The purposes of this study were to investigate whether heart failure (HF) with reduced ejection fraction (HFrEF) and heart failure with preserved ejection fraction (HFpEF) share echocardiographic predictors and elucidate E/(e′ × s′) that predicts major adverse cardiovascular events (MACE) independent of other echocardiographic parameters in each HF group. We assessed tissue Doppler-derived parameters [E/e′, E/(e′ × s′)] and left ventricular and right ventricular longitudinal strains in HFrEF (n = 340) and HFpEF (n = 102). Left ventricular and right ventricular longitudinal strains were significantly higher and E/(e′ × s′) was lower in patients with HFpEF compared with those with HFrEF, whereas E/e′ was similar between the groups. During a median follow-up of 342 days, MACE developed in 95 patients with HFrEF and 29 with HFpEF. The univariable analysis revealed similar echocardiographic predictors between the 2 groups, including E/e′, E/(e′ × s′) and pulmonary artery systolic pressure. No 2-dimensional speckle tracking–derived parameter remained significant in multivariable models in each HF group. E/(e′ × s′) was an only independent predictor with an incremental prognostic value over the Meta-analysis Global Group in Chronic Heart Failure score and was superior to the E/e′ ratio in both HFrEF and HFpEF. In conclusion, despite differences in echocardiography-based cardiac function parameters between HFrEF and HFpEF, these HF phenotypes shared the same echocardiographic predictors of future MACE. E/(e′ × s′) was an only independent predictor for future cardiac events in both HF populations.
Approximately half of the patients with heart failure (HF) have preserved left ventricular (LV) ejection fraction (HFpEF). Although there are apparent differences in clinical and cardiovascular features between HFpEF and heart failure with reduced ejection fraction (HFrEF), hospitalization risk and mortality were similarly poor. Similar to HFrEF, several echocardiographic parameters are associated with worse cardiovascular outcomes in HFpEF. A recent study demonstrated that E/(e′ × s′), an index combining systolic and diastolic Doppler parameters, was associated with increased risk of adverse cardiac events in both HFrEF and HFpEF. However, it remains unclear whether HFrEF and HFpEF share similar echocardiographic predictors of future adverse events. Thus, we conducted a multicenter prospective observational study (EVIDENCE; Predictive Indices of Echocardiography in Congestive Heart Failure) that addressed the prognostic value of echocardiographic variables in patients with HF. The purposes of this study were to investigate whether HFrEF and HFpEF share the same echocardiographic predictors and elucidate the E/(e′ × s′) that predicts major adverse cardiovascular events (MACE) independent of other echocardiographic parameters in each HF type.
Methods
The study population consisted of 475 patients who were hospitalized from July 2011 to December 2014 in one of 6 Japanese hospitals (Hospital of the University of Occupational and Environmental Health, Gunma University Hospital, St. Marianna University School of Medicine Hospital, Gunma Prefectural Cardiovascular Center, Showa University Fujigaoka Hospital, and Nagoya City University Hospital) due to HF. We excluded 33 patients because of more than mild mitral stenosis (n = 5), previous mitral valve surgery (n = 18), a repeat examination (n = 1), and poor-quality echocardiographic images (n = 9), leaving 442 participants in the final study population. The institutional ethics review board of each hospital approved this study, and informed consent was obtained from all patients.
HF was diagnosed using the standard definition. Patients were subsequently categorized as having HFpEF, if LVEF was ≥45% or as having HFrEF and if LVEF was <45% by echocardiography before their discharge. There were 340 HFrEF and 102 patients with HFpEF. Clinical HF risk was assessed using the Meta-analysis Global Group in Chronic Heart Failure (MAGGIC) project risk score.
