CHAPTER 83 Robotic Cardiac Surgery and Novel Visualization Systems
Traditionally, cardiac surgery has been performed through a median sternotomy, which provides generous operative exposure and allows ample access to all structures in and around the heart. This has allowed complex cardiovascular procedures to be performed safely with the use of cardiopulmonary bypass. Only after closed-chest cardiopulmonary bypass and cardioplegic arrest methods were developed1,2 did reductions in incision size become possible. These advances, in combination with advances in intracardiac visualization, instrumentation, and robotic telemanipulation, have hastened a shift toward minimally invasive robotic cardiac surgery. Already, improvements in endoscopic technology and techniques in noncardiac surgical procedures have demonstrated safety and efficacy. Today, robotic cardiac surgery, particularly valve surgery, has become standard practice for some surgeons. Herein, we describe our experience with robotic mitral valve surgery. Furthermore, we highlight recent progress in robotic coronary artery bypass surgery. Finally, various technological advancements that might enhance future robotic systems are outlined as well.
ROBOTIC TELEMANIPULATION SYSTEMS
Six degrees of freedom are required to allow free orientation in space. Thus, standard endoscopic instruments with only four degrees of freedom reduce dexterity significantly. When working through a fixed entry point, such as a trocar, the operator must reverse hand motions (fulcrum effect). At the same time, instrument shaft shear, or drag, induces the need for higher manipulation forces, leading to hand muscle fatigue.3 Also, human motor skills deteriorate with visual-motor incompatibility, which is associated commonly with endoscopic surgery. Computer-enhanced instrumentation systems have been developed to overcome these and other limitations. These systems provide both telemanipulation and micromanipulation of tissues in small spaces. The surgeon operates from a console, immersed in a three-dimensional (3D) view of the operative field, and through a computer interface, the surgeon’s motions are reproduced in scaled proportion through “micro-wrist” instruments that are mounted on robotic arms that are inserted through the chest wall. These instruments emulate human x–y–z axis wrist activity through seven full degrees of freedom.
The da Vinci S (Fig. 83-1) is an updated version that incorporates 3D high-definition visioning that increases viewing resolution, providing improved clarity and detail of anatomic structures. A fourth arm is now fully integrated and all instrument arms are smaller, providing better patient access. The patient cart is motorized, allowing rapid setup at the patient bedside. Ergonomics have been improved and interfaces have been streamlined to provide the latest in robotic telemanipulation systems.
EVOLUTION OF ROBOTIC CARDIAC SURGERY
Initially, minimally invasive cardiac valve surgery was based on modifications of previously used incisions and performed under direct vision. Mini-sternotomies, parasternal incisions, and mini-thoracotomies were used in the first truly minimally invasive aortic valve operations.4–6
Since its introduction in 1996 at Stanford University,1,2 Port-Access (Cardiovations, Inc., Ethicon, Somerville, NJ) technology combined a minimal surgical approach (avoidance of median sternotomy) with total cardiopulmonary bypass and an arrested heart. The system provides extrathoracic cardiopulmonary bypass with a specialized set of endovascular cannulas and catheters that allow antegrade or retrograde cardioplegic arrest, as well as ventricular decompression. Initial encouraging results confirmed the feasibility and safety of these techniques and further advanced the next level of minimal invasiveness.
Advances in video-optics initiated a wave of new endoscopic approaches in orthopedic, urologic, general, and gynecologic procedures. Cardiac surgery has lagged behind these specialties in utilizing the benefits of video assistance because fine coronary anastomoses and complex valve reconstructions are the centerpiece of contemporary adult cardiac surgery. Nevertheless, video assistance was used first for closed chest internal mammary artery harvests and congenital heart operations.7–9 Carpentier and colleagues performed the first video-assisted mitral valve repair via a mini-thoracotomy using ventricular fibrillation in February 1996.10 Three months later, our group at East Carolina University performed a mitral valve replacement using a microincision, videoscopic vision, percutaneous transthoracic aortic clamp, and retrograde cardioplegia.11,12
In 1997, Mohr first used the AESOP (Intuitive Surgical, Inc., Sunnyvale, CA) voice-activated camera robotic arm in minimally invasive videoscopic mitral valve surgery, and cardiac surgery entered the robotic age.13 This technology enabled the use of even smaller incisions with better valve and subvalvular visualization. In June of 1998, our group performed the first video-directed mitral operation in the United States, using the voice-controlled AESOP 3000 robotic arm and a Vista (Vista Cardiothoracic Systems, Inc., Westborough, MA) 3D camera.11,12 Visual accuracy was improved by voice manipulation of the camera by the operating surgeon. We now routinely use the robotic arm, endoscope, and a conventional two-dimensional (2D) monitor and have done more than 800 videoscopic mitral operations successfully with this method. The addition of 3D visualization, robotic camera control, and instrument tip articulation were the next essential steps toward a totally endoscopic mitral operation, in which wrist-like instruments and 3D vision could transpose surgical manipulations from outside the chest wall to deep within cardiac chambers.
