Robotic-Assisted Surgery in Thoracic Diseases
Kemp H. Kernstine
Natalie A. Ramirez
Simeon Cua
Minimally invasive surgery (MIS) is an integral part of current surgical practice and offers patients a number of potential advantages. In the hands of surgeons with proper training, the techniques are safe and produce long-term oncologic outcomes comparable to those of conventional surgery. However, MIS techniques in the thoracic cavity present special challenges, involving limited maneuverability, unnatural eye–hand coordination, and lack of true three-dimensional visibility. Because the vital organs present in close proximity, there is the potential for disastrous complications.
Through computer-assisted technology, robotic surgical systems provide visual clarity and unusual dexterity. Robotic systems are designed to simulate hand and finger movement close to the tissues rather than instrument movement with a fulcrum at the level of the ribs and chest wall. This motion may limit trauma to tissues and to the intercostal nerves. Other claims for robotic chest procedures include further reduction in pain, improved cosmesis, and promotion of an earlier return to the patient’s preoperative functional status, thus improving outcome and lowering the cost of care already realized with video-assisted techniques.
Proof of concept and early research began around 1990. In its current form the robot is embraced by specialties such as urology and gynecology, where operations are performed on organs that are fixed in position. In 1998, Himpens et al.39 reported that Cadiere had performed the first robotic cholecystectomy. By 2000, Chitwood and colleagues21 were using the robot for mitral valve repair. Today, interest is growing in anatomic lung resection, antireflux procedures, and treatment for dysmotility, and the resection of esophageal masses, including cancer. As with all tools, experience and further refinement in robotic instrumentation will lead to broader usage.
History and Current Technology
In December 1992, Computer Motion, Inc. (Santa Barbara, CA), completed a National Institutes of Health grant proposal to develop a robot with a voice-control interface. In September 1993, Computer Motion developed the first surgical robot, the AESOP 1000, which was approved by the U.S. Food and Drug Administration (FDA). Operated with foot controls, it was introduced clinically in October 1994. In 1996, Computer Motion completed development of a voice-controlled robot, the AESOP 2000, which was used in a MIS trial. Soon thereafter, the AESOP 3000, Computer Motion’s next voice controlled robot, was introduced. Computer Motion also developed the Zeus Surgical Control System and the Hermes Control Center, both of which use voice control.
Both Computer Motion and Intuitive Surgical, Inc. (Sunnyvale, CA), its competitor, developed machines to provide similar features: three-dimensional vision, high-definition visual clarity, and dampened instrument motion with multiple arcs of rotational freedom.
In July 2000, the FDA cleared Intuitive Surgical’s da Vinci Surgical System as an endoscopic instrument control system for use in laparoscopic surgical procedures. Its unique FDA labeling states that its purpose was to assist in “advanced surgical techniques such as cutting and suturing.” At that time, the ZEUS was cleared only to assist in grasping, holding, and moving structures out of the way but not for cutting or suturing. In March 2001, the FDA cleared da Vinci for use in general noncardiac thoracoscopic surgical procedures including the lungs, esophagus, and internal thoracic artery. In June 2001, the FDA cleared da Vinci for use during laparascopic removal of the prostate (radical prostatectomy).
Today, the da Vinci Surgical System is the only commercially available surgical robotic system designed to overcome the limitations observed in the current videoscopic techniques and equipment. It is comprised of three separate components: (1) a four-arm mounted platform from which extend the three-dimensional videoscope and two or three arms on which operative instruments can be interchanged; (2) the surgeon console; and (3) the integrative control cart, which provides communication between the surgeon console and the robotic arms (Fig. 34-1). In the operating room, the robotic equipment and console are almost always in proximity to the patient (Fig. 34-2A,B). The robotic system’s multiarticulated instruments provide 7 degrees of rotational freedom, referred to as the EndoWrist, mimicking the movement of the human wrist. The instruments that are attached to the arm enable retraction, grasping, cutting, ligation, and suturing. All fit through an 8-mm trocar and are mounted on 5-mm arms, most with the EndoWrist technology. Visual acuity and depth perception are
enhanced through a three-dimensional high-definition system with magnification.
enhanced through a three-dimensional high-definition system with magnification.
