studies also identified subpopulations of Club cells that contribute to lung regeneration . The most frequently used severe bronchiolar injury model involves intraperitoneal or aerosolized delivery of naphthalene, a derivative of coal tar [27–30]. Naphthalene administration causes significant, Club cell-specific toxicity in murine airways [31]. The extent and severity of this injury can be experimentally determined through both dose and route of administration [29, 30]. The cause of Club cells’ unique sensitivity to cytotoxic injury is their expression of cytochrome P450 monooxygenases; this family of metabolic enzymes acts to oxidize potentially damaging exogenous compounds rendering them more water soluble. Specifically, airway Club cells express cytochrome P450 isozyme 2 F2 (CYP2F2) which produces the toxic metabolite 1R, 2S-naphthalene oxide upon naphthalene exposure [28, 32].
Following naphthalene-mediated Club cell ablation, a small number of naphthalene-resistant Club cells (termed variant CCSP-expressing cells; vCE cells) survive by virtue of their reduced expression of CYP2F2. These vCE cells subsequently proliferate and regenerate all differentiated distal bronchiolar cell types [33, 34]. These phenotypically variant stem cells are typically found in association with both neuroepithelial bodies (NEBs) and at bronchiolar duct junctions [25, 33, 35]. In addition, a population of bronchiolar duct junction-associated vCE cells has been shown to express both CCSP and surfactant protein-C (SPC) [36]. Lineage tracing experiments involving these cells, termed bronchioalveolar stem cells or BASCs, demonstrate that they are capable of differentiation into both bronchiolar and alveolar epithelial cell types following certain types of lung injury [24, 37].
In addition to normal and variant Club cells, recent studies identified a population of p63-expressing basal-like cells that contribute to distal lung repair following influenza infection [38]. At the peak of influenza infection, the abundance of these p63+ basal cells increased dramatically and resulted in restoration of a phenotypically normal airway with abundant Club cells [38]. Subsequent work by this same group demonstrated that p63-expressing cells were in fact an essential component of normal distal airway regeneration post-influenza infection [39]. These studies, along with recent complementary evidence of dedifferentiation of CCSP+ cells into upper airway basal stem cells , suggest a previously unappreciated lineage plasticity among cells that survive epithelial injury [40, 41].
In contrast to murine airways, CCSP-expressing cells are significantly less abundant in human airways [9, 42] and a combination of both Club and basal cells are present in human distal bronchioles [43]. This suggests that either CCSP-expressing cells or basal cells might function as stem cells in human bronchioles [44]. In vitro studies using human cells support the hypothesis that keratin 5 and/or keratin 14-expressing basal cells might function as bronchiolar stem cells in human lungs [38]. Further studies by Teixeira et al. provide quantitative analysis of distal bronchiolar cell types within intact human airways [43]. These results suggest that basal cells are abundant and widely distributed human airway stem cells (Fig. 7.1b). It is as yet unclear whether these basal stem cells responsible for human airway homeostasis and regeneration are equivalent to the keratin-5 and keratin-14-expressing p63+ cells associated with post-influenza lung regeneration in mice [38, 39]. The relative contribution of CCSP-expressing Club cells and keratin 5-expressing basal cells to human distal lung homeostasis and repair also remains to be determined.
Recently, expression of Wnt-responsive genes such as leucine-rich repeat-containing G-protein coupled receptor 5 (Lgr5) and Lgr6 have been found in rare stem cells in a broad range of epithelial organs [45], including the lung [46]. Following these discoveries, a discrete population of E-Cadherin+/Lgr6+ cells was isolated from human small bronchioles [47]. Reminiscent of murine BASCs, single cells were capable of significant expansion in vitro and generated differentiated bronchioalveolar cell types when injected under the kidney capsule of immune-deficient mice [47]. The relationship of these cells to other known stem cell populations in either mouse or human lungs and their relative importance to in vivo homeostasis and repair will be an important question in future studies.