Before their discharge, all patients underwent echocardiography examination with commercially available ultrasound systems (iE33; Philips Medical System, Andover, Massachusetts; Vivid 7, GE Healthcare, Horten, Norway; or Aplio 400; TOSHIBA Medical Systems, Otawara, Japan). The DICOM-formatted files were stored digitally and were analyzed using vendor-independent 2-dimensional (2D) speckle-tracking software (Epsilon Imaging Inc., Ann Arbor, Michigan). One experienced investigator performed all echocardiography measurements with speckle-tracking and conventional echocardiographic analysis (MO). For the measurement of LV global longitudinal strain (LVGLS), the endocardium was manually traced in 3 apical views, and the width of the region of interest was adjusted to fit the wall thickness. The software (“Cardio Oncology”) automatically performed speckle-tracking analysis on a frame-by-frame basis and generated time domain regional longitudinal strain curves. This software also provided LV end-diastolic and end-systolic volumes and the LVEF using the biplane method of disk formula ( Supplementary Figure 1 ). Next, the right ventricular (RV) endocardial border was traced in an RV-focused apical 4-chamber view and another software (RV) automatically measured the RV longitudinal free wall strain, RV fractional area change, and tricuspid annular plane systolic excursion ( Supplementary Figure 1 ). The RV free wall longitudinal strain was determined by averaging end-systolic longitudinal strain in 3 RV free wall segments. To avoid unnecessary confusion, we have reported the absolute values of LV/RV longitudinal strains in this article, figures, and tables in accordance with definitions from the standardization task force. Maximum LA volume was calculated with the biplane area-length method. The early filling (E wave) and peak late diastolic (A wave) velocities and deceleration times were obtained from transmitral flow. The peak systolic (s′), early diastolic (e′), and late diastolic (a′) mitral annular velocities were recorded at the septal corner of the mitral annulus. The ratio of early mitral diastolic inflow velocity to early diastolic mitral annular velocity (E/e′) was calculated, and we obtained E/(e′ × s′) index, as previously described. Mitral regurgitation (MR) was quantified using the vena contracta width from either the parasternal long-axis view or the apical long-axis views, and MR severity was grouped into 4 groups (0 to 3). Diastolic dysfunction was categorized into 4 groups according to previous reports.
Intraobserver and interobserver intraclass correlation coefficient (ICC) and percent variability for strain measurements and Doppler-derived parameters were assessed in 20 randomly selected patients by the same observer (MO) and another experienced reader (MT). The intraobserver variabilities were as follows: LVGLS, 5.2%; RV free wall strain, 3.9%; E/e′ ratio, 4.0%; and E/(e′ × s′), 5.3%. The corresponding interobserver variabilities were 7.3%, 5.8%, 6.5%, and 6.7%, respectively. The intraobserver ICCs were 0.98, 0.98, 0.99, and 0.99, respectively. The corresponding interobserver ICCs were 0.96, 0.98, 0.97, and 0.98, respectively.
Follow-up information was obtained by clinical visits or telephone interviews. The primary end point was a composite of MACE (cardiovascular death, nonfatal myocardial infarction, and HF exacerbation). Myocardial infarction was defined as acute onset of prolonged chest pain with a development of new electrocardiographic changes and elevation of cardiac biomarkers of myocardial necrosis (troponin I). HF exacerbation was defined as dyspnea, accompanied by pulmonary edema or congestion on chest x-ray requiring hospitalization. Death was classified as noncardiac if cardiovascular events were excluded as a cause of death.
All continuous variables are presented as means ± SD, unless otherwise specified. Comparisons between the groups were assessed by the Fisher’s exact test, Student’s t test, or Mann-Whitney U test. We assessed survival-free status using the Kaplan-Meier analysis, and the prognostic values of echocardiographic variables were determined using the Cox proportional hazards model. Because E/e′ and E/(e′ × s′) were closely related to each other and colinear (r = 0.89, p <0.001), we did not add them together in the same multivariable model. Because the number of MACE was limited in HFpEF, we tested the robustness of echocardiographic parameters in progressive models, as previously described. The proportional hazards assumption, assessed by the goodness-of-fit approach for each independent variable, was not violated. The incremental value was assessed by comparing the global chi-square values for each model. There were 17 missing data (3.8%) in MAGGIC score, which were handled by multiple imputation using predictive mean matching. A 2-sided p value <0.05 was accepted as indicating statistical significance. All data were analyzed using SPSS, version 23.0 (SPSS, Chicago, Illinois) and MedCalc, version 12.5.0.0 (MedCalc Software, Mariakerke, Belgium).
Results
Clinical characteristics of the study patients are listed in Table 1 . As expected, patients with HFpEF had significantly smaller LV end-diastolic and end-systolic volume indexes but had a larger LV stroke volume index, LVEF, and LVGLS than those with HFrEF. The RV systolic functional parameters were also higher in HFpEF. Although patients with HFpEF had significantly higher E-wave, A-wave, e′ and s′ velocities, and larger LA volume compared with those with HFrEF, there was no difference in the E/e′ ratio between the groups. Patients with HFpEF had a significantly lower E/(e′ × s′) index and higher pulmonary artery systolic pressure (PASP) than those with HFrEF. The differences between HFpEF and HFrEF in all echocardiographic parameters remained significant after adjusting for age, gender, and heart rate, except for tricuspid annular plane systolic excursion and A-wave velocity.
