Fig. 6.1
Schematic representation of our current understanding of stem and progenitor cells responsible for maintenance and repair of the proximal airway surface epithelium and submucosal glands. LRC label retaining cells, ALDH aldehyde dehydrogenase, MEC myoepithelial cells, K keratin, SMA smooth muscle actin
Different Stem Cell Subtypes Within the Airway Basal Cell Pool
Basal cells in several organs and tissues express Trp-63 (p63), cytokeratins (K)5 and K14 [13]. Airway basal cells were thought to constitutively express K14 [14] like basal cells of other epithelia, but this was later shown not to be the case [15, 16]. Naïve mouse tracheal basal cells express K14 in only 10–20 % of cells [16, 17] while in apparently healthy human bronchi K14 is expressed in only 1–2 % of cells [18]. K14 is also expressed in the basal and myoepithelial cells (MEC) of the SMG of both human and mouse [17, 18], as discussed below. However, after various injuries and/or infection and with chronic inflammation K14 expression is upregulated in many basal cells [17–19].
The functional role of K14 upregulation after injury and in pathological conditions is not clear. A recent study of stratified epithelium showed that loss of K14 expression was associated with reduced cell proliferation and delay in cell cycle progression [20]. As naïve airway basal cells proliferate very slowly, the need to upregulate K14 expression would be understandable in the context of “activation” of an otherwise-dormant basal stem cell to quickly proliferate in response to injury (Fig. 6.1). Whether K5+/K14− and K5+/K14+ represent two different subtypes of basal cell with some degree of plasticity, or whether they are the same cell in a different state of activation is still controversial [16].
Evidence that basal cells are the “facultative” stem cells of the proximal airways and that they maintain and repair the proximal airways after injury has accumulated over many years [21, 22]. The most concrete characterization of their behavior as stem cells comes from lineage tracing studies. Engelhardt et al. performed a thorough study of the ability of the human proximal airway epithelium to reconstitute a denuded tracheal xenograft [23]. Epithelial cells were color-labeled using retroviral vectors so that the resulting clone sizes and differentiation potential could be followed. They observed that approximately 45 % of clones were multipotent, giving rise to basal, intermediate, secretory, and ciliated cells [23]. This finding is consistent with later rigorous lineage tracing experiments using a K5-CreER transgenic mouse model to follow the fate of K5+ tracheal basal cells in vivo during early postnatal growth, adult tracheal homeostasis, and tracheal repair after injury [24]. This study showed that basal cells self-renew and differentiate into both Club and ciliated cells, and that a long observation period of up to 14 weeks did not lead to loss of the label from basal cells indicating that they are the genuine stem cells in the trachea [24]. By contrast, lineage labeling of tracheal CC10+ (Club) cells showed that while labeled tracheal Club cells do proliferate and differentiate into ciliated cells they are gradually replaced over time by unlabeled cells, presumably the progeny of basal cells [25]. These experiments also indicated that while the differentiative potential of Club cells in basal cell-containing airways was more restricted than that of basal cells during homeostasis or after limited injury, they seemed to be able to generate basal cells after severe epithelial injury (albeit with very low efficiency) [25]. This ability of Club cells to “de-differentiate” into basal cells with a greater developmental potential was recently extensively studied by combining lineage tracing of Club cells with cellular ablation of basal cells (Fig. 6.1) [26]. Hegab et al. used the syngeneic heterotopic transplantation of mouse trachea as a model for severe hypoxic/ischemic injury for airway epithelium [27]. Soon after the injury, most cells of the tracheal surface epithelium underwent apoptosis and sloughed, with only a few surviving cells that were mostly K5+/K14− basal cells. Over the following days, these surviving basal cells, proliferated, acquired K14 expression, and started to differentiate gradually into secretory cells followed later by ciliated cells. BrdU labeling confirmed that most of the proliferating cells through the early repair phase were K5+ basal cells while in the later repair and remodeling period; some secretory cells were also BrdU labeled (Fig. 6.1) [27].
Collectively, it seems that during homeostasis and after different forms of injury, many basal cells throughout the proximal airways possess the ability to self-renew and differentiate towards the secretory and ciliated lineages. Club cells, in the presence of basal cells, have limited proliferative capacity but if basal cells were extensively damaged, they may contribute to the repair process by enhanced proliferation and possibly (de-) differentiation.