Technical Considerations
One of the major hurdles for the surgical team is getting used to the massive setup and space that the robot takes up. When in position and properly draped, there is little room for the scrub nurse and the assistant. However, both are vital to the operation, since instrumentation may need to be repositioned in order to accomplish the task from a variety of angles (Fig. 34-3).
For most robotic procedures, there is little guidance in the details of planning and preparation necessary for an efficient robotic thoracic procedure. Any technology used in the chest must accommodate the motion of the heart and lungs, but in abdominal and pelvic robotic procedures, it is not a consideration. The thoracic surgeon must have an understanding of videoscopic techniques and a three-dimensional understanding of the anatomy and pathology for port placement and patient positioning. Procedures involving the lower esophagus, periesophageal, and diaphragmatic structures are likely best approached transabdominally. Ports and retractors for laparoscopic procedures on the esophagus are described in Chapters 135, 140, and 155. The robotic arm length is capable of reaching as high as the mid-mediastinum.
Transthoracic robotics necessitates different planning than the thoracoscopic approach. The rigidity of the chest wall combined with the movements of the heart, lungs, and mediastinum present technical challenges. Important variables to be considered in determining the patient’s body position and port placement include location of the pathology, patient morphometry, and pulmonary and cardiac function. The preoperative computed tomography (CT) is critical for planning. The port locations for the robotic arms should be chosen to enable maximum dexterity of the instruments and avoid instrument interference in or outside of the chest. If the ports are positioned too close to each other or the videoport arm, significant function may be lost. For children and small adults, the ports should be the furthest distance possible from the target structure to preserve robotic functional freedom. Considering the current robotic equipment, not all patients will be capable of undergoing robotic surgery.
Patient Positioning
Patient positioning in robotic chest surgery, as in other minimally invasive approaches, is a critical factor in providing sufficient exposure and maintaining the pace of the operation. The weight of the mediastinal and lung structures can be used as an advantage to provide exposure of normally concealed areas and create room for dissection and countertension to planes of dissection. The supine position with a slight tilt in the operating room table appears best for anterior mediastinal structures. For example, a mass in the right aspect of the middle to upper mediastinum might be most easily approached with the patient in the supine position with the right side of the table slightly raised or tilted, 15 to 30 degrees, and in slightly reverse Trendelenburg. Anatomic lung resections and hilar dissections may be better approached by a near lateral decubitus position with 30-degrees anterior tilt for lower lobe or posterior hilar resections and 30-degrees posterior for upper lung and anterior hilar resections. For more posterior mediastinal structures, such as the esophagus, the nearly prone position provides better exposure of masses and most of the esophagus. Once the patient is properly positioned and the robot is in place, further changes in the patient’s position are prohibited and likely to result in injury.
To provide further exposure, CO2 insufflation may be used to distend the mediastinum, creating more operative space for dissection and compressing the ipsilateral lung and the diaphragm away from the surgical target.
Port Placement
Like patient positioning, proper port placement is critical to ensure efficiency. Once the patient is in position and prior to docking the robot, the surgical target can be drawn on the patient’s chest. This might not represent the pathology to be addressed. In the case of a lobectomy, the target is the hilum, rather than the location of a mass in the lung. For patients with small masses, the range of robotic view and movement is ideally suited for the currently available robot machine—i.e. small view and limited range of motion. In the case of a large mass or a procedure such as an “extended” thymectomy or an esophagectomy, the range is broad and may present challenges.
After the target is drawn on the patient’s chest, the videoport location is selected opposite and at a sufficient distance away to allow adequate instrument insertion and maximization of instrument function. For small chests, the site may be toward the patient’s back.
