The implanted prosthetic heart valve (PHV) size is vital for the evaluation of suspected PHV dysfunction and in case of reoperation or valve-in-valve transcatheter approaches. The labeled size is not always known, and discrepancies exist between manufacturers’ labeled sizes and true sizes. Reproducible methods for noninvasive PHV size assessment are lacking. We determined the reliability and agreement of PHV size measurements using multidetector-row computed tomography (MDCT) and provide reference values of MDCT measurements compared with manufacturer specifications. In vitro, 15 different PHV types in available sizes (total n = 63) were imaged. In vivo, available MDCT acquisitions of patients with PHVs were retrospectively gathered in 2 centers, and 230 patients with 249 PHVs were included. Inner valve area and area-derived diameter were measured in all PHVs. For mechanical PHVs, the inner diameter was also measured. Data were analyzed using the intraclass correlation coefficient and Bland-Altman plots and related to manufacturer specifications. Measurements could be obtained for all PHV types, except the Björk-Shiley (n = 7) because of severe valve-related artifacts hampering the image quality. Intrarater and interrater reliability was excellent for biological and mechanical PHVs (intraclass correlation coefficients ≥0.903). Agreement was good for all measurements with an overall maximal mean difference (95% confidence interval) of −2.61 mm 2 (−37.9 to 32.7), −0.1 mm (−1.1 to 1.0), and 0 mm (−0.4 to 0.3) for valve area, area-derived diameter, and inner diameter, respectively. MDCT reliably discriminated consecutive PHV sizes as labeled by the manufacturer because the absolute ranges for the measurements never overlapped. In conclusion, MDCT allows assessment of the implanted PHV size with excellent reliability and agreement and can discriminate between PHV sizes for contemporary prostheses. MDCT can be used to noninvasively identify the manufacturer-labeled PHV size.
For the analysis of prosthetic heart valve (PHV) function, the labeled prosthesis size may help to discriminate between valve stenosis and patient prosthesis mismatch. Both the size and type of the PHV are important for the decision to replace a dysfunctional PHV to prevent patient prosthesis mismatch and predict the potential gain of replacing the PHV. For transcatheter valve-in-valve procedures, knowledge of the PHV size is paramount for choosing the size of the valve to be implanted because direct measurement as used during conventional surgery cannot be performed. In addition, valve-in-valve replacement in small sizes of biological PHVs may lead to excessively high transprosthetic gradients. Sometimes, the labeled size may not be documented in the patients’ medical files, and obtaining medical records from other hospital centers can be difficult or even impossible. In addition, the manufacturer-labeled PHV size does not reflect the true prosthetic internal diameter, effective valve area, or the external PHV tissue diameter, and important differences may exist between valve types of the same labeled size. Noninvasive assessment of the valve size may improve the interpretation of echocardiographic results and optimize the patient-specific decision-making process. Conventional echocardiography is a readily available noninvasive technique but may provide unsatisfactory results for prosthetic size assessment because of its 2-dimensional nature and impaired image quality because of prosthetic components causing acoustic shadowing. In our daily practice, preoperative multidetector-row computed tomography (MDCT) is routinely performed to assess the cause or extension of PHV pathology and for the determination of re-entry strategy before redo surgery. Since MDCT offers 3-dimensional information, it might be a promising modality for concomitant noninvasive PHV size assessment. The purpose of this study was (1) to assess the reliability and agreement of PHV size measurements using MDCT acquisitions in vitro and in vivo and (2) to provide a reference overview of MDCT size measurements compared with manufacturer specifications.
Methods
In vitro, we evaluated 8 mechanical and 7 biological PHVs from various manufacturers and in different sizes (17 to 33 mm). A total of 63 PHVs were included. The complete list of studied PHVs is presented in Supplementary Table 1 . All valves remained in the original packaging and were imaged in nonpulsatile setting.
Images were acquired with either a 256- or 64-slice MDCT scanner (Philips Healthcare, Best, The Netherlands) using retrospective electrocardiographic (ECG) gating with an ECG simulator and the following parameters: slice collimation of 128 or 64 × 0.625, pitch of 0.2, gantry rotation time of 0.27 or 0.40 seconds, tube voltage of 120 kV and tube current-time product of 150 mAs. All raw data were reconstructed with filtered back projection. Because images were acquired in still valve position, only the 75% phase of the RR interval was used for analysis. Reconstructed image thickness was 0.9 mm with an increment of 0.45 mm.