Variable | HFrEF (n = 340) | HFpEF (n = 102) | p Value |
---|---|---|---|
Age (years) | 71 ± 14 | 77 ± 11 | 0.001 |
Women | 119 (35%) | 58 (57%) | <0.001 |
Body mass index (kg/m 2 ) | 22.1 ± 3.9 | 21.6 ± 3.6 | 0.538 |
Hypertension | 219 (64%) | 70 (69%) | 0.477 |
Diabetes mellitus | 118 (35%) | 30 (29%) | 0.341 |
Coronary artery disease | 128 (38%) | 27 (27%) | 0.044 |
Dyslipidemia | 109 (32%) | 25 (25%) | 0.177 |
Current smoker | 60 (18%) | 16 (16%) | 0.765 |
Atrial fibrillation | 96 (28%) | 33 (32%) | 0.457 |
Chronic obstructive pulmonary disease | 16 (5%) | 9 (9%) | 0.140 |
NYHA functional class (I, II, III, IV), (%) | 65/30/4/1 | 56/35/9/0 | 0.136 |
HF duration > 18 months | 92 (27%) | 31 (30%) | 0.530 |
Systolic blood pressure (mm Hg) | 123 ± 23 | 131 ± 28 | 0.013 |
Diastolic blood pressure (mm Hg) | 71 ± 14 | 69 ± 14 | 0.269 |
Heart rate (bpm) | 73 ± 16 | 66 ± 12 | <0.001 |
Creatinine (μmol/L) | 88 (71-129) | 90 (64-139) | 0.496 |
MAGGIC risk score | 23.1 ± 6.7 | 22.7 ± 6.7 | 0.578 |
LV end-diastolic volume index (ml/m 2 ) | 104 ± 41 | 68 ± 24 | <0.001 |
LV end-systolic volume index (ml/m 2 ) | 75 ± 35 | 34 ± 13 | <0.001 |
LV stroke volume index (ml/m 2 ) | 29 ± 10 | 34 ± 12 | 0.001 |
Cardiac index (l/min m 2 ) | 2.1 ± 0.7 | 2.2 ± 0.7 | 0.171 |
LV ejection fraction (%) | 30 ± 8 | 50 ± 4 | <0.001 |
LV global longitudinal strain (%) | 8.4 ± 2.7 | 14.3 ± 2.8 | <0.001 |
RV free wall strain (%) | 13.7 ± 6.1 | 17.6 ± 5.8 | <0.001 |
RV fractional area change (%) | 24 ± 9 | 28 ± 9 | 0.001 |
Tricuspid annular plane systolic excursion (cm) | 1.20 ± 0.56 | 1.36 ± 0.50 | 0.009 |
E-wave (cm/sec) | 80 ± 31 | 94 ± 32 | <0.001 |
A-wave (cm/sec) | 74 ± 29 | 85 ± 27 | 0.006 |
e′ velocity (cm/sec) | 4.5 ± 1.7 | 5.4 ± 2.2 | <0.001 |
a′ velocity (cm/sec) | 6.2 ± 2.4 | 6.6 ± 2.8 | 0.458 |
s′ velocity (cm/sec) | 4.4 ± 1.5 | 5.3 ± 1.5 | <0.001 |
E/e′ ratio | 19.8 ± 10.2 | 19.7 ± 9.5 | 0.955 |
E/(e′ x s′ ) (sec/cm) | 5.4 ± 4.4 | 4.3 ± 3.1 | 0.012 |
Maximum LA volume index (ml/m 2 ) | 57 ± 37 | 69 ± 50 | 0.015 |
Mitral regurgitation grade, (0-3) | 0.8 ± 0.7 | 0.9 ± 0.8 | 0.758 |
Mitral regurgitation vena contracta width (mm) | 2.3 ± 1.8 | 2.4 ± 2.0 | 0.833 |
Diastolic dysfunction grade (%) indeterminate/0/1/2/3 | 20/5/17/37/21 | 25/7/12/43/13 | 0.171 |
Pulmonary artery systolic pressure (mm Hg) | 31 ± 12 | 37 ± 13 | <0.001 |
Beta-blockers | 246 (72%) | 54 (53%) | <0.001 |
ACEIs/ARBs | 256 (75%) | 63 (62%) | 0.011 |
Diuretics | 271 (80%) | 70 (69%) | 0.023 |
Calcium channel antagonists | 96 (28%) | 48 (47%) | 0.001 |
Statin | 109 (32%) | 24 (24%) | 0.110 |
Antiplatelets | 141 (42%) | 36 (35%) | 0.300 |
Oral anticoagulants | 125 (37%) | 45 (44%) | 0.202 |
During a median follow-up of 342 days (interquartile range 160 to 644), there were 124 MACE (HFrEF = 95, HFpEF = 29) including 46 cardiac deaths (HFrEF = 36, HFpEF = 10), 2 nonfatal myocardial infarctions (HFrEF = 2, HFpEF = 0), and 96 HF hospitalizations (HFrEF = 71, HFpEF = 25). All-cause deaths developed in 78 patients (HFrEF = 62, HFpEF = 16). Kaplan-Meier analyses showed that MACE-free survival was significantly decreased in a group of patients with an E/(e′ × s′) above the median values for each HF phenotype ( Figure 1 ). In addition to E/(e′ × s′), several parameters, including E-wave velocity, s′, E/e′, and PASP were statistically significant predictors of MACE in both HF phenotypes ( Table 2 ). LVGLS, e′, and a′ were significantly associated with increased risk of MACE in patients with HFrEF but not in those with HFpEF. RV functional parameters were not predictive of MACE in either HF group although tricuspid annular plane systolic excursion (TAPSE) showed a borderline significance in HFrEF. Multivariable analyses revealed that E/(e′ × s′) was an only independent predictor of MACE in both HFrEF and HFpEF ( Table 3 ). E/e′ also remained significant after adjusting for MAGGIC score, LVGLS, TAPSE, LA volume index, MR vena contracta width, and PASP in HFrEF (p = 0.007), whereas it showed a borderline significance after adjusting for MAGGIC score and PASP in HFpEF (p = 0.055).