One interesting finding from basal cell lineage tracing is that basal cells responded to injury induced by SO2 inhalation by differentiating more towards a ciliated than Club cell fate, while during homeostasis the reverse was true [24]. This suggests that the fate of the basal cell progeny is dependent on interactions with its surrounding environment, namely, the niche. However, it may also indicate the presence of two or more subtypes of basal cells: one that is active during homeostasis and tends to differentiate more into Club cells, while the other subtype is activated in response to injury, which in the case of SO2 inhalation injury produces more ciliated cells. It is also possible that a different subtype of basal cell could respond to different types of injury. When Ghosh et al. lineage traced basal cells after inducing a selective injury to Club cells by naphthalene administration, they detected four different types of clones at 40 days post-injury. 50 % of these clones contained only basal cells, 25 % contained basal and secretory cells, and 19 % of clones had basal, secretory, and ciliated cells, while only 6 % contained basal and ciliated cell clones [16].
Indeed, there is accumulating evidence that there is a subset of basal cells, which has more stem cell characteristics than the rest of the basal cells. When basal cells with higher expression of K5 were sorted from a K5-GFP transgenic mouse, they produced more colonies that were also larger in size compared to basal cells with lower K5 expression [28]. Recently, sorting basal cells based on their aldehyde dehydrogenase activity (a commonly used marker of normal tissue and cancer stem cells [29]), enriched for a subset of basal cells that possessed more efficient two-dimensional (2D) [30] and three-dimensional (3D) colony/sphere formation ability (Fig. 6.1) [19, 31]. In the 3D colony/sphere formation assay, unfractionated basal cell spheres consistently formed spheres with a wide range of sizes [17, 24]. Further analysis of these spheres showed that they also had variable wall thicknesses and ratios of basal to differentiated cells (Hegab AE unpublished data).
Collectively, these data suggest the presence of different types of basal cells that have different responses to injury and possess different proliferative and differentiation abilities. Further studies are warranted to detect markers that can identify these different types in order to perform individualized characterization of their behavior both in vivo and in vitro.
Signaling Pathways Governing Basal Cell Proliferation and Differentiation
It is only recently that we have started to understand the factors regulating basal cell self-renewal versus differentiation during homeostasis and in response to injury. All basal cells in different epithelial tissues are typically marked by the transcription factor p63 (TP63, Trp63). P63 and basal cells seem to be essential for proper function of many organs. For example, loss of p63 from mammary gland basal cells leads to dramatic defects in luminal cell proliferation and differentiation, resulting in lactation failure [32], and loss of p63 in basal cells in the prostate results in disturbed ductal integrity and abnormal differentiation of luminal cells [33]. On the other hand, the trachea of P63 null mice contains no basal cells but shows a well-arranged columnar ciliated epithelium [34].
It is now well established that many stem cells can divide symmetrically, especially during organ development or in response to injury, when cell number expansion becomes a priority to establish an epithelial barrier, and this is also true of airway basal stem cells [35]. Asymmetric cell division (where daughter cells resulting from a cell division adopt divergent fates) is considered as a defining characteristic of stem cell differentiation and is associated with a significant drop in symmetric cell division [35]. Notch has been found to play two temporally distinct roles in airway epithelial repair—firstly, in self-renewal of basal cells and secondly in guiding the differentiation of basal cells towards ciliated or secretory lineages. Tsao et al. used a mouse model with conditional disruption of epithelial (but not mesenchymal) Notch. Inhibition of Notch in the developing lung of this model resulted in intrapulmonary airways that were entirely lined by ciliated cells but did not show any effect on the numbers of tracheal basal cells [36].