For the in vivo study, all patients with biological and mechanical PHVs and available cardiac MDCT imaging performed from January 2007 to March 2014 were retrieved from the medical archives of the University Medical Center Utrecht and the Academic Medical Center Amsterdam, The Netherlands. Both retrospective ECG-gated and prospective ECG-triggered acquisitions routinely used for cardiac imaging and performed on a 64- or 256-slice MDCT scanner (Philips Healthcare) were included for analysis. Most images in this study were acquired at 120 kV (95%) and the remaining at 100 (4%) and 140 kV (1%). Our MDCT acquisitions are reconstructed using filtered back projection. For patients with multiple MDCT acquisitions, only 1 was included. Data on implanted PHV type and size were obtained for each patient from the medical records and served as the reference standard. Exclusion criteria were unknown valve type or size, annuloplasty rings, and stentless valves because these do not contain a radiopaque structure that can serve as a reference point. MDCT acquisitions of all included patients were collected and sent to a dedicated workstation for analysis. Informed consent was waived by the local medical ethical committee.
Images were analyzed by 2 observers independently using commercially available software (Extended Brilliance Workstation, version 4.5; Philips Healthcare). The observers were blinded for the nominal PHV size. For each PHV, images were reconstructed in 3 planes: 1 in plane with the PHV (short axis) and 2 perpendicular to the PHV, of which 1 plane was set perpendicular to the valve leaflets (long axis, Figure 1 ). All measurements were performed using a default window for bone, with a window level of 800 and window width of 2,000 Hounsfield units, to obtain standardized measurements and limit measurement errors due to blooming of the PHV components. The cardiac phase providing the best MDCT image quality of the PHV was used for analysis. Image magnification was conducted at the discretion of the reader.
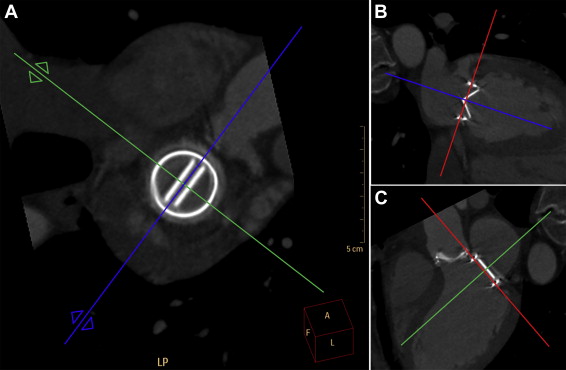
For all valves, the inner PHV area was measured on the transverse plane using a circular region of interest (ROI) tool available in the software. The circle ROI was drawn either within the narrowest inner part of the radiopaque valve ring or, if no ring was visible, within the 3 tops of the biological valve struts ( Figure 2 ). If a complete circular ROI was not possible because of (biological valve) ring deformation, the inner valve area was defined manually by drawing points connected into a spline curve ( Figure 2 ). For each PHV, the inner valve area (mm 2 ) and area-derived diameter (mm) were documented. In case of mechanical PHVs, the plane perpendicular to the valve leaflets was used additionally to measure the inner valve diameter using the straight-line tool from the inner edge of the PHV ring to the inner edge on the opposing side of the ring ( Figure 2 ). For in vivo acquisitions, the image quality was scored on a 3-point scale: (0) poor, (1) fair, and (2) good ( Figure 3 ).
