Fig. 27.1
(a) Duplex ultrasound demonstrating moderate stenosis with an elevated peak systolic velocity of 200 cm/s in the midportion of SFA stent. (b) Duplex ultrasound demonstrating high-grade stenosis with an elevated peak systolic velocity of 540 cm/s in proximal portion of SFA stent. (c) Angiogram demonstrating high-grade stenosis in proximal SFA stent. (d) Angiogram following atherectomy and re-angioplasty with resolution of in-stent stenosis
For aortoiliac interventions, color-flow Doppler imaging is obtained from the distal aorta along the iliac arteries and through the common femoral arteries, the profunda femoris arteries, and the superficial femoral arteries. Spectral velocity waveforms are measured and waveforms and PSVs within treated segments are compared to velocities proximally in the native vessel. For femoropopliteal interventions, the common femoral artery, the femoral bifurcation, and the length of the superficial femoral artery, as well as the popliteal artery and proximal tibial vessels, are imaged. As with iliac interventions, spectral waveforms and PSVs within treated femoropopliteal arterial segments are compared to those in the segments proximally.
Methods of Surveillance Criteria Determination for Lower Extremity Endovascular Stenting
Previously, duplex and noninvasive criteria for the diagnosis of in-stent stenosis had been generalized from data concerning the detection of de novo lesions in previously untreated femoropopliteal vessels or in the detection of vein bypass graft stenosis. However, it has been shown that stent placement within an arterial segment results in a change in vessel compliance which may alter velocities as measured by duplex [22]. It has also been demonstrated that stent placement in the carotid circulation alters the velocity thresholds for the detection of significant recurrent internal carotid artery stenosis [23]. We have described criteria for the determination of in-stent stenosis after angioplasty and stenting of the superficial femoral artery and use these criteria for the follow-up of patients who have undergone endovascular lower extremity interventions [17]. As present time, there are no specific criteria for determination of in-stent restenosis after iliac interventions, and, by convention, data from studies of untreated vessels and native stenoses have been applied.
To determine criteria of in-stent stenosis after angioplasty and stenting of the superficial femoral arteries, we reviewed all endovascular interventions for femoropopliteal occlusive disease between May 2003 and May 2008, during which time 330 limbs underwent femoropopliteal angioplasty and stenting. Data pairs of duplex and angiographically measured stenoses within 30 days of each other were analyzed. Angiograms were obtained in anteroposterior and oblique views at the time of initial and secondary or tertiary imaging. The view demonstrating the greatest degree of stenosis was used to determine the percentage of in-stent stenosis.
Linear regression analyses were performed, and receiver operator characteristic (ROC) curves were used to compare angiographic stenosis with PSV and stented SFA velocity/proximal SFA velocity ratio to determine optimal criteria equating to ≥50 and ≥80% stenosis. A linear regression model of PSV versus degree of angiographic stenosis showed a strong adjusted correlation coefficient (R 2 = 0.60, P < 0.001). Additionally, there was a strong adjusted correlation coefficient (R 2 = 0.55, P < 0.001) for velocity ratio versus degree of angiographic stenosis but only a moderate adjusted correlation coefficient (R 2 = 0.31, P = 0.02) for decrease in ABI versus degree of angiographic stenosis (Fig. 27.2).

Fig. 27.2
Scatter plots of the peak systolic velocities (PSV) (a) and in-stent PSV to proximal superficial femoral artery PSV ratio (b)
To distinguish <50% stenosis from ≥50% stenosis, the cut point on the PSV ROC curve was 189 cm/s. Rounding this value to 190 cm/s was associated with a sensitivity of 88%, a specificity of 95%, a positive predictive value (PPV) of 98%, and a negative predictive value (NPV) of 72%. To distinguish a <50% stenosis from ≥50% stenosis, the cut point on the PSV ratio ROC curve was 1.55. Rounding this value to 1.50 was associated with a sensitivity of 93%, a specificity of 89%, a PPV of 96%, and a NPV of 81%. Combining a PSV ≥ 190 and a ratio ≥1.50 to determine a ≥50% stenosis was associated with a sensitivity of 85%, a specificity of 95%, a PPV of 98%, and a NPV of 67%. Additionally, the odds ratio OR for determining a ≥50% stenosis based on a PSV ≥ 190 and a velocity ratio ≥1.50 was 99.99 (95% CI 11.82–845.55, P < 0.001).