Notch has also been found to be important in adult airway epithelium homeostasis and during repair after injury. Using an in vivo reporter allele and genetic and pharmacologic gain- and loss-of-function experiments to demonstrate the role of Notch in cell fate determination of the proximal airway epithelium, Rock et al. found that Notch signaling drove basal cell differentiation to the secretory lineage, while the absence of Notch shifted the balance towards producing more ciliated cells [37]. They also speculated that Notch signaling is not required for self-renewal of basal cells [37]. However, further in-depth studies revealed that different combinations of Notch receptor activation and ligand binding resulted in completely different airway epithelial differentiation profiles [38]. It seems that components of the Notch pathway play complicated and tightly regulated roles in directing basal cell destiny. Paul et al. examined Notch pathway components both in vitro and in vivo to determine their role in controlling basal cell proliferation in response to injury [39]. Wild type mice received intratracheal polidocanol in order to slough off the airway surface epithelium and then the repairing airway epithelium was examined for Notch pathway components. Several components were upregulated during the repair process and were weakly expressed under steady-state conditions, suggesting that some Notch pathway components are active during basal cells proliferation.
Paul et al. also showed that dynamic changes in the level of reactive oxygen species (ROS) directly influence the ability of basal cells to self-renew and proliferate and that this is a tightly regulated process. This intriguing finding also suggests that exogenous ROS from air pollutants and cigarette smoke may influence airway epithelial cell proliferation and differentiation by altering the balance between the self-renewal and differentiation of basal stem cells. Thus, homeostasis is achieved by a regulatory loop in which the rise in ROS levels activates NRF2, the master transcription factor regulating the antioxidant response and NRF2, in its turn, has a direct regulating role on the Notch-mediated role in basal cell proliferation [39].
The epidermal growth factor receptor (EGFR) pathway in the lung epithelium regulates several key cellular processes including self-renewal, proliferation, repair, and differentiation. Aberrant EGFR signaling can cause basal cell hyperplasia, goblet cell hyperplasia, and induction of mucus production and/or promote fibrosis and collagen deposition [40]. An activating mutation in EGFR is associated with non-small cell lung cancer (NSCLC) and drugs that specifically target the EGFR pathway are now being used to treat NSCLC [41]. EGFR was found to have a role in controlling basal cell proliferation in homeostasis and during repair [42]. The EGFR inhibitor, AG1478, decreased basal cell proliferation and mucin production in experimental models [42, 43]. The Wnt-β-catenin pathway has also been shown to play a role in basal cell proliferation and differentiation both in vivo and in vitro [44–46].
One additional aspect of basal cell regulation was delineated with the identification of Myb as a key transcription factor that is expressed in post-mitotic epithelial cells that are destined to become multi-ciliated cells. Myb was shown to act downstream of Notch and upstream of Foxj1 and thus to be an essential regulator of basal cell differentiation towards ciliated lineages [47]. Another study demonstrated that Myb not only marked intermediate cells of the airway epithelium but was also critical in controlling their differentiation to both the ciliated and secretory lineages. Inhibiting Myb in airway epithelium in vitro, and Myb gene deletion in vivo, resulted in p63-negative cells which could not proceed to differentiate into ciliated or secretory cell lineages [48].
The Stem Cell Niche of the Airway Epithelium
It is well established that transcriptional regulation of a stem cell’s decision to proliferate or differentiate towards a specific lineage is markedly affected by mutual signaling with various components in the stem cell niche [49]. Tadokoro et al. have recently described a novel interaction between IL-6, a niche component, and Stat3, a transcription factor. They showed that IL-6 originating from cells in the nearby stroma activate basal cell Stat3-dependent signaling resulting in a shift of basal cell differentiation towards a ciliated cell fate. This regulation was found to be both at the level of ciliogenesis-related genes and transcription factors like the Multicilin gene and FoxJ1, as well as by inhibition of the Notch pathway [50].
Culturing human bronchial and mouse tracheal epithelial cells (MTEC) at an air–liquid interface (ALI) has been the standard assay for studying airway epithelium and its niche in vitro for many years [51, 52]. In this assay, a mixture of airway epithelial cells is seeded on a collagen-coated membrane in a transwell. Cells are submerged in medium and allowed to form “cobblestones” for several days before the medium is removed from the upper chamber to generate an ALI. A pseudostratified epithelium containing basal, Club, ciliated, and goblet cells is usually apparent starting after 5 days at the ALI. Various experiments, treatments, and interventions can be applied to the ALI and the effect on cells can be examined at varying time points.