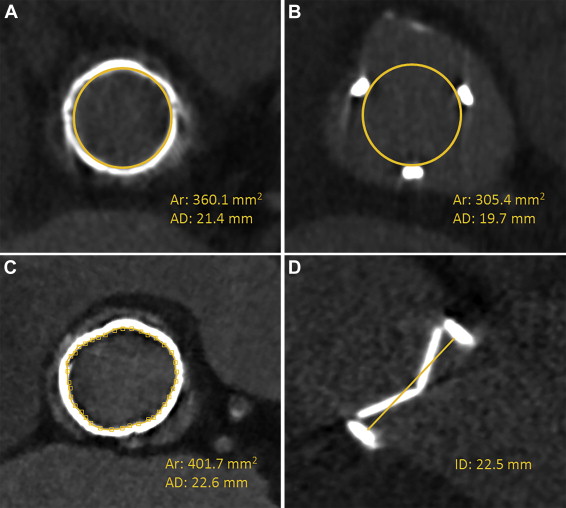
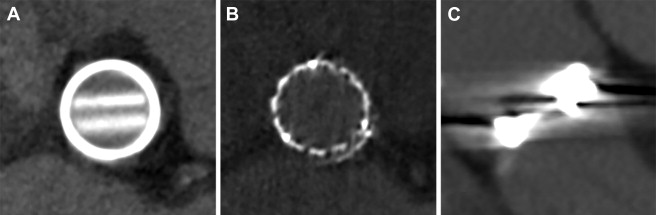
Two readers with experience of 2 and 6 years in cardiac MDCT independently measured all in vitro acquisitions (DS and PS, respectively). After 1 month, 1 reader (DS) measured all in vitro images again for the intrarater analysis. All measurements in the patient acquisitions were performed by 1 observer with 1-year experience (CD) and repeated in a random sample of 70% of patients after a period of at least 3 weeks. In addition, a second observer (DS) measured a random sample of 10% of the patient acquisitions for the interrater analysis.
Collected data were analyzed using IBM SPSS Statistics 20.0 for Windows (IBM Corp., Armonk, NY). Intrarater and interrater reliabilities were assessed by the 2-way random intraclass correlation coefficient (ICC) with absolute agreement. Intrarater and interrater agreements were evaluated using the Bland-Altman plots with 95% limits of agreement. The possibility to discriminate consecutive PHV sizes was studied in all PHV subgroups by the median and maximal absolute range.
Results
Both intrarater and interrater reliabilities for the in vitro measurements were excellent for valve area, area-derived diameter, and inner diameter (ICC ≥ 0.995). Agreement within and between raters was excellent as well ( Table 1 ). The greatest difference found for diameter measurements between readers was 0.8 mm. This is less than the minimal difference between 2 studied consecutive PHV sizes (1.5 mm) with differing internal diameters as provided by the manufacturers.
Mean Δ (SD) | 95% CI | ICC | ||
---|---|---|---|---|
Intrarater | Area | -0.7 (5.5) | -11.5 – 10.1 | 0.999 |
AD | 0 (0.2) | -0.4 – 0.4 | 0.999 | |
ID | 0 (0.1) | -0.2 – 0.2 | 1.000 | |
Interrater | Area | 6.3 (10.6) | -14.5 – 27.1 | 0.995 |
AD | 0.2 (0.3) | -0.4 −0.8 | 0.995 | |
ID | 0 (0.2) | -0.4 – 0.4 | 1.000 |
A total of 230 patients with available MDCT imaging were included comprising 249 PHVs: 166 mechanical and 83 biological valves. The position of the implanted PHV was 200 aortic, 43 mitral, 5 tricuspid, and 1 pulmonary. The image quality was scored as good in 164 (66%), fair in 52 (21%), and poor in 33 PHVs (13%). In the latter group, poor image quality due to valve-related artifacts precluded assessment of the inner valve diameter in 6 valves (all Björk-Shiley) and in 1 valve the area measurements as well could not be performed because of extensive valve-related artifacts (Björk-Shiley). In total, 48 PHVs (31 mitral) were assessed in systolic phase (10% to 40% of the RR interval) and 200 (184 aortic) in diastolic phase (70% to 0% of the RR interval). The vast majority was assessed in closed valve position.
Results of the intrarater and interrater reliabilities for the in vivo measurements are presented in Table 2 . An excellent correlation was found for both mechanical and biological valves (ICC ≥0.903). Good intrarater and interrater agreements were found in general and after stratification by main PHV type. All differences for diameter measurements within and between readers were less than the minimal difference between 2 consecutive sizes. The results on measurement agreement are presented in Table 2 and Figure 4 .