Finally, by 1998, Carpentier performed the first mitral valve repair using an early prototype of the da Vinci articulated intracardiac “micro-wrist” robotic device.14 Two years later, our group performed the first complete repair of a mitral valve in North America using the da Vinci system.15 Using the articulated wrist instruments, a trapezoidal resection of a large P2 was performed, with the defect closed using multiple interrupted sutures, followed by implantation of a #28 Cosgrove annuloplasty band. Although a 4-cm incision is still used for assistant access, advancements in 3D video and robotic instrumentation have progressed to a point where totally endoscopic mitral procedures are feasible. In fact, Lange and associates in Munich were the first to perform a totally endoscopic mitral valve repair using only the 1-cm ports with da Vinci.16
In contrast, minimizing incisions and performing closed-chest (or nonsternotomy) coronary artery bypass surgery could be done only once the robotic telemanipulation system had been developed. Conventional thoracoscopic instruments could not be used to perform technically complex maneuvers such as a coronary anastomosis secondary to the lack of movement in all three axes. The “endo-wrist” was critical in achieving this goal. Early reports of success originated from several centers that were pioneering this effort.17–20 To date, only a few centers continue this work, and most recent contributions to the literature include case series and retrospective reviews. Robotic coronary surgery has gained less traction than mitral valve surgery. Future refinements in these devices will be needed to apply this new technology more widely.
CLINICAL APPLICATIONS/PATIENT SELECTION
In the development of any robotic cardiac surgical program, essential steps for each surgical operation (e.g., mitral repair, coronary artery bypass) should be learned and integrated in a stepwise fashion. This will allow the surgical team to build on its experience until the entire operation can be performed effortlessly. Furthermore, strict inclusion and exclusion criteria should be followed. In our initial experience, all patients had isolated mitral insufficiency without the valvular or coronary artery disease that might have required operative intervention (Box 83-1). Patients with a severely calcified mitral annulus were not candidates. Decalcification requires further development of instruments, as well as a reliable means of evacuating any calcium that falls into the left ventricle. Those with a previous right thoracotomy were excluded from the da Vinci procedures. Patients with mitral valve stenosis were excluded in the early trials of the U.S. Food and Drug Administration (FDA); however, patients treatable by commissurotomy would be suitable candidates for robotic repair. The improved visualization of the valve and subvalvular apparatus along with improved maneuverability of bladed microinstruments would facilitate performance of a commissurotomy.