Next, a triangle is drawn on the patient’s chest creating a “triangle of visibility”: with the videoport site at the apex of the triangle and the surgical target at the base. The location of robotic arms two and three should be outside of the triangle to minimize instrument collision. Additionally, the robotic arm ports should be placed 8 to 10 cm away from the videoport and 12 to 15 cm from the surgical target. The length of the robotic instruments varies and maneuvers to improve their reach may be required later. For example, graspers, needle holders, and cautery units are longer than the ultrasonic unit and provide greater reach. As necessary, additional ports may be placed for a fourth robotic arm or accessory instrument port to be used by the surgical assistant for retraction or additional dissection. The basic setup for pulmonary resection and esophageal surgery has been published (Figs. 34-4 and 34-5).
Techniques to improve the reach of the robotic arms include (1) inserting the trocars further into the chest so that they are
flush with the chest wall, (2) moving the trocar a rib space above or below through the same skin incision, (3) adding positive end-expiratory pressure to the opposing lung, and (4) removing some of the CO2 used during the procedure. To gain further advantage, the 0-degree scope’s or a 30-degree scope’s upward or downward gaze is useful in attempting to view remote areas around unretractable structures. For retraction, the partially open fan retractor (U.S. Surgical, Norwalk, CT) appears to be adequate in most cases except for those involving severe lung disease, where it may traumatize the lung. The paddle retractor (U.S. Surgical) is less traumatic in these cases but fairly bulky where the cloth on the paddle can bunch and obstruct the view. Given its fairly soft and atraumatic construct, the paddle is ideal for retracting and in some situations rotating the heart and great vessels to provide exposure.
flush with the chest wall, (2) moving the trocar a rib space above or below through the same skin incision, (3) adding positive end-expiratory pressure to the opposing lung, and (4) removing some of the CO2 used during the procedure. To gain further advantage, the 0-degree scope’s or a 30-degree scope’s upward or downward gaze is useful in attempting to view remote areas around unretractable structures. For retraction, the partially open fan retractor (U.S. Surgical, Norwalk, CT) appears to be adequate in most cases except for those involving severe lung disease, where it may traumatize the lung. The paddle retractor (U.S. Surgical) is less traumatic in these cases but fairly bulky where the cloth on the paddle can bunch and obstruct the view. Given its fairly soft and atraumatic construct, the paddle is ideal for retracting and in some situations rotating the heart and great vessels to provide exposure.
Robotics in Specific Procedures
Esophagus
The first robotic thoracic procedures performed were gastric fundoplications and esophageal myotomies. Success with these procedures has led to a steady rise in the utilization of robotics in this area over the subsequent decade.
Fundoplication
Published reports of robotic fundoplications demonstrate that these procedures have been performed safely, with operating times in the range of 2 to 3 hours (Table 34-1). There have been
nearly 500 reported cases in the literature with a median length of stay of 3 to 4 days, a median morbidity of 4%, and no reported mortality (Table 34-1). Critics of robotic fundoplication have raised issues about the awkward robotic docking and trocar placement, unadapted trocar length for use in obese patients, and a narrow optical field as contributing factors to a relatively inefficient surgical procedure.75 In the six reported trials comparing MIS with robotic techniques, the initial experience with robotic fundoplication required a 30% longer operating time, had a 10% rate of esophageal perforation, and a morbidity that was usually less than 20% (Table 34-2). With greater experience, the operating room times appear similar, with a nearly equivalent hospital stay, morbidity, no reported mortality, and no perforations at the time of surgery. Long-term comparisons are not available.
nearly 500 reported cases in the literature with a median length of stay of 3 to 4 days, a median morbidity of 4%, and no reported mortality (Table 34-1). Critics of robotic fundoplication have raised issues about the awkward robotic docking and trocar placement, unadapted trocar length for use in obese patients, and a narrow optical field as contributing factors to a relatively inefficient surgical procedure.75 In the six reported trials comparing MIS with robotic techniques, the initial experience with robotic fundoplication required a 30% longer operating time, had a 10% rate of esophageal perforation, and a morbidity that was usually less than 20% (Table 34-2). With greater experience, the operating room times appear similar, with a nearly equivalent hospital stay, morbidity, no reported mortality, and no perforations at the time of surgery. Long-term comparisons are not available.