Mechanical | Biological | ||||||
---|---|---|---|---|---|---|---|
Mean Δ (SD) | 95% CI | ICC | Mean Δ (SD) | 95% CI | ICC | ||
Intrarater | Area | 2.4 (11.8) | -20.7 – 25.5 | 0.993 | 1.6 (12.1) | -22.1 – 25.3 | 0.988 |
AD | 0.1 (0.4) | -0.7 – 0.9 | 0.992 | 0 (0.3) | -0.6 – 0.6 | 0.988 | |
ID | 0.1 (0.3) | -0.5 – 0.7 | 0.994 | n/a | |||
Interrater | Area | -4.8 (12.7) | -29.7 – 20.1 | 0.991 | 0.1 (23.4) | -45.8 – 46.0 | 0.910 |
AD | -0.1 (0.3) | -0.7 – 0.5 | 0.993 | 0 (0.7) | -1.4 – 1.4 | 0.903 | |
ID | 0 (0.2) | -0.4 – 0.4 | 0.998 | n/a |
MDCT examples of the most common valves are presented in Figures 5 and 6 . The reference overview in Table 3 lists the median and range of the valve area and inner diameter measured in vitro and in patients for all studied mechanical valves obtained from the first measurements of observer 1 (CD). For biological valves, the median and range for the inner valve area and area-derived diameter are presented in Table 4 . When available, results are compared with the dimensions provided by the manufacturers. As shown, the maximal range for specific PHV size measurements does not overlap with the consecutive labeled PHV size; thus, PHV sizes can be discriminated. This is valid for consecutive labeled PHVs that truly differ in diameter of area as provided by the manufacturer.
Manufacturer | Size | N ∗ | Area † (mm 2 ) | ID (mm) | ||||
---|---|---|---|---|---|---|---|---|
MFR | In vitro | In vivo | MFR | In vitro | In vivo | |||
Carbomedics | 21 | 6 | 207 | 223.8 | 218.3 [212.4-235.8] | 16.7 | 16.8 | 16.9 [16.4-17.3] |
23 | 21 | 256 | 275.6 | 267.7 [261.1-276.6] | 18.5 | 18.8 | 18.6 [18.1-18.8] | |
25 | 18 | 316 | 346.0 | 325.9 [307.7-335.1] | 20.5 | 21 | 20.4 [19.6-20.8] | |
27 | 17 | 384 | 414.2 | 395.6 [364.1-410.4] | 22.5 | 23.0 | 22.5 [22.0-23.1] | |
