This chapter discusses the use of the Doppler principle and the modified Bernoulli equation for the assessment of gradients and regurgitations caused by stenotic or insufficient valves, as well as the evaluation of physiologic or pathologic communications within the circulatory system. These principles are applied to clinical bedside decision making in the context of neonatal functional echocardiography.
An important application of echocardiography is its use in analyzing the direction and velocity of blood flow within the heart and the blood vessels, and the difference in pressure values (pressure gradient) between these chambers. As described in Chapter 1, these measurements are possible thanks to the Doppler principle and the Bernoulli equation. The Doppler principle is used to derive information about velocity of blood flow, and this information can then be applied to the Bernoulli equation to extrapolate an estimation of pressure gradients.
The Doppler principle states that, to a stationary observer, the original frequency of sound emitted from a source is altered when the source of the sound is moving. The difference in frequency is called the Doppler shift. For echocardiography, the stationary observer is the transducer, emitting the ultrasound waves at a given frequency and receiving the returning ultrasound waves. The red cells within the bloodstream are the moving objects that reflect the incident sound waves and therefore serve as the “source” responsible for producing the Doppler shift. The returning signals will have a higher frequency (positive numerical value) if the red cells are moving toward the transducer, and a lower frequency (negative numerical value) if moving away from the transducer (Figure 6-1).
The Doppler shift (or frequency difference) is calculated using the following formula:
where:
Fd = Doppler shift (frequency difference)
2 = Factor used because there are two shifts: one because the wave incident on the moving red blood cells is Doppler shifted and an additional shift because the reflection is from a moving object.
Ft = Frequency of transmitted beam (transducer frequency)
V = Velocity of the blood
Cos θ = Cosine of angle of incidence (insonation) between the ultrasound beam and the blood flow
C = Speed of sound in human tissue (1540 m/sec or m/s)
The importance of the angle of incidence θ becomes obvious when one considers the following:
For any angle between 0° and 90°, the cosine of the angle returns a value <1, which will result in a lower Doppler shift and resultant error, for example:
Cosine of 20∘ is 0.94 and that means it adds a 6% error.
Cosine of 30∘ is 0.86 (14% error).
Cosine of 40∘ is 0.76 (24% error).
Therefore, the greater the angle of incidence, the larger is the error and the more the velocity will be underestimated.
Once the Doppler shift is measured, the Doppler formula can be rearranged to solve for velocity:
All modern echocardiography systems offer the technology to perform Doppler evaluation of moving structures, such as blood. Several different Doppler modalities are available (as described below). With this technology, the following information can be obtained:
If there is blood flow (sound, color flow, and spectral Doppler)
The direction of blood flow (color flow and spectral Doppler)
The magnitude of blood flow velocity (color flow Doppler for a qualitative visual assessment, spectral Doppler for a quantitative estimation of velocity)
Once velocity of flow is determined, the modified Bernoulli formula is applied to calculate a pressure difference (gradient or drop) by relating velocity to pressure. Bernoulli’s principle states: “As the velocity of a fluid increases, the pressure exerted by that fluid decreases.” Higher velocities are therefore associated with a greater pressure drop, resulting in a larger pressure gradient. According to the simplified Bernoulli equation, velocity can be related to change in pressure by the following formula:
where ΔP is the pressure gradient and V is flow velocity.
The Doppler-measured flow values can be displayed by the ultrasound machine as a graph of time on the x-axis versus velocity (meters/second) on the y-axis; this method of display is commonly known as spectral Doppler. By convention, positive values are represented above the baseline and negative values are represented below the baseline. The two spectral Doppler modes are pulsed wave (PW) and continuous-wave (CW). PW Doppler offers the advantage of being able to evaluate a Doppler velocity at any point on the two-dimensional (2D) echocardiographic image. However, this requires range gating, in which the selected area of interest (also known as a sample volume) is localized a specific distance from the transducer, as calculated from the transmit/receive time of an emitted pulse of sound (assumed speed of sound 1540 m/s). Only the signals from the selected area are evaluated for Doppler shifts. This dependence on awaiting the return of the range-gated signals results in an upper velocity limit for PW Doppler that depends on the echocardiography system, the depth of the selected area of interest, and the frequency of the transducer (lower-frequency transducers can detect higher velocities). On the other hand, CW Doppler is not range-gated. Therefore, CW can be aimed in a direction but not at any pinpoint location in that direction. CW Doppler detects velocities all the way along its path and does not have an upper limit to detectable velocities. The highest velocity along that path produces the highest velocity on the spectral tracing.