Table 34-1 Robotic Fundoplication Procedures | ||||||||||||||||||||||||||||||||||||||||||||||||||||||||||||||||||||||||||||||||||||||||||||||||||||||||||||||||||||||||||||||||||||||||||||||||||||||||||||||||||||||||||||||||||||||||||||||||||||||||||||||||||||||||||||||||||||||||||||||||
---|---|---|---|---|---|---|---|---|---|---|---|---|---|---|---|---|---|---|---|---|---|---|---|---|---|---|---|---|---|---|---|---|---|---|---|---|---|---|---|---|---|---|---|---|---|---|---|---|---|---|---|---|---|---|---|---|---|---|---|---|---|---|---|---|---|---|---|---|---|---|---|---|---|---|---|---|---|---|---|---|---|---|---|---|---|---|---|---|---|---|---|---|---|---|---|---|---|---|---|---|---|---|---|---|---|---|---|---|---|---|---|---|---|---|---|---|---|---|---|---|---|---|---|---|---|---|---|---|---|---|---|---|---|---|---|---|---|---|---|---|---|---|---|---|---|---|---|---|---|---|---|---|---|---|---|---|---|---|---|---|---|---|---|---|---|---|---|---|---|---|---|---|---|---|---|---|---|---|---|---|---|---|---|---|---|---|---|---|---|---|---|---|---|---|---|---|---|---|---|---|---|---|---|---|---|---|---|---|---|---|---|---|---|---|---|---|---|---|---|---|---|---|---|---|---|---|---|---|---|---|---|---|---|---|---|---|---|---|---|---|
|
Table 34-2 Prospective Clinical Trials Comparing Robotic and Videoendoscopic Fundoplication Procedures | |||||||||||||||||||||||||||||||||||||||||||||||||||||||||||||||||||||||||||||||||||||||||||||||||||||||||||||||||||||||||||||||||||||||||||||||||
---|---|---|---|---|---|---|---|---|---|---|---|---|---|---|---|---|---|---|---|---|---|---|---|---|---|---|---|---|---|---|---|---|---|---|---|---|---|---|---|---|---|---|---|---|---|---|---|---|---|---|---|---|---|---|---|---|---|---|---|---|---|---|---|---|---|---|---|---|---|---|---|---|---|---|---|---|---|---|---|---|---|---|---|---|---|---|---|---|---|---|---|---|---|---|---|---|---|---|---|---|---|---|---|---|---|---|---|---|---|---|---|---|---|---|---|---|---|---|---|---|---|---|---|---|---|---|---|---|---|---|---|---|---|---|---|---|---|---|---|---|---|---|---|---|---|
|
Several comparisons exist for cost comparison. The direct medical costs for laparoscopic versus robotic fundoplication were similar in relation to the surgical procedure expense, pharmacy, and hospital stay.75 When the depreciation of the robotic machine, maintenance expenses, and instrumentation are considered, expense is quite different. In an institution where the robot is utilized for 500 other cases per year, the added cost for a robotic fundoplication was $2,356 per case. However, when the singular cost was considered for robotic fundoplication, it was over $32,725 per case. With robotics, Morino et al.72 found a 40% longer operating time and an additional $2,094 cost per case.