Ex Vivo Analyses of Distal Bronchiolar Stem Cells
Investigation of the growth and differentiation properties of prospectively isolated airway cells ex vivo represents an alternative strategy for the identification of distal bronchiolar stem cells [48, 49]. These studies establish the value of ex vivo lung stem cell cultures, with high correlation between in vitro stem cell characteristics and the endogenous behaviour of these cells in human and murine airways [50, 51]. The continued improvement of these protocols for lung stem cell isolation, expansion, and differentiation will not only reduce reliance on animal models but also provide a novel means to interrogate the relevance of stem cells in human lung disease.
Initially, murine lung stem cells were isolated from intact lungs using the cell surface markers Sca-1 and CD34 [36]. These cells expressed CCSP and SPC, were capable of in vitro self-renewal and exhibited multipotent differentiation into Club-like cells as well as type I and II alveolar epithelial cells. Thus, dual Sca1 and CD34-positive cells appear to represent an ex vivo equivalent of terminal bronchiole-associated BASCS [36]. Despite this, the purity and homogeneity of these prospectively isolated cells has been contested, in that the cell surface markers Sca-1 and CD34 additionally isolate non-BASC cell types [52–54]. It is similarly unclear how these findings might inform the prospective isolation of human distal bronchiolar stem cells given the lack of a human homologue for Sca-1 [55].
In separate studies, McQualter and colleagues determined that a population of CD24low/integrin α6β4+/EpCam+ cells were capable of multilineage differentiation [56]. These cells represent a non-ciliated population of airway cells with properties consistent with CCSP+ stem cells . Specifically, co-culture of isolated integrin α6β4+/CD24low cells with lung -derived mesenchymal cells produces differentiated epithelial organoids with both bronchiolar Club and ciliated cell populations [56]. Here, mesenchyme-derived FGF10 was identified as an important mediator of epithelial stem cell expansion in vitro [56, 57]. This was consistent with evidence that parabronchial smooth muscle-derived FGF10 maintains vCE cell populations in vivo [58, 59]. As above, it remains to be determined whether human lungs contain an equivalent cell type with characteristics similar to these integrin α6β4+/CD24low cells.
The Distal Bronchiolar Stem Cell Niche
A detailed understanding of the mechanisms that regulate airway stem cell behaviour will be necessary in order to use these cells for improving human lung health. In vivo, stem cells exist in a complex three-dimensional (3D) microenvironment , the “stem cell niche ,” which is finely tuned to generate the signals required to balance self-renewal and differentiation [60–62]. Thus, the stem cell niche functions as a dynamic system that integrates specific extracellular components, growth factors , signalling molecules, biomechanical properties, and spatial constraints that direct and determine cellular fate and phenotype [63]. While the niche of some stem cells has been studied extensively (such as that of intestinal or hematopoietic stem cells [64, 65]), the specific signals that regulate distal bronchiolar stem cells are less well understood.
In murine airways, vCE cells located adjacent to neuroepithelial bodies (NEBs) and bronchioalveolar duct junctions (BADJs) regenerate the airways following injury making these anatomical locations putative airway stem cell niches [33, 35, 36]. In support of this, epithelial cells in close proximity to pulmonary neuroendocrine cells and neuroepithelial bodies (NEBs) can exhibit increased proliferation [66, 67]. Similarly, in adult mice exposed to naphthalene, surviving vCE cells are co-localized with neuroendocrine cells located at airway branch points [35, 68]. Separately, the bronchioalveolar duct junction represents a second stem cell niche identified in distal bronchiolar airways [33, 36]. Here, neuroendocrine cells are largely absent, suggesting that other cell populations regulate BASC activation [33]. It is as yet unclear whether neuroepithelial bodies or terminal bronchioles serve a similar role in maintaining populations of injury-resilient stem cells in human conducting airways .