SURGICAL TECHNIQUES
Cardiopulmonary bypass is established at 28° C using femoral arterial inflow cannula (19 or 21 French) and kinetic venous drainage through a femoral (22 or 23/25 Fr) and right internal jugular vein (17 Fr) cannula in the majority of cases. If the femoral artery is too small or atherosclerotic, either a Bio-Medicus (Medtronic, Minneapolis, MN) or Directflow (Cardiovations, Inc., Somerville, NJ) cannula can be placed through a second interspace port for antegrade aortic perfusion. Sometimes the right axillary artery can be cannulated as well. A 3- to 4-cm inframammary incision is used, and a subpectoral 4th intercostal space (ICS) mini-thoracotomy is developed to provide cardiac access. The pericardium is opened under direct vision, 2 cm anterior to the phrenic nerve (Fig. 83-2). Antegrade cardioplegia is given by an aortic needle or vent placed either under direct vision or videoscopically. To minimize intracardiac air entrainment, the thoracic cavity is flooded continuously with carbon dioxide at 1 to 2 L/min via a 14-gauge plastic catheter. A transthoracic aortic cross-clamp (Scanlan International, Inc., Minneapolis, MN) is positioned in the midaxillary line via a 4-mm incision in the 2nd ICS, and intermittent antegrade cold blood cardioplegia is used to maintain cardiac arrest and myocardial protection. Under video-assisted guidance, the posterior tine of the clamp is passed through the transverse sinus with care taken not to injure the right pulmonary artery, left atrial appendage, left main coronary, or aorta. After cardioplegic arrest, a 3- to 4-cm left atriotomy is made medially to the right superior pulmonary vein, and, after valve inspection, positions for da Vinci left, right, and fourth arm port incisions are determined. The right trocar is placed in the 4th ICS posterolateral to the incision and parallel to the right superior pulmonary vein. Occasionally, the 5th ICS provides a better angle for the right robotic arm. The left trocar is generally placed 6 cm cephalad and medial to the right trocar, ensuring internal clearance between arms to avoid both external and internal conflicts. Optimal robotic arm convergence avoids left atrial wall tearing during instrument manipulations. The fourth arm is positioned 1 to 2 cm lateral to the right internal mammary artery and one intercostal space cephalad to the working port incision (Fig. 83-3). A “high-magnification” camera is used with a 30-degree, 3D endoscope placed through the medial portion of the mini-thoracotomy. The remainder of the incision is used as a working port for the assistant. Needles are retrieved using a long magnetic device, and suture remnants are removed from the surgical field using vacuum assistance.
CLINICAL OUTCOMES
Mitral Surgery
In the year 2000, we completed and presented to the FDA our results from the first approved robotic mitral valve trial,21 which consisted of 10 patients. With the flexibility of the da Vinci we successfully performed quadrangular leaflet resections, leaflet sliding plasties, cord transfers, polytetrafluoroethylene chord replacements, reduction annuloplasties, and annuloplasty band insertions. The mean total arrest time was 150 minutes, with 52 minutes assigned to leaflet repairs. Of the total arrest time, a mean of 42 minutes was needed to place an average of 7.5 annuloplasty band sutures. Total operating room times averaged 4.8 hours. There were no device-related complications, and only one reexploration for bleeding from an atrial pacing wire. The average postoperative stay was 4 days (range, 3 to 7 days). At 3-month follow-up, echocardiography revealed nothing more that trace mitral regurgitation. All patients returned to normal activity by 1 month after surgery. On the basis of these results, an FDA extension of this safety and efficacy trial was granted at our institution.
In reviewing the Leipzig robotic mitral experience, Mohr and associates described 17 patients who underwent robotic mitral valve repair.3 His cohort was relatively young (58 ± 9 years) and predominantly female (n = 9). Fourteen of the 17 patients underwent a successful mitral valve repair with the da Vinci system. In three patients, conversion to conventional endoscopic instruments became necessary. The average cross-clamp time was 89 ± 18 minutes. After the repair, intraoperative TEE demonstrated no regurgitation in 13 patients and trace regurgitation in three. One patient had a grade 2 leak requiring immediate endoscopic valve replacement. Postoperative results were notable for one failure of a repair requiring emergent valve replacement on postoperative day 3, secondary to a disrupted annuloplasty ring.
In 2003, we reported results of our first 38 mitral repairs with the da Vinci system.22 Total robot time represents the exact time of robot deployment after valve exposure and continues until the end of annuloplasty band placement. This time decreased significantly from 1.9 hours in the first group of 19 patients to 1.5 hours in the second group. At the same time, leaflet repair times fell significantly from 1.0 hour to 0.6 hours. Also, total operating times decreased significantly from 5.1 hours in the first to 4.4 hours in the second group of patients. Both cross-clamp and bypass times decreased significantly with experience as well. For the entire group of 38 patients, the total length of stay was 3.8 days, with no difference between the two groups. Of all patients in the study, 84% demonstrated a grade 3 or greater reduction in mitral regurgitation at follow-up. In the entire series, there were no device-related complications or operative deaths. One valve was replaced at 19 days because of hemolysis secondary to a leak that was directed against a prosthetic cord.