Color flow Doppler presents a visual map of mean Doppler velocities, shown as colors, superimposed upon a concurrently obtained 2D image. The color represents moving blood within the heart or a vascular structure. With color flow Doppler, assignment of color to velocities is usually based upon direction (red toward transducer and blue away from it) and magnitude (different color hues, with higher velocities shown as lighter saturations). This type of Doppler is extremely useful for obtaining a comprehensive, global picture of blood flow within the heart or a vascular structure. It is particularly useful for overall assessment of valvar pathology and intracardiac shunts, and plays an invaluable role in directing spectral Doppler evaluation.
It is important to remember that the ultrasound machine is not capable of directly detecting or measuring pressures and velocities; direct noninvasive measurement of pressure in any cardiac chamber is not possible. Therefore, what is ultimately determined by echocardiography is not an absolute pressure value, but a pressure difference (gradient or drop). The presence of gradients and regurgitation caused by stenotic or insufficient valves, as well as physiologic or pathologic communications within the circulatory system, allows the observer to extrapolate values of pressures. Stenotic or regurgitant valves, as well as intra- or extracardiac shunts, create pressure gradients that in turn are responsible for the velocity changes detected by Doppler. By properly analyzing the Doppler information, one can gain important hemodynamic as well as structural information.
Diseases of the cardiac valves can produce total absence of flow across the valve (atresia) or varying degrees of narrowing (stenosis), backflow (regurgitation/insufficiency), or a combination of stenosis/regurgitation. Doppler echocardiography is used to interrogate the valves and obtain a numerical value for flow velocity in m/s. Then, by using the simplified Bernoulli equation, the echocardiography machine translates this velocity into a pressure difference (gradient).
The best views to interrogate each valve are as follows:
Mitral valve: Apical four chamber, left parasternal long axis
Aortic valve: Apical four chamber, subcostal sagittal and coronal, suprasternal
Tricuspid valve: Apical four chamber, left parasternal long axis, parasternal right ventricular inflow, left parasternal short axis at aortic valve level
Pulmonic valve: Parasternal long and short axis, subcostal sagittal and coronal
Valvar stenosis/atresia and their evaluation will not be extensively discussed, since the detection of these conditions in a neonate should prompt a pediatric cardiology consultation. However, given below are brief discussions of the various type of valvar pathologies.
Mitral and tricuspid valve stenosis can be found as isolated conditions in an otherwise anatomically normal heart, but they are extremely rare conditions. In contrast, both mitral and tricuspid valve atresia (atresia being defined as complete lack of patency with no flow across the valve plane) are always associated with other significant cardiac anomalies.
Aortic and pulmonic valve atresia are, for practical purposes, always associated with other cardiac malformations. In contrast, mild to moderate pulmonic stenosis—in the absence of any other associated cardiac anomaly—is the most common isolated valvar disease in the neonate, and its presence or absence must be considered when right ventricular pressure (RVP) is determined with the TR jet.
The most common aortic valve abnormality found in newborn patients is a bicuspid aortic valve. However, most bicuspid aortic valves are neither stenotic nor regurgitant, and might not become hemodynamically significant until later in life. Isolated aortic valve stenosis due to an abnormal aortic valve (which can be tricuspid, bicuspid, or unicuspid) can be found in the neonate, though it is an uncommon. When encountered, the clinical severity depends upon the degree of aortic valve stenosis—from mild (patients are asymptomatic and present only with a murmur) to critical (patients are dependent upon a patent ductus arteriosus for survival).