To distinguish <80% stenosis from ≥80% stenosis, the cut point on the PSV ROC curve was 265 cm/s. Rounding this value to 275 cm/s was associated with a sensitivity of 97%, a specificity of 68%, a PPV of 67%, and a NPV of 97%. To distinguish a <80% stenosis from ≥80% stenosis, the cut point on the PSV ratio ROC curve was 3.50. This value corresponded to a sensitivity of 74%, a specificity of 94%, a PPV of 77%, and a NPV 88%. Combining a PSV ≥ 275 and a ratio ≥3.50 to determine a ≥80% stenosis was associated with a sensitivity of 74%, a specificity of 94%, a PPV of 88%, and a NPV of 85%. Additionally, the odds ratio for determining a ≥80% stenosis based on a PSV ≥ 275 and a velocity ratio ≥3.50 was 42.17 (95% CI 10.20–174.36, P < 0.001) (Fig. 27.3).

Fig. 27.3
Receiver operator characteristic curves to differentiate between ≥50 and <50% stenosis in stented arteries by (a) PSV and (b) velocity ratio. Differentiation between ≥80 and <80% stenosis in stented arteries by (c) PSV and (d) velocity ratio
Additional Studies to Determine Criteria for Lower Extremity Endovascular Stenting
With regard to in-sent restenosis of the femoropopliteal artery, we have found, as described above, a PSV > 275 cm/s or Vr > 3.5 to be highly specific and predictive of an 80% stenosis.
In a subsequent study by Shrikhande et al. [24], similar methodology was utilized to attempt to define duplex criteria for restenoses following endovascular intervention. In this study, repeat angiograms were performed on 345 lesions in 143 patients, and 254 lesions in 103 patients had a corresponding duplex ultrasound. This review included femoropopliteal as well as tibial lesions . Additionally, this study included both stented (70%) and nonstented (30%) arteries. PSV was found to have a strong correlation for angiographic stenosis in the femoropopliteal segment but not in tibial vessels. Specifically, ROC curve analysis showed that to detect ≥70% stenosis in the SFA, a PSV ≥ 204 cm/s had a sensitivity of 97.6% and specificity of 94.7%. To detect ≥70% stenosis in the overall femoropopliteal region, a PSV ≥ 223 cm/s had a sensitivity of 94.1% and specificity of 95.2%. Additionally, velocity ratios were evaluated, and it was found that for a Vr < 2.0, 12.8% had >70% angiographic stenosis; for lesions with ratios from 2 to 2.5, 92.3% had >70% angiographic stenosis; and for lesions with ratios >2.5, 92.0% had >70% angiographic stenosis. This study did not specifically combine the two criteria which we have shown to have the strongest predictive ability.
More recently, a study by Troutman et al. [25] reported on duplex criteria of failing stent grafts. This review evaluated 142 stent grafts in 92 arterial segments in patients who underwent at least one surveillance duplex. Stent grafts were placed in 29 iliac and 52 femoropopliteal arteries and in 11 failing infrainguinal bypass grafts . In retrospective fashion, lesions were then classified as having abnormal duplex ultrasound findings if they had the following: focal PSVs > 300 cm/s, uniform PSVs < 50 cm/s throughout the graft, and a Vr > 3.0. Fifteen of 20 patients with one or more of these abnormal duplex findings underwent prophylactic intervention (n = 8) or occluded without intervention (n = 7), whereas only 2 of 72 with normal duplex ultrasound findings occluded (P = 0.0001). When the 8 patients who underwent prophylactic intervention were excluded, 7 of 12 patients with abnormal duplex ultrasound findings occluded without intervention compared to 2 of 72 with normal findings (P = 0.0001). Based on these findings, the authors suggest that follow-up duplex ultrasound surveillance can predict failure of stent grafts placed for lower extremity occlusive disease specifically when the following criteria are utilized: focal PSVs > 300 cm/s, Vr > 3.0, and most importantly, uniform PSVs < 50 cm/s throughout the stent graft.
Clinical Benefits of Duplex Surveillance
From the detailed hemodynamic and anatomic data provided by duplex examination, there are multiple clinical benefits obtained from duplex surveillance of lower extremity interventions. As with surveillance following surgical bypass, benefits may be seen over the course of the surveillance period. Early technical issues may be recognized on immediate post-procedure duplex studies, and later, progression of disease of the treated segment and failing interventions may be identified. Additionally, progression of proximal and distal disease may be detected via surveillance.