Variable | HFrEF | HFpEF | ||
---|---|---|---|---|
Hazard Ratio (95% CI) | p Value | Hazard Ratio (95% CI) | p Value | |
LV end-diastolic volume index (1ml/m 2 ) | 1.00 (0.99-1.01) | 0.354 | 0.99 (0.98-1.01) | 0.705 |
LV end-systolic volume index (1ml/m 2 ) | 1.00 (0.99-1.01) | 0.332 | 0.99 (0.96-1.02) | 0.633 |
LV stroke volume index (1ml/m 2 ) | 1.00 (0.99-1.02) | 0.674 | 0.99 (0.96-1.03) | 0.807 |
Cardiac index (1l/min m 2 ) | 1.15 (0.88-1.49) | 0.310 | 1.06 (0.63-1.76) | 0.834 |
LV ejection fraction (1%) | 0.99 (0.96-1.01) | 0.340 | 1.04 (0.96-1.12) | 0.324 |
LV global longitudinal strain (1%) | 0.92 (0.85-0.99) | 0.026 | 0.99 (0.87-1.13) | 0.920 |
RV free wall strain (1%) | 0.98 (0.94-1.02) | 0.252 | 1.00 (0.94-1.07) | 0.994 |
RV fractional area change (1%) | 0.98 (0.96-1.01) | 0.192 | 0.99 (0.96-1.04) | 0.968 |
Tricuspid annular plane systolic excursion (1cm) | 0.67 (0.44-1.02) | 0.063 | 0.90 (0.44-1.84) | 0.765 |
E-wave (1cm/sec) | 1.01 (1.00-1.01) | 0.040 | 1.02 (1.01-1.04) | <0.001 |