In recent years, studies have determined that the regulation of stem cells within their niche is coordinated through both intrinsic and extrinsic mechanisms [63]. Specific components of niche–stem cell coordination include stem cell–basement membrane interactions (governed by extracellular matrix stiffness and composition), cell–cell interactions (including direct communication and contact inhibition-mediated cell proliferation and signalling), and regulation via local and systemic secreted components, inflammatory and immune cells, and pathogenic stimuli (Fig. 7.2, [61, 69–71]). These are likely to be coordinated through pattern recognition receptors (such as toll-like receptors), cell adhesion molecules normally involved in regulation of adherens and tight junctions (such as cadherins, junctional adhesion molecules (JAMs) and other molecules including nectins and nectin-like molecules), receptor tyrosine kinases (RTKs), G-protein coupled receptors (GPCRs), and cytokine receptors. In turn, this coordinated signalling is thought to regulate cell homeostasis, proliferation, apoptosis, senescence , and motility via several downstream networks. Although incompletely characterized, these are known to include the PI3K/Akt, Erk/MAPK, canonical and non-canonical beta-catenin, Notch-Delta, Jak/Stat, and Hedgehog signalling pathways [72–74].
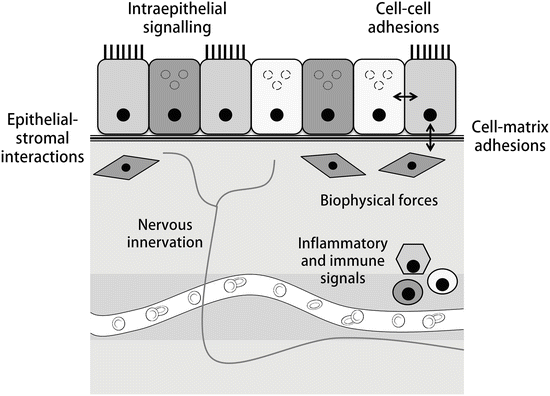
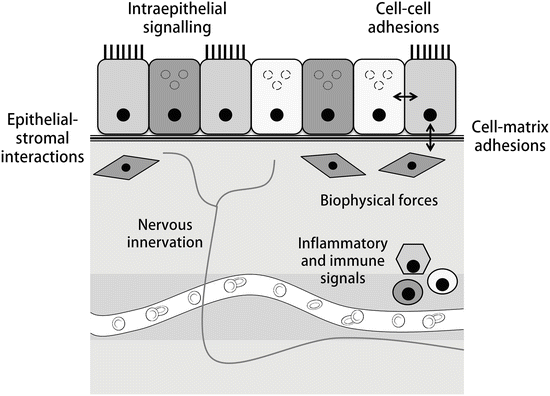
Fig. 7.2
Components of an epithelial stem cell niche . The niche, or local microenvironment where stem cells reside, functions as a dynamic system that integrates local, systemic, and cell-intrinsic signals to determine cellular fate and phenotype [60]. Local signals include interactions among and between cells and their extracellular matrix as well as intra-epithelial and bidirectional epithelial-stromal signalling cascades [63]. In turn, these signals both influence and respond to biophysical components of the niche including matrix stiffness, composition, local tension, and microenvironment shape. In addition, signals from nearby inflammatory and immune cells, nervous innervation, and the local circulation are known to influence stem cell phenotypes
In vivo, bronchiolar stem cell behaviour is determined by multiple niche -derived signalling networks [75, 76]. In particular, it was shown that stabilization of beta-catenin activity in bronchiolar stem cells promotes generation of a hyperplastic epithelium and expansion of naphthalene-resistant stem-like cells [77]. Similarly, ablation of Gata6, a negative regulator of the Wnt receptor Frizzled2, causes loss of epithelial differentiation and increased BASC abundance [78]. In each case, bronchiolar stem cell beta-catenin activity was governed by stromal cell-derived local Wnt and/or Fgf secretion [58, 59, 77, 78].