The severity of semilunar valve stenosis is based upon:
The Doppler-derived numerical value of the flow velocity (in m/s) across the valve
The same value (flow velocity) translated into a pressure gradient expressed in mmHg, using the simplified Bernoulli equation (4 × Velocity2)
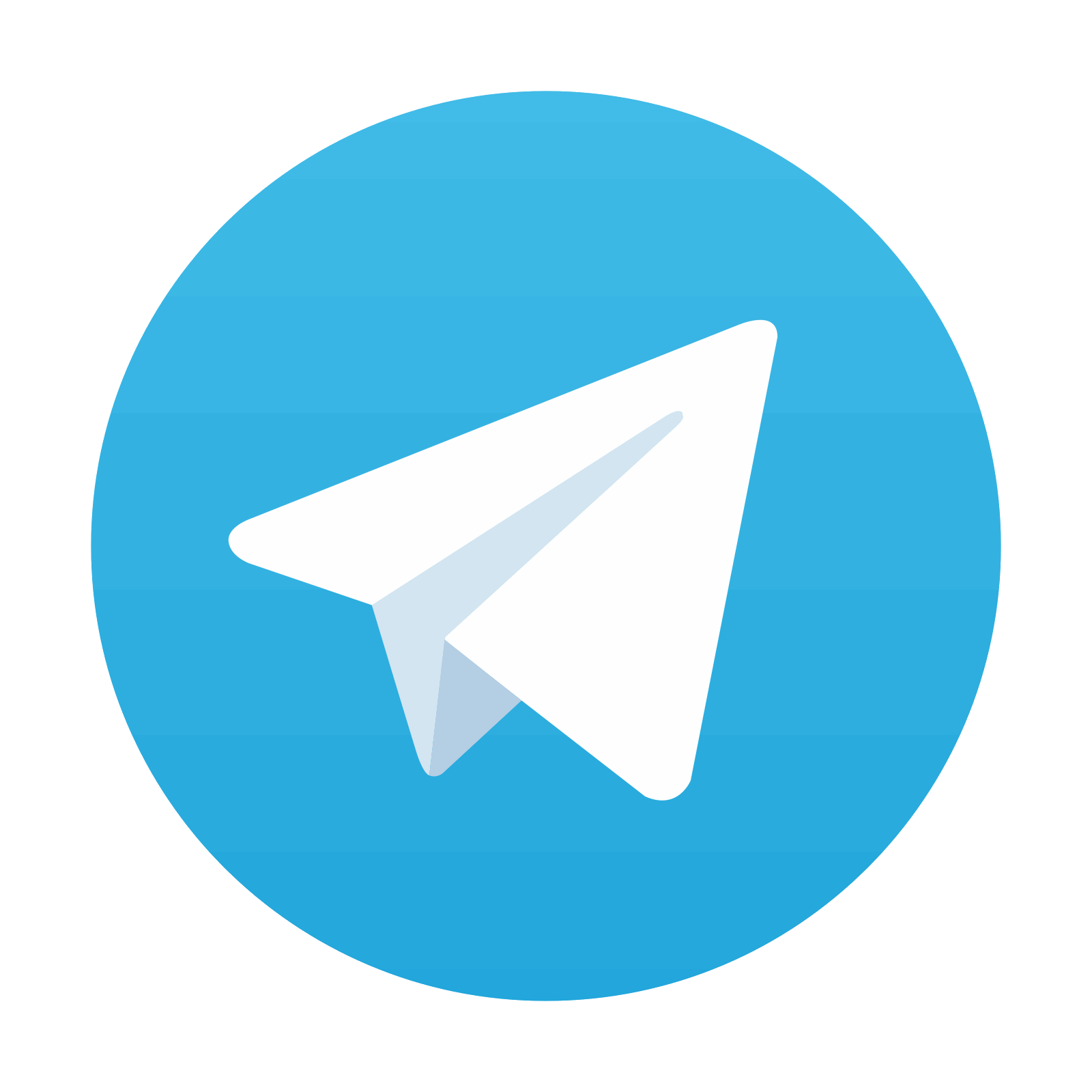
Stay updated, free articles. Join our Telegram channel

Full access? Get Clinical Tree
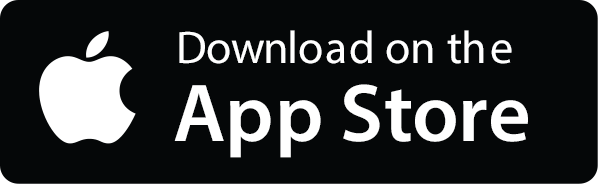
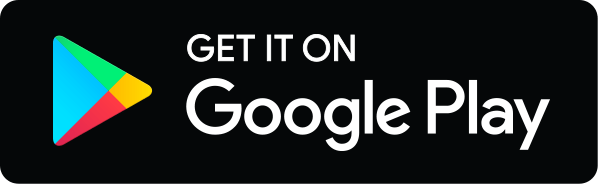