In the immediate post-procedure period (<1 month), duplex has been demonstrated to detect abnormalities more frequently than completion angiography [26]. Following intervention, intimal flaps, dissections, fractured plaques, and residual stenoses may be missed on angiography, particularly if multiplanar imaging has not been judiciously used. Given this, reports have even advocated for performing endovascular interventions using primarily duplex imaging at the time of the procedure rather than angiography [27]. Furthermore, abnormal duplex findings on post-procedure imaging have been associated with increased rates of adverse outcomes. Specifically, patients with abnormalities on post-procedure duplex imaging when compared to patients with normal post-procedure studies have been shown to have lower primary patency rates and higher rates of amputation [26, 28, 29]. Additionally, there has been good clinical correlation seen with duplex findings as in the study by Jones et al. wherein the median peak systolic velocity ratio was higher in patients with symptom recurrence (2.99 symptomatic vs. 1.69 asymptomatic; P < 0.001) in the femoropopliteal segment in patients treated for claudication [30]. Furthermore, duplex has been demonstrated to be a useful adjunct in combination with clinical examination and hemodynamic measurements, whereby up to one-third of significant lesions may be missed if these modalities are used without concomitant duplex imaging [31].
In addition to surveillance of the treated segment, duplex imaging after endovascular interventions is beneficial in recognizing lesions proximally and distally. This has been shown particularly in the iliac arteries, where up to 44% restenoses occur above or below a treated segment and can be recognized by duplex surveillance [32]. Additionally, duplex surveillance of iliac lesions has demonstrated even greater utility in patients with concomitant outflow reconstructions, a population who is at greater risk for intervention failure [28]. With regard to femoropopliteal lesions, the natural history of stenoses treated using endovascular means and the progression of disease in untreated segments proximally and distally remain poorly elucidated, again, emphasizing the need for surveillance of the entire artery and the neighboring arterial segments.
Future Directions
Long-term durability of lower extremity endovascular interventions remains an issue which has plagued the advancing technology. Predictors of restenosis and failure of endovascular interventions include anatomic factors such as occlusions, long lesions, and multilevel disease along with patient factors including hypercholesterolemia, hypertension, diabetes mellitus, and renal failure [15, 33–35]. Additional factors that are likely to contribute to decreased long-term patency of endovascular interventions include the degree of calcification, plaque ulceration , and other morphologic characteristics. These morphologic factors have not been fully studied and are not routinely incorporated into pre- or post-procedure studies; however, further investigation may lead to identifying plaque characteristics on pre- and post-procedure duplex imaging which may portend poorer longer-term outcomes and failure [36].
With regard to treated lesions, their long-term natural history also remains unclear. Although restenosis is a well-known complication of endovascular interventions, there are various patterns of restenosis in both the aortoiliac and femoropopliteal segments. With this in mind, particular questions remain unanswered. In particular, do mid-stent restenotic lesions have a different natural history than stent margin lesions and do anatomic location of a stent influence the surveillance data? With the use of evolving technology including drug-coated balloons and drug-eluting stent lesion recurrences as well as increasing use of covered stents, there may be a decrease in the rates of in-stent restenosis, but the risk of stent margin stenosis will remain. Along with this, more detailed duplex criteria might be applicable to help define these stenoses (Fig. 27.4).

Full access? Get Clinical Tree
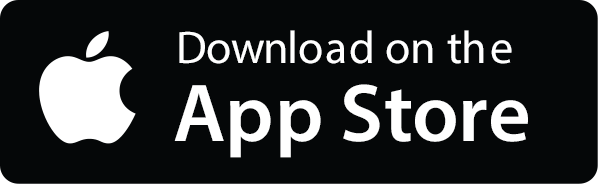
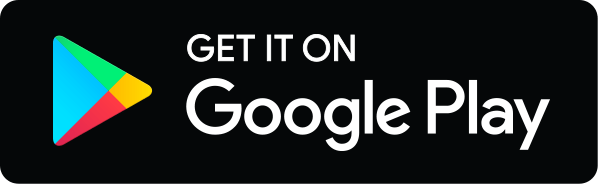