Notch signalling also impacts upon the differentiation status of the distal airway . Activation of canonical Notch signalling in developing murine airways results in goblet cell metaplasia and reduced numbers of ciliated cells [79]. Loss of Notch signalling in Hes1−/− mice reduces the number of Club cells due to an increase in the proportion of cells that differentiate as pulmonary neuroendocrine cells during lung development [80]. In addition, naphthalene-mediated Club cell ablation is fatal in Notch1 mutant mice due to an inability to efficiently repair the damaged epithelium [81]. Loss of Notch signalling in adult mice through conditional inactivation of Pofut1 reduces Club cell number and increases the abundance of ciliated cells [82]. The depletion of Club cells in the absence of Notch signalling may arise as a consequence of lateral inhibition from ciliated cells that prevents neighbouring cells from adopting a Club cell phenotype [83]. Overall, these studies indicate that low levels of Notch favour differentiation towards ciliated and neuroendocrine lineages whereas elevated Notch signalling promotes Club and goblet cell differentiation via SAM-pointed domain containing ETS transcription factor (SPDEF) activation [84, 85]. Interestingly, misexpression of the active Notch intracellular domain in the proximal airway epithelium causes goblet cell metaplasia and reduced numbers of ciliated cells in the proximal but not distal airways [79], suggesting that the effects of Notch signalling are both spatially and temporally dependent.
In murine models, the vasculature is also important in driving signalling pathways that control lung regeneration . In a unilateral pneumonectomy model, matrix metalloproteinase 14 (MMP14) produced by endothelial cells causes increased release of epidermal growth factor (EGF) protein family ectodomains to stimulate epithelial regeneration [86]. Recently, a novel endothelial cell-derived signalling axis was found to influence the fate of BASCs [71]. Primary murine lung endothelial cells were able to support multiple passages of BASCs with bronchiolar and alveolar differentiation capacity in 3D culture [71]. Thrombospondin1 (TSP1)-deficient endothelial cells tip the balance of BASC differentiation in favour of bronchiolar cell types, suggesting that TSP1 is an endothelial-derived factor which promotes alveolar differentiation of BASCs [71]. Addition of recombinant BMP4 to BASC cultures produced the reverse effect in a TSP1-dependent manner, favouring alveolar differentiation [71]. Thus BMP4, likely produced by epithelial cells [87], induces TSP1 expression in endothelial cells via the calcineurin-NFAT pathway, which in turn promotes alveolar differentiation of murine distal lung stem cells .
Unfortunately, the signalling pathways and factors that regulate stem cell activity in human lungs remain only partially characterized. Despite this, studies of prospectively isolated Lgr6+ human lung stem cells injected under the murine kidney capsule have identified endogenous cells and factors that are involved in lung stem cell growth and differentiation [47]. Expression of the cytokine stromal cell-derived factor 1 (SDF-1) in transplanted stem cells activates and recruits stromal fibroblasts [88]. These fibroblasts secrete tumour necrosis factor α (TNFα), which provides an activating signal for lung stem cells to produce more TGFβ and consequently more SDF-1. Furthermore, endothelial cells were recruited to the transplantation site in a process dependent on secretion of interleukin 8 (IL-8) and vascular endothelial growth factor (VEGF) by activated fibroblasts [88]. While the relevance of these ex vivo findings to the native human lung stem cell niche remains unclear, these data support the aforementioned studies indicating the importance of endothelial signals in the murine BASC niche [71].
Distal Stem Cells in Lung Cancer
As well as their role in homeostasis, repair , and regeneration , there is now agreement that many types of epithelial tumours originate from single, dysfunctional stem cells [89]. This is consistent with the unique properties of tissue stem cells including their capacity for long-term self-renewal and generation of extensive progeny [90–92]. In the lungs, there is a good correlation between stem cell niches and the sites at which lung tumors are most commonly observed [93].