29 | 5 | 444 | 468.7 | 454.3 [440.7-463.6] | 24.2 | 24.5 | 23.9 [23.5-24.4] | |
31 | 5 | 444 | 473.3 | 450.8 [439.5-455.3] | 24.2 | 24.4 | 23.8 [23.6-24.2] | |
33 | 2 | 444 | 479.7 | 449.7 [440.6-458.7] | 24.2 | 25.1 | 24.0 [23.7-24.2] | |
St.Jude Regent | 17 | – | 187 | 209.3 | – | 15.9 | 16.9 | – |
19 | – | 239 | 270 | – | 17.8 | 18.6 | – | |
21 | 5 | 290 | 328.3 | 328.0 [319.6-342.5] | 19.6 | 20.6 | 20.3 [19.9-21.2] | |
23 | 9 | 345 | 394.1 | 386.2 [381.0-395.8] | 21.4 | 22.5 | 22.2 [22.0-22.6] | |
25 | 7 | 402 | 461.7 | 447.5 [439.5-469.5] | 23.0 | 24.3 | 23.9 [23.4-24.1] | |
27 | 2 | 469 | 530.6 | 525.5 [525.3-525.5] | 24.9 | 26.1 | 26.0 [25.9-26.1] | |
29 | 2 | 544 | 624.2 | 599.7 [599.4-599.7] | 26.8 | 28.4 | 28.1 [27.9-28.2] | |
St.Jude Masters | 25 | – | 309 | 359.4 | – | 20.4 | 21.2 | – |
27 | 2 | 367 | 428.7 | 405.4 [398.5-412.3] | 22.5 | 23.4 | 22.8 [22.4-23.2] | |
29 | – | 441 | 505.3 | – | 24.2 | 25.3 | ||
31 | 5 | 518 | 583.1 | 552.7 [535.2-576.7] | 26.1 | 27.2 | 26.9 [26.3-27.2] | |
33 | 3 | 518 | 586.7 | 566.3 [559.4-569.6] | 26.1 | 27.1 | 27.0 [26.7-27.1] | |
St.Jude HP | 21 | 1 | 255 | – | 270.6 | 18.6 | – | 18.3 |
St.Jude Silzone | 23 | 1 | n/a | – | 333.7 | n/a | – | 20.8 |
St.Jude CAVG | 25 | 2 | 309 | – | 267.3 [256.0-267.3] | 20.4 | – | 18.2 [18.0-18.3] |
Sorin Slimline | 19 | – | 233 | 209.9 | – | 17.6 | 16.2 | – |
21 | – | 291 | 260.6 | – | 19.6 | 18.4 | – | |
23 | 1 | 355 | 321.9 | 317.2 | 21.7 | 20.3 | 19.9 | |
25 | 2 | 426 | 388.9 | 368.4 [346.1-390.6] | 23.8 | 22.1 | 21.1 | |
27 | – | 512 | 474.6 | – | 26.1 | 24.7 | – | |
Sorin Fitline | 25 | 1 | 355 | 326.0 | 303.0 | 21.3 | 20 | 19.9 |
27 | – | 426 | 388.2 | – | 23.3 | 22.6 | – | |
29 | 2 | 512 | 470.4 | 483.8 [476.8-490.8] | 25.6 | 24.3 | 24.9 [24.2-25.6] | |