We recently conducted a phase II clinical trial involving 112 patients at 10 institutions, which prompted FDA approval for robotic mitral repair.23 Valve pathology was primarily myxomatous (92%), with the majority of patients having type II leaflet prolapse. Leaflet repair times averaged 36.7 ± 0.2 minutes, with annuloplasty times of 39.6 ± 0.1 minutes. Total robot, aortic cross-clamp, and cardiopulmonary bypass times were 77.9 ± 0.3 minutes, 2.1 ± 0.1 hours, and 2.8 ± 0.1 hours, respectively. At 1-month echocardiographic follow-up, nine patients (8%) had grade 2 mitral regurgitation, and 6 (5.4%) of these had reoperations (five replacements and one repair). There were no deaths, strokes, or device-related complications. Although we regarded this initial number of reoperations as relatively high, the failures were distributed among four centers, all of which had enrolled at least 10 patients in the study.
In 2006, Murphy and colleagues published their retrospective review of 127 robotic mitral valve operations.24 Mean age was 54 ± 13 years and 58% of the patients were male. Five underwent conversion to median sternotomy and one to small thoracotomy, with 121 patients (95%) undergoing successful robotic surgery. Mitral valve replacement was necessary in seven (5.8%) patients. Mitral valve repair was completed in 114 patients, with the majority undergoing ring annuloplasty and posterior leaflet resection. Mean aortic occlusion time (with endoaortic balloon) was 102 ± 28 minutes, and mean cardiopulmonary bypass time was 131 ± 34 minutes. Although sutures were placed robotically, knots were tied extracorporeally by an assistant. Two patients (1.7%) required reoperation on the mitral valve. There was one (0.8%) hospital death and one late death. Two patients (1.7%) had a stroke. Mean follow-up was 13.7 ± 8.9 months and was 100% complete. Echocardiographic follow-up was available for 98 patients with a mean follow-up of 8.4 ± 8.1 months. Ninety-five of these studies demonstrated 0 to 1+ regurgitation, with 2+ in three patients.
One of the initial major concerns surrounding robotic mitral surgery has been the ability to perform complex mitral repair procedures. We recently reported on the largest cohort of patients (N = 66) who underwent either anterior or bileaflet da Vinci repair.25 Mean age was 52.6 ± 7.1 years and the majority (79%) underwent bileaflet repair. Repair techniques consisted of Gore-Tex neochord placement, chordal transfer, and annular sliding plasty. Mean cardiopulmonary bypass and cross-clamp times were 171 ± 52 and 132 ± 39 minutes, respectively. Complications included stroke (3%) and reexploration for bleeding (3%). Length of hospital stay was 5 ± 3 days. Thirty-day mortality was 3%, and six patients (9%) required valve reoperation. Mean follow-up in 60 patients was 795 ± 495 days, and echocardiographic mitral regurgitation was none or trace in 35 patients (58.3%), mild in 19 patients (31.6%), moderate in two patients (3.3%), and severe in four patients (6.7%). This report demonstrated overall feasibility and safety with robotic repair techniques applied to this complex group of patients with outcomes and degree of late mitral regurgitation similar to those seen in series using conventional techniques. Furthermore, a recent major technological addition, the incorporation of a robot-driven, left atrial retractor activated by the fourth robotic arm, enabled a more aggressive robotic approach for these patients. This observation has been substantiated by a recent report from Smith and coworkers.26
We have completed over 400 robotic mitral valve repairs with the da Vinci system, and the trends noted here continue to be observed. A retrospective review of our first 300 robotic mitral operations reflects the largest single-institutional experience in the world.27 Thirty-day mortality was 0.7%, and late mortality was 2%. Reoperations were required in 5.3%, the majority of which resulted from technical failures. Follow-up was obtained in 93%, and 94% had no mitral regurgitation. Five-year Kaplan-Meier survival and freedom from reoperation rates were 96.6% ± 1.5% and 93.8% ± 1.6%, respectively. These results compare favorably with traditional sternotomy-based mitral operations.