Four of the trials comparing laparoscopic and robotic fundoplication also included outcome analysis. On average, patients had similar DeMeester scores and normalization of the pH exposure up to 2 years postoperatively. Draaisma and colleagues26 at the University Medical Center of Utrecht, The Netherlands, randomized 50 patients to a laparoscopic versus robotic loose Nissen performed without a bougie. All of the patients had a crural repair, short gastrics divided, and a 3-cm intra-abdominal esophagus restored around which a 2.5- to 3.5-cm three-suture wrap was performed. The surgeons in the study reported that there were improvements in visualization and ergonomics. They studied their patients with endoscopy, pH monitoring, barium swallow, manometry and quality-of-life assessment. Over 90% of the patients were satisfied with the surgery and the same number felt that their GERD symptoms were relieved. Nineteen of the 25 in each group (76%) had preoperative esophagitis of various degrees, which was nearly eliminated in 16 (84%) in each group. Lower esophageal acid exposure normalized in all but three patients (12%) in the robotic group, who were asymptomatic and normalized with antisecretory medication. One patient in the robotic group and three patients in the laparoscopic group had sliding hiatal hernias documented by both endoscopy and barium swallow. Within 2 years, reoperations for failed Nissen were required in one robotic patient and two laparoscopic patients. In a separate publication, the group found that there was a $10,220 extra cost for a robotic procedure, which would increase by another $1,309 if upgrades were made to the system.27 The investigators concluded that the extra costs of robotics were not justified given the equivalent clinical results.
In children, similar results have been found in a retrospective review of robotic fundoplication, which has been considered feasible and safe. However, development of pediatric-sized robotic instrumentation was considered important for future adoption of robotic surgery in children and infants.36