More recently, the sphere/colony formation assay in a 3D culture system has emerged as a useful method to study and characterize various stem cell properties, such as proliferation, differentiation, and niche interactions [53, 54]. In this assay, the size and number of spheres reflects the proliferation capacity of stem and progenitor cells, while the ability to serially propagate spheres with subsequent passages is considered a measure of self-renewal potential of the basal cells. The sphere assay has several advantages for studying stem cells over the ALI culture system. It allows for direct observation of the proliferation and self-renewal potential of basal cells with various treatments or genetic alterations. Mixing of different types of cells or differently labeled cells, allows for identification of the spatial relationship between putative stem cells and putative niche cells and/or their differentiated progeny. There are an increasing number of studies exploiting this sphere/colony formation assay to identify various properties of airway basal cells and niche interactions [17, 19, 24, 31, 39]. However, as with any in vitro assay, the interpretation of findings about the behavior of basal cells from the sphere/colony formation assay needs to be evaluated carefully and further validated in vivo.
Intermediate Cells
Using specific markers, cell types populating the airway pseudostratified epithelium were identified as basal (30 % of cells), ciliated (30 %), secretory (goblet, serous and/or Club cells) (30 %), and rare neuroendocrine cells (1–2 %) [1, 3]. However, about 8–10 % of cells do not stain for any of these known markers and are known as “indeterminate” cells or “intermediate” cells which are thought to be in a state of transition/differentiation [1, 55]. They were also thought of as early short-lived progenitor cells because they were observed to arise from basal cells that survived SO2 injury before eventually differentiating into ciliated or secretory cell lineages. During this transition, they lost their basal cell markers and had not yet acquired ciliated or Club cell markers [37]. A disturbance in basal cell proliferation and differentiation programs, as might be seen in smokers and patients with COPD, characterized by basal cell hyperplasia or metaplasia is associated with an increase in incidence of both basal and intermediate cells [48]. Likewise, there is an increased incidence of intermediate cells and goblet cells in goblet cell hyperplasia [48]. The recent identification of the transcription factor, Myb, as a marker of these intermediate or early progenitor cells in both mouse and human (Fig. 6.1) [48] will likely pave the way for their further characterization by lineage tracing and exploration of their role during homeostasis and potential contribution to diseases of the lungs.
The Submucosal Glands
SMGs are complex branching structures located in the submucosal tissue of the human trachea and bronchi, but only in the most upper part of the trachea in mice. The SMG ducts open into the epithelium lining the surface of the airways. The duct branches into several collecting ducts which collect secretions from multiple mucus and serous tubules that form the glandular acini. Serous and mucus tubules are lined with polyhedral serous and mucus cells. The SMG tubules are surrounded by elongated MEC which express K5 and K14 in addition to α-smooth muscle actin. The SMG ducts are lined with a thin layer of stratified/pseudostratified epithelium. In the proximal part of the ducts, the luminal cells are ciliated. Both ciliated and basal cells of the SMG ducts seem to be in continuity with the ciliated and basal cells on the surface epithelium but basal cells of the SMG ducts differ from surface epithelial basal cells in that they are more flattened and express K14 [17, 18, 27]. Serous cells of the SMGs secret electrolyte-rich watery secretions, lysozyme, neutral mucins, and immunoglobulins. Mucus cells secret acidic mucins. The rate and nature of secretions from the SMGs is regulated by the cholinergic and non-cholinergic nervous systems and is affected by interactions with inflammatory cells, chemokines and allergens [56, 57]. Hypertrophic SMGs with enhanced secretion volume and a change in secretion constituents are important components of several lung diseases such as chronic bronchitis [58], cystic fibrosis [59], and asthma [60].
Stem Cells of the SMG
Much of the research on SMG stem cells has been conducted using animal models. It is therefore important to consider the structural and cellular differences between rodents and human airways and SMG [61], also highlighted in the introduction, when extrapolating these studies to humans. SMGs develop from the so-called primordial glandular placodes (PGPs), which are clusters of basally positioned epithelial progenitor cells within the developing airway epithelium. These PGPs start to invade into the submucosa and branch in response to signaling cues from the surrounding mesenchyme, eventually forming the branched networks of serous and mucus tubules of the SMGs [61].