A-wave (1cm/sec) ∗ | 1.00 (0.99-1.01) | 0.926 | 1.00 (0.99-1.02) | 0.677 |
e′ velocity (1cm/sec) | 0.82 (0.71-0.95) | 0.006 | 0.91 (0.76-1.08) | 0.277 |
a′ velocity (1cm/sec) ∗ | 0.82 (0.72-0.93) | 0.003 | 0.88 (0.70-1.09) | 0.236 |
s′ velocity (1cm/sec) | 0.71 (0.60-0.84) | <0.001 | 0.68 (0.50-0.92) | 0.013 |
E/e′ ratio (1 unit) | 1.03 (1.02-1.05) | <0.001 | 1.05 (1.02-1.07) | <0.001 |
Maximum LA volume index (1ml/m 2 ) | 1.00 (0.99-1.01) | 0.097 | 1.00 (0.99-1.01) | 0.744 |
Mitral regurgitation grade (1 grade) | 1.37 (1.13-1.65) | 0.001 | 1.09 (0.79-1.53) | 0.596 |
Mitral regurgitation vena contracta width (1mm) | 1.14 (1.02-1.27) | 0.020 | 1.10 (0.93-1.30) | 0.276 |
Pulmonary artery systolic pressure (1mm Hg) | 1.02 (1.01-1.04) | 0.005 | 1.04 (1.02-1.07) | <0.001 |
E/(e′ x s′) index (1 sec/cm) | 1.08 (1.06-1.11) | <0.001 | 1.23 (1.13-1.34) | <0.001 |
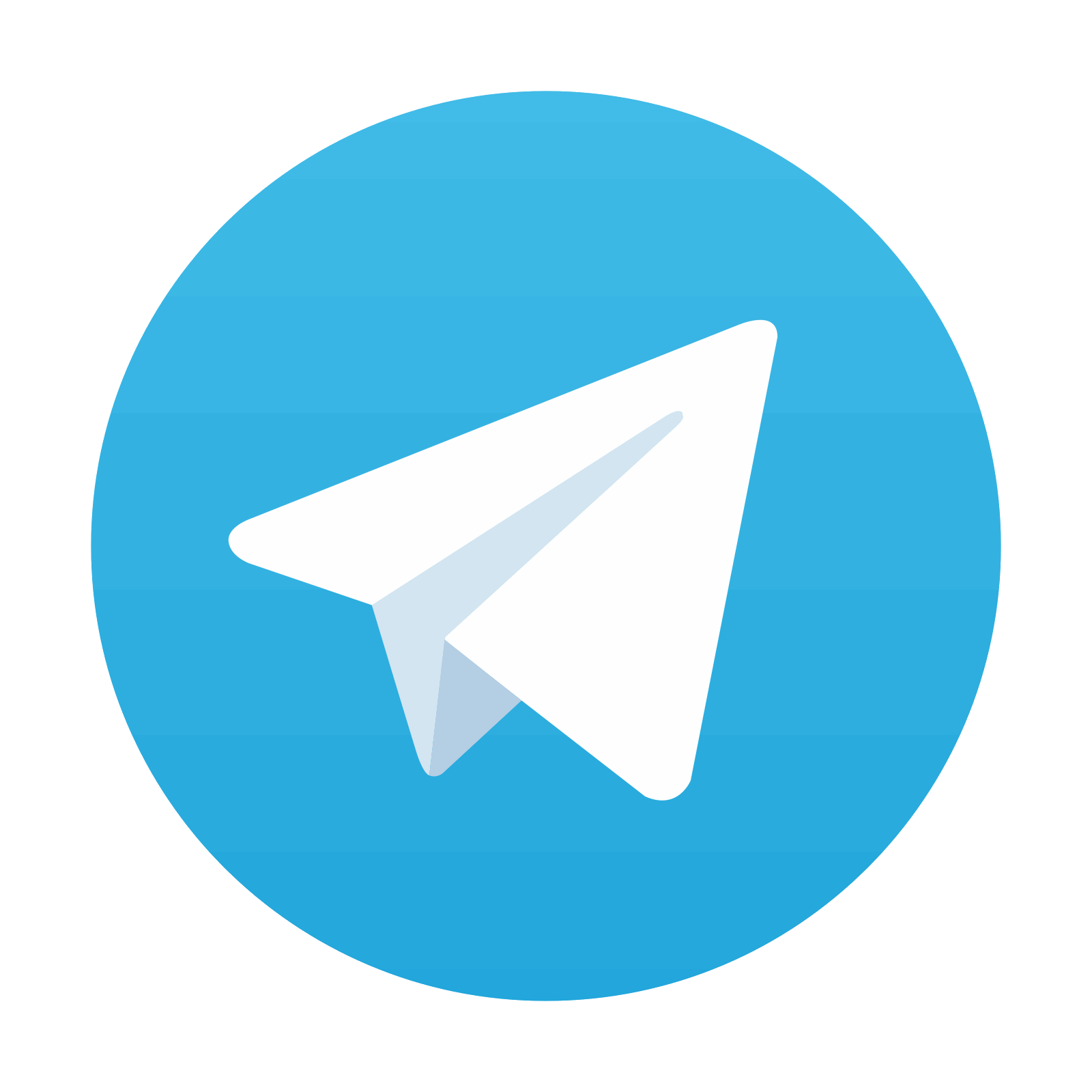
Stay updated, free articles. Join our Telegram channel

Full access? Get Clinical Tree
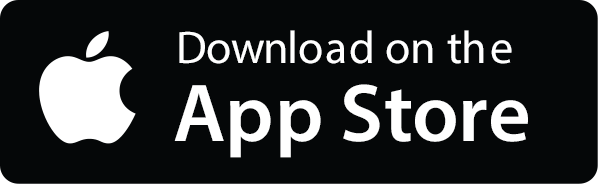
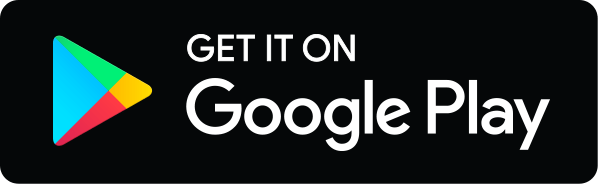