In distal airways, alveolar type II cells have traditionally been considered a likely cell of origin for lung adenocarcinomas based on the observation that this tumor type often expresses abundant surfactant protein C [94]. Indeed, in murine models of K-Ras-induced adenocarcinoma, recombination with SPC-CreER or CCSP-CreER was observed in both type II cells and BASCs but tumors were only observed in the alveoli [94, 95]. However, the BASC subset expressing both CCSP and SPC expands during tumorigenesis, suggesting that this population of cells may represent an alternative cancer initiating cell [36]. Recent attempts to clarify this problem have used CCSP-Cre and SPC-Cre adenoviruses to initiate K-Ras-dependent tumorigenesis [96] or induced recombination from more wide ranging promoters such as polr2a [97]. The former study showed CCSP-Cre adenovirus administration caused tumorigenesis in both the BADJ and the alveoli but infrequently in the bronchioles [96]. The latter study showed that K-Ras was activated in a range of epithelial cell types including CCSP+, SPC+, and CCSP+/SPC+ populations but that the development of adenocarcinomas occurred exclusively in the alveoli [97]. More of these BADJ lesions, some of which progressed to invasive cancer, occurred when intratracheal administration of Cre adenovirus was used to initiate recombination. Altogether, these studies suggest that multiple lung cell types may be capable of giving rise to K-Ras-induced tumours. This finding is consistent with the extensive plasticity observed following severe lung injury as well as the growing appreciation that environmental factors (in particular, immune surveillance via PD-1/PD-L1 signalling) play a dominant role in driving lung cancer initiation and progression [98, 99].
Conclusions and Perspectives
Substantial progress has been made in recent years in improving our understanding of the mechanisms of murine airway homeostasis, repair , and regeneration . The locations and identities of various endogenous airway stem cells are now well established and markers identified to facilitate the prospective isolation and characterization of these various populations. We are also beginning to understand the molecular mechanisms and signalling pathways that govern airway stem cell behaviour in lung health and disease. Despite this, an improved appreciation of how the local microenvironment or niche responds to and directs lung injury and repair remains a key research priority. It is also important to improve our understanding of the identity, location, plasticity, and functional significance of human lung stem cells in health and disease. Answering these questions in a convincing manner is likely to influence the development of new therapies for lung disease and subsequently improve human lung health.
References
1.
Hasleton PS (1972) The internal surface area of the adult human lung. J Anat 112(Pt 3):391–400PubMedCentralPubMed
2.
Rezaee F, Georas SN (2014) Breaking barriers. New insights into airway epithelial barrier function in health and disease. Am J Respir Cell Mol Biol 50(5):857–869PubMedCentralCrossRefPubMed
3.
4.
Massaro GD, Singh G, Mason R, Plopper CG, Malkinson AM, Gail DB (1994) Biology of the Clara cell. Am J Physiol 266(1 Pt 1):L101–L106PubMed
5.
6.
Parker D, Prince A (2011) Innate immunity in the respiratory epithelium. Am J Respir Cell Mol Biol 45(2):189–201PubMedCentralCrossRefPubMed
7.
Rock JR, Onaitis MW, Rawlins EL, Lu Y, Clark CP, Xue Y et al (2009) Basal cells as stem cells of the mouse trachea and human airway epithelium. Proc Natl Acad Sci U S A 106(31):12771–12775PubMedCentralCrossRefPubMed
8.
Rock JR, Randell SH, Hogan BL (2010) Airway basal stem cells: a perspective on their roles in epithelial homeostasis and remodeling. Dis Model Mech 3(9–10):545–556PubMedCentralCrossRefPubMed
9.