Interestingly, when labeled human proximal airway epithelium was used to reconstitute a denuded tracheal xenograft, epithelial cells not only reconstituted the denuded tracheal surface but also invaded the submucosa and formed labeled SMGs, though infrequently [23]. Recently, the gradual appearance of age-related, gland-like structures (ARGLS) has also been described [62]. Interestingly, these ARGLS also seemed to arise by budding from the surface epithelium, similar to what is seen during SMG development and in the xenograft model (Fig. 6.1).
Collectively, it seems that some cells within the proximal airway surface epithelium, presumably some of the basal cells, are responsible for SMG embryonic development and they seem to preserve the potential to “bud” into the submucosa in a transplantation model and with aging.
SMG Duct Cells Are a SMG Stem Cell
Being in a relatively protected environment compared to the airway surface epithelium, SMG cells are expected to cycle even more slowly than the surface epithelium. Ciliated cells in the ducts of the SMG have a turnover rate of more than 18 months, much longer than the 6 months suggested for tracheal ciliated cells [10]. As mentioned above, LRCs were identified in SMG ducts after repeated injury to the surface epithelium [7]. In the same study, the ability of mouse SMG cells to contribute to the tracheal epithelial lining was suggested because when an enzyme-denuded trachea was transplanted subcutaneously in a nude mouse and examined after 28 days, the submucosal region showed cystic spaces lined by a “bronchiolized” ciliated epithelium [7]. These results suggest the potential participation of SMG duct stem cells in repairing injured surface epithelium. However, there is currently no injury model or cell ablation technique available that will specifically injure the SMG to help identify the stem cells maintaining and/or repairing the glands themselves.
Hegab et al. performed several studies to characterize the stem cells in the SMG ducts. In one study, they examined the chronological persistence or reappearance of epithelial cells in a tracheal graft after severe acute hypoxic/ischemic injury [27]. At day 1 after injury, all cells in the SMG bed were apoptotic and only a few K5+/K14+ cells in the gland ducts survived. These surviving duct cells were presumed to be the cells responsible for the ensuing repair [27]. Over the following days, these cells proliferated and gradually differentiated into secretory cells and MEC that invaded deeply into the submucosa eventually forming tubules that appeared to be functional. BrdU labeling confirmed that most proliferating cells during the early repair phase were K5+ duct cells while MECs were also seen to be proliferating during the later stages of repair and remodeling (Fig. 6.1) [27]. This study also confirmed the previous findings of Borthwick et al., [7] by demonstrating the ability of SMG cells to reconstitute the tracheal epithelium; enzymatically denuded prior to transplantation. Hegab et al. showed that SMGs could regenerate the denuded surface epithelium but this process was much slower than when surviving basal cells repaired the surface epithelium (Fig. 6.1) [27]. In another study, an alternative strategy was used to enrich SMG cells based on the finding that SMG duct cells, but not tubular cells, express Trop2 [17]. A 2-step enzyme digestion protocol was used to firstly detach the surface epithelium, and then digest SMG and other stromal cells in a second step prior to FACS sorting SMG duct cells based on their differential expression of Trop2 [17]. In human bronchi, a similar 2-step enzyme digestion protocol was used to separate surface epithelium from SMG cells and then sort basal and SMG duct cells on the basis of their differential expression of CD44 and CD166, respectively [19]. In the mouse, the self-renewal and differentiation potential of sorted SMG duct cells was demonstrated using both in vivo ectopic transplantation and in vitro colony/sphere formation assays, as well as lineage tracing of cells expressing K14 during repair after transplantation.