31 | 1 | 512 | 472.0 | 452.6 | 25.6 | 24.5 | 23.7 | |
33 | – | 512 | 473.4 | – | 25.6 | 24.6 | – | |
ON-X | 19 | – | 222 | 290.4 | – | 17.4 | 19.1 | – |
21 | 1 | 279 | 354.7 | 333.6 | 19.4 | 21.3 | 20.4 | |
23 | 3 | 342 | 439.9 | 435.5 [429.9-439.9] | 21.4 | 23.3 | 23.2 [23.0-23.4] | |
25 | 5 | 409 | 504.0 | 503.8 [491.9-525.5] | 23.4 | 25.3 | 25.4 [24.9-25.8] | |
27/29 | 3 | 409 | 505.6 | 494.3 [491.0-503.2] | 23.4 | 25.1 | 25.1 [25.0-25.6] | |
31/33 | – | 409 | 501.4 | – | 23.4 | 25.3 | – | |
Medtronic-Hall | 20 | 1 | 201 | – | 186.5 | 16 | – | 14.9 |
22 | 7 | 254 | – | 220.2 [210.5-238.1 | 18 | – | 16.7 [16.1-17.0] | |
23 | 5 | 254 | – | 215.8 [212.1-222.3] | 18 | – | 16.6 [16.5-16.8] | |
25 | 3 | 314 | – | 266.3 [256.6-280.6] | 20 | – | 18.6 [18.6-18.8] | |
27 | 2 | 380 | – | 349.3 [342.4-356.1] | 22 | – | 20.8 [20.6-20.9] | |
29 | 1 | 452 | – | 402.8 | 24 | – | 22.8 | |
Duromedics | 21 | 2 | n/a | – | 178.1 [175.4-180.0] | n/a | – | 14.9 [14.3-15.5] |
23 | 1 | n/a | – | 241.3 | n/a | – | 17.3 | |
25 | 1 | n/a | – | 257.4 | n/a | – | 17.1 | |
29 | 1 | n/a | – | 394.0 | n/a | – | 22.0 | |
31 | 1 | n/a | – | 381.4 | n/a | – | 21.3 | |
ATS | 23 | 1 | 256 | – | 282.3 | 18.8 | – | 19.2 |
27 | 1 | 384 | – | 400.2 | 22.8 | – | 22.9 | |
Björk-Shiley | 19 | 2 | n/a | – | 125.7 [117.1-134.2] | n/a | – | Poor IQ |
23 | 1 | n/a | – | Poor IQ | n/a | – | Poor IQ | |
25 | 2 | n/a | – | 224.2 [213.4-234.9] | n/a | – | Poor IQ | |
29 | 1 | n/a | – | 455.2 | n/a | – | Poor IQ | |
31 | 1 | n/a | – | 381.1 | n/a | – | Poor IQ |
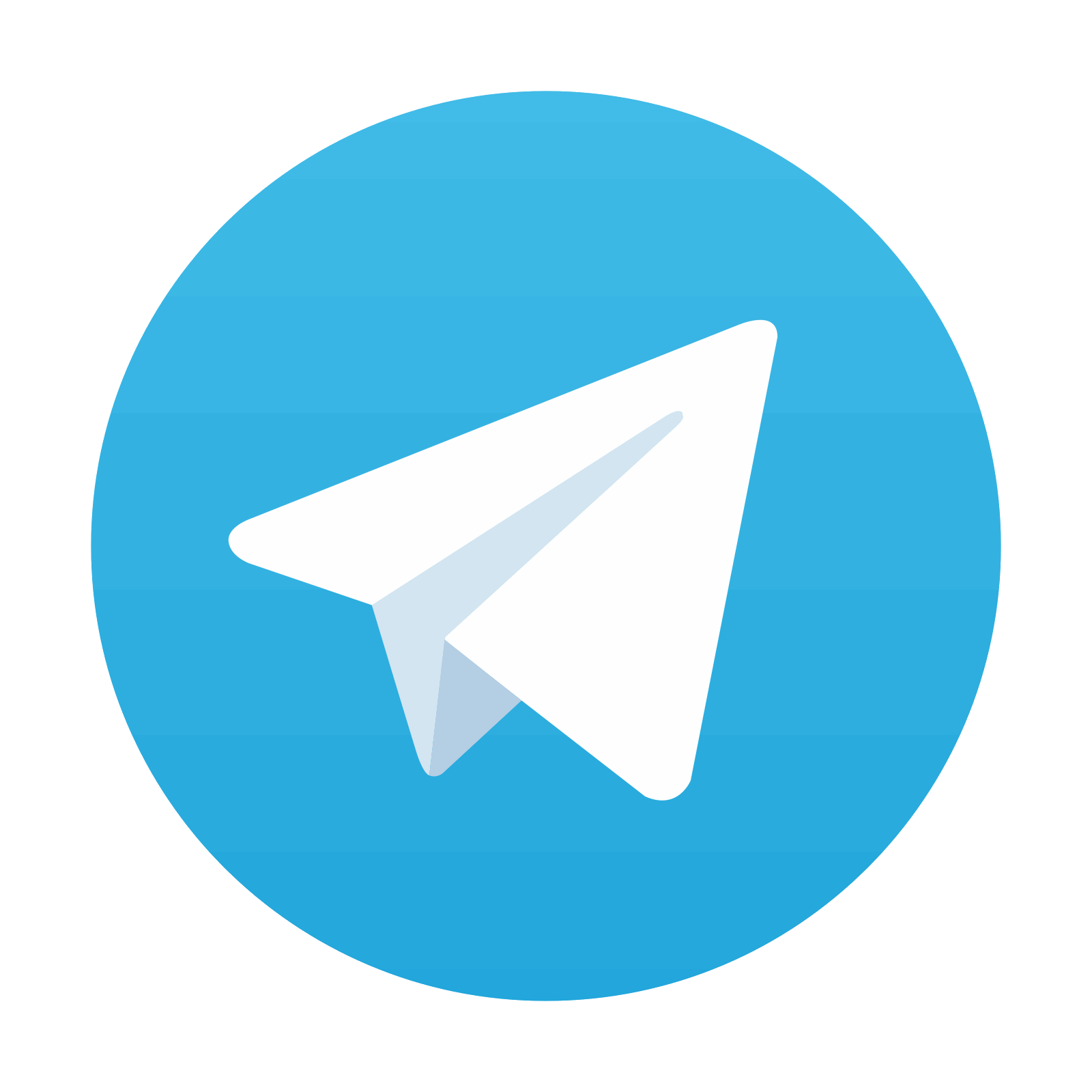
Stay updated, free articles. Join our Telegram channel

Full access? Get Clinical Tree
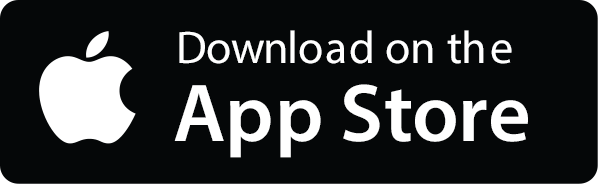
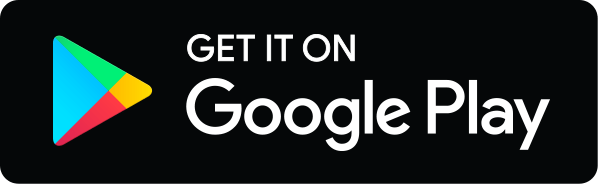