Heller Myotomy
Its enhanced visibility and dexterity appear to demonstrate benefit of the robotic Heller myotomy (Fig. 34-6). There have been nearly 300 cases reported in 10 publications with an average conversion rate of <1%, a median of 2 hours operative time, and mucosal perforation in two patients (1%). Table 34-3 summarizes the robotic experience. There are no reported mortalities and the overall morbidity is less than 3%. Melvin et al. report the largest series to date (104 patients): all patients had a combined partial fundoplication.65 Over the course of the study from 2000 to 2004, there was a 30% reduction in operative time to a mean of 1.9 hours. There were no mucosal perforations, but one patient required conversion to an open laparotomy. Sixty-two percent of patients were discharged on the first postoperative day.
Symptoms improved in all patients, and over the year-long follow-up, no patient required a reoperation.
Symptoms improved in all patients, and over the year-long follow-up, no patient required a reoperation.
Three prospective trials compared the robotic and the laparoscopic Heller–Dor or Heller–Toupet myotomy (Table 34-4). The largest published trial was performed by Horgan and colleagues.41 This was a multi-institutional retrospective comparison of 120 patients and reported a lower mucosal perforation rate, lower blood loss, and shorter hospitalization for the patients who underwent robotic surgery compared with those who received laparoscopy. The overall operating time was similar between the two groups, with a median of 2 hours. Overall, the operating time for the Heller–Dor myotomy is approximately 2 to 4 hours in similar trials.35,41,44,46 Also, times are similar for robotic Toupet procedures.35,42,67,102
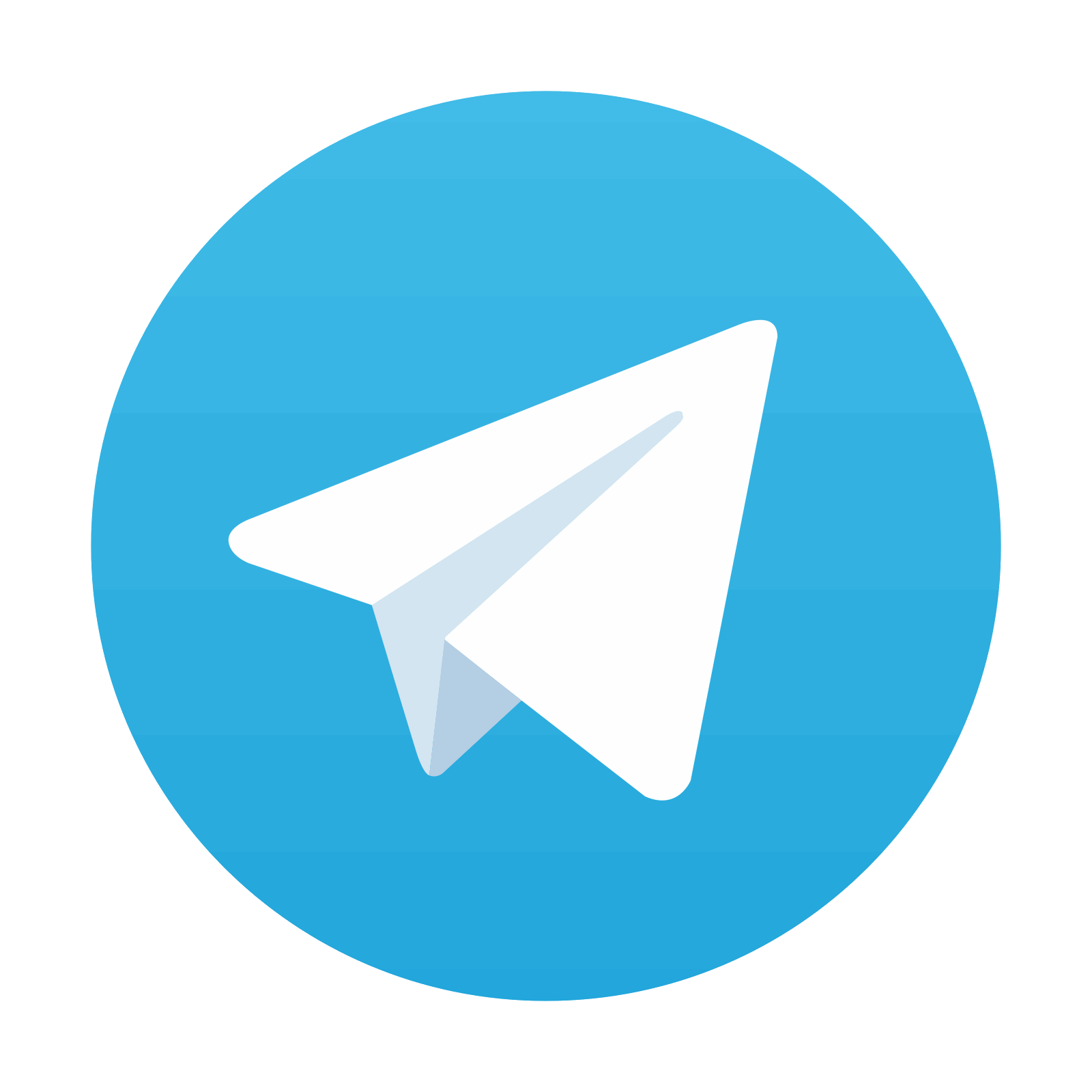
Stay updated, free articles. Join our Telegram channel

Full access? Get Clinical Tree
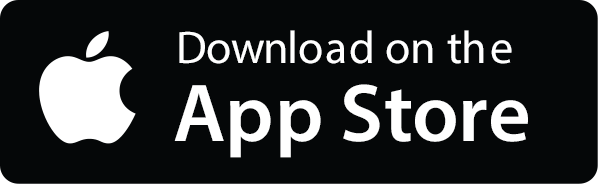
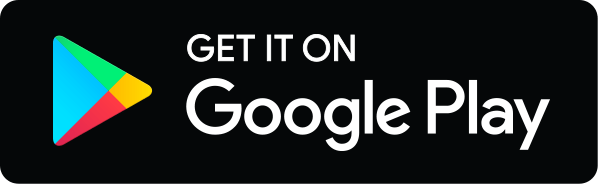