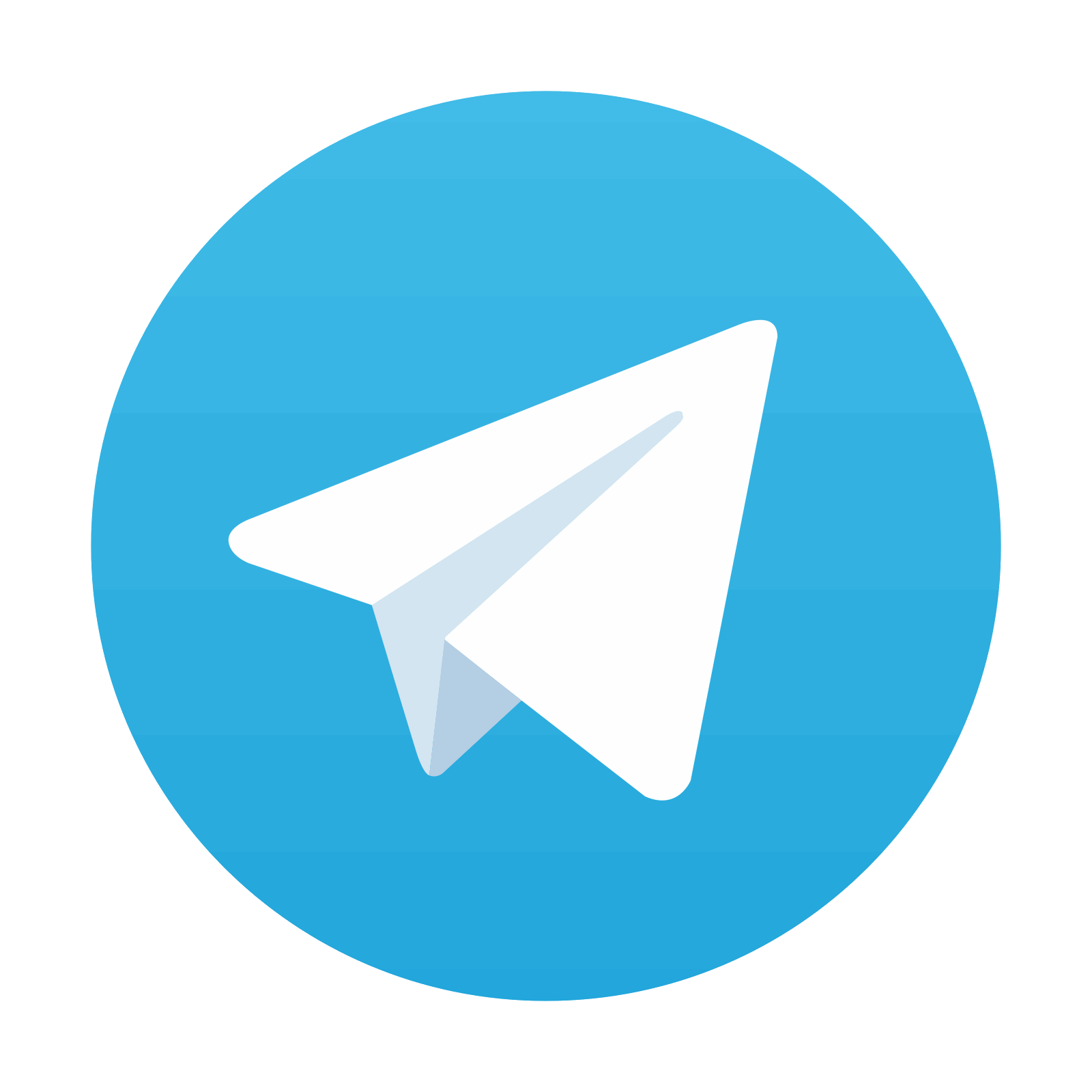
Stay updated, free articles. Join our Telegram channel

Full access? Get Clinical Tree
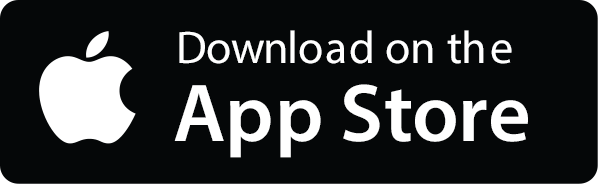
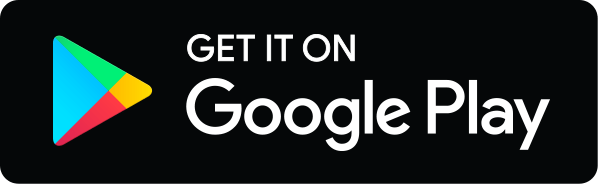