Boers JE, Ambergen AW, Thunnissen FB (1999) Number and proliferation of clara cells in normal human airway epithelium. Am J Respir Crit Care Med 159(5 Pt 1):1585–1591CrossRefPubMed
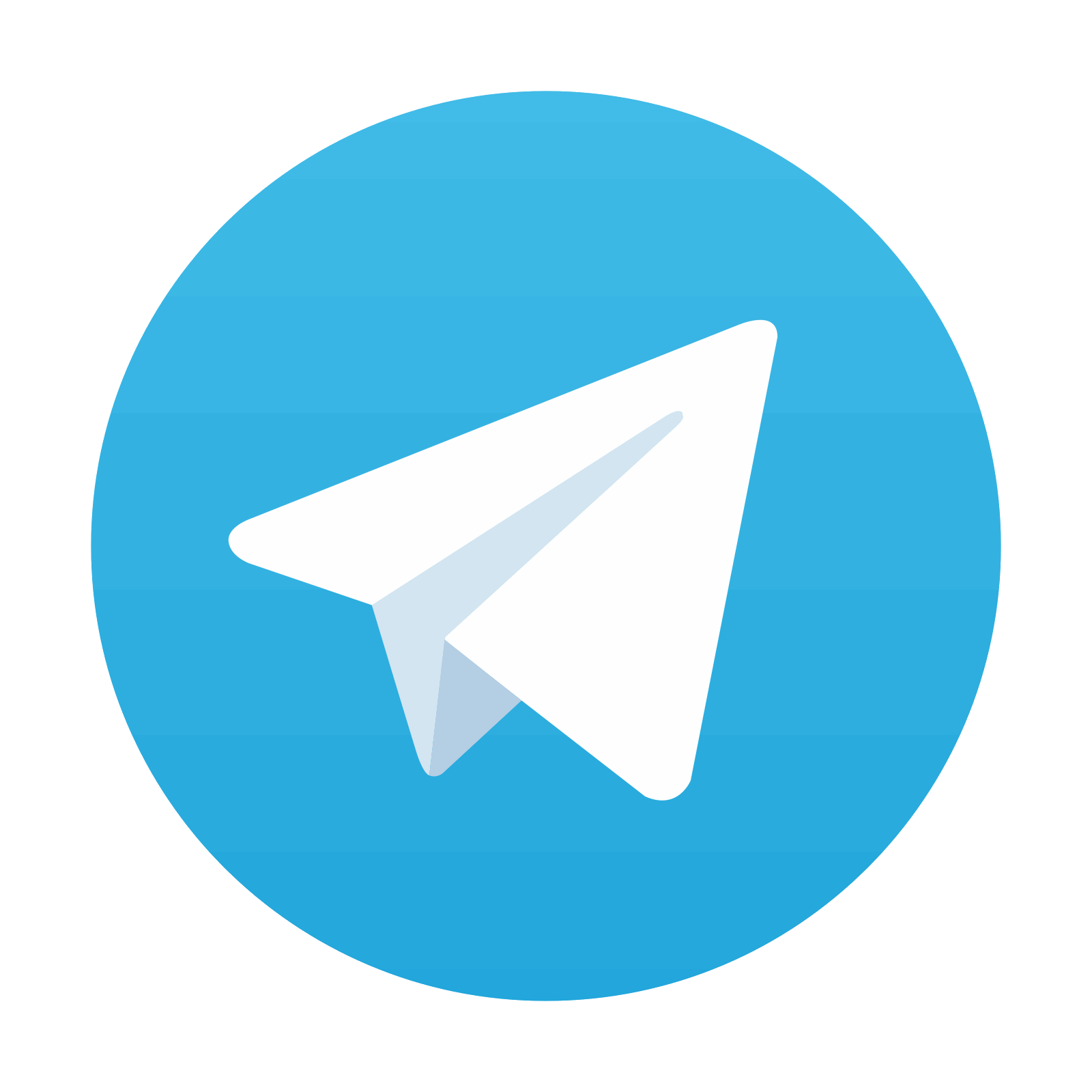
Stay updated, free articles. Join our Telegram channel

Full access? Get Clinical Tree
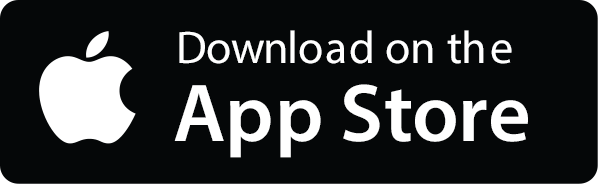
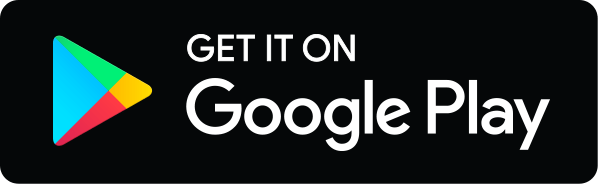