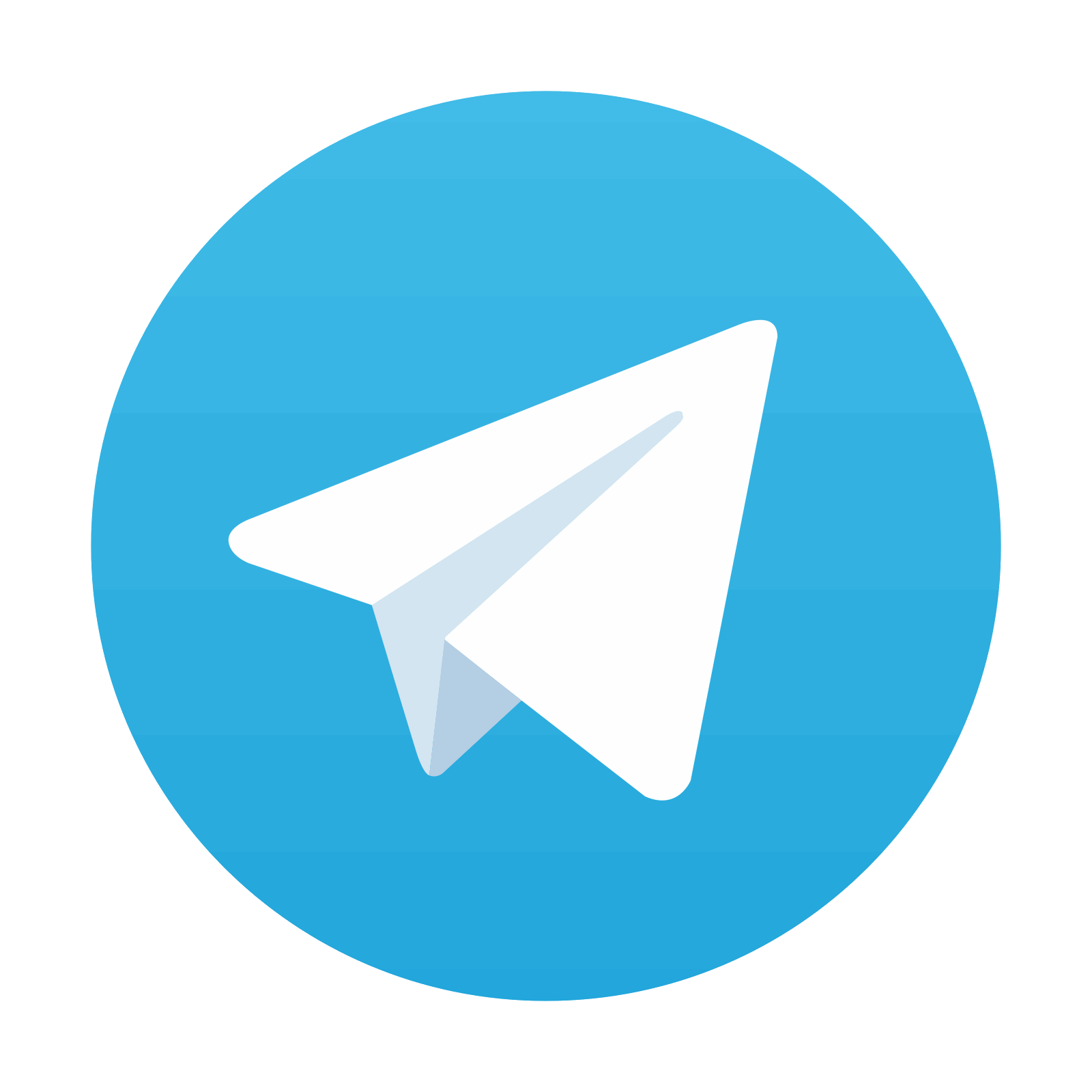
Stay updated, free articles. Join our Telegram channel

Full access? Get Clinical Tree
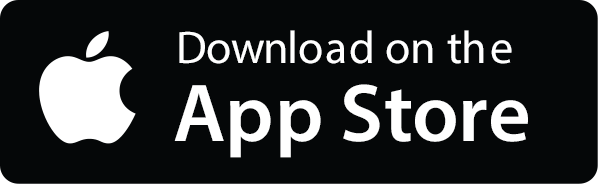
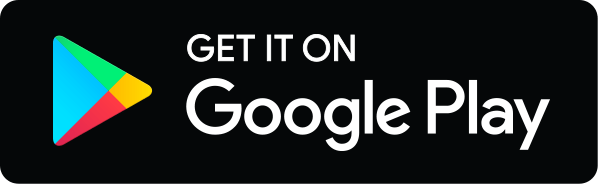