Ultrasound machines have greatly evolved during the last few decades. Bulky machines with limited capability have been transformed to much smaller units with enhanced capability of performing all modalities of echocardiography that we take for granted at present (Figure 2-1). Portable and even handheld devices have capabilities unimaginable 20 to 30 years ago. These units are technologically advanced, enabling performance of high-quality, complete studies. Regardless of their sizes, all have basically the same components and operate in the same manner.1,2
Ultrasound machines have two major components: the transducer (or probe) and the main unit.
The transducer is the part of the system that generates and sends out ultrasound pulses, and then receives echoes coming back from the target (Figure 2-2). These sound waves are shaped via the transducer as beams that are focused and electronically scanned through tissues. This process is done by converting electrical energy into ultrasonic (mechanical) energy, and vice versa. The element that facilitates this conversion of energy is PZT, a ceramic plate made of lead, zirconium, and titanium. PZT has piezoelectric properties, which means that applied voltage deforms the material, causing it to vibrate and generate ultrasonic waves, and reflected ultrasound received by the PZT element(s) produces voltage changes that are processed and converted to echocardiographic images (Figure 2-2). Since transducer elements are solid and have high acoustic impedance, if no compensation is made for a much lower impedance of skin and tissues, most ultrasound energy will be reflected and little will be transmitted to the target organs, resulting in virtually no usable information (see Chapter 1). To prevent this, and to facilitate ultrasound transmission, the face of the transducer is covered by a matching layer with an impedance value intermediate to the transducer and tissue. A backing layer is also applied to absorb the extra sounds, thus keeping the pulses short. This will ease the transfer of pulses between the transducer and the patient, and vice versa. The transducer element is then placed in a casing and covered by a lens to enable more effective transmission and reception from a particular region. Figure 2-3 demonstrates the basic structure of a single-element transducer.
In older transducers, beam forming and scanning were done by rotating or swinging the transducer element inside the probe (mechanical transducers). For the new generation of transducers, multiple elements (arranged in an array) are used in each probe and the ultrasonic beam is formed electronically by delaying the signals to and from each element. These phased array scanners contain a large number of elements (up to 128) and come in a variety of shapes and sizes, depending upon the usage. For cardiac use, the elements are placed very close to each other and provide a sector scanning format, which yields a pie-shaped sector.
The operating frequency of the transducer is determined by the propagation speed and the thickness of its element. For diagnostic ultrasound, the thickness is between 0.22 to 1.0 mm, with thinner elements yielding higher frequencies. Although a transducer should produce a frequency equal to its native operating frequency, in practice the ultrasound pulses produced can be composed of different frequencies. This range of frequencies is called bandwidth. Wide-bandwidth transducers give the operator the option of using the same transducer for different applications by selecting one of a range of available frequencies. If a higher frequency is chosen, better resolution can be achieved. However, if the penetration is insufficient, a lower frequency can be selected to obtain the desired penetration, although this will result in lower resolution (see Chapter 1). Each manufacturer provides a variety of transducers to be used for its ultrasound system for different applications (Figure 2-4). For pediatric and neonatal imaging, transducers with frequencies between 5 to 12 MHz are generally used, providing high-resolution imaging for neonates even less than 500g.
As mentioned earlier, the ultrasound unit ranges from a handheld device to a full-sized machine. In this section, we will describe the components that are most commonly shared by all these machines. Some of these components (Figure 2-5) are integrated into the machine without any access by the operator, while others are controlled by different knobs, buttons, or touch screens. The core components of the main unit are as follows:
Control panel
Pulse generator and beam former
Receiver
Memory
Recording device
Display
The control panel is the part of the system that coordinates the action and interaction among other components; it is unique to each machine. It includes a keyboard for entering information and a trackball to control cursors. Knobs and touch-screen icons (Figure 2-6) are used to access various modalities used in the ultrasound machines.
The pulse generator (pulser) produces electrical pulses which in turn are changed to ultrasound pulses by the transducer. The pulser’s job for a single-element transducer appears to be straightforward, but for phased array transducers, where sequencing and phasing are involved, it becomes much more complicated. In array transducers, switching, delays, and variation in pulse amplitudes (for multiple elements) are necessary for the electronic control of scanning, steering, transmission, and focusing of the beam of ultrasound pulses. These tasks are performed by the beam former.
The amplitude and intensity of the ultrasound pulse that is produced by the transducer is determined by the voltage amplitude produced by the pulser. The transducer output can be adjusted from the control panel by the sonographer, with the goal of achieving adequate ultrasound penetration while limiting the amount of ultrasonic energy that is directed at the patient. Transducer output is also called output gain, acoustic power, pulser power, energy output, and transmitter output. Output levels are often shown on the display in terms of percentage or decibel relative to maximum output. To the degree that output setting is increased, the amplitude of returning ultrasound pulses is enhanced. One should, however, be careful while using high power output, since the biological effects of ultrasound, such as peak negative acoustic pressure and intensity, could increase the likelihood of cavitation and tissue heating.
Echoes returning from the tissue are received by the transducer and produce electric voltages in the transducer that are sent through the beam former to the receiver for further processing. The functions of the receiver include (1) amplification, (2) compensation, (3) compression, (4) demodulation, and (5) reject.
Not all returning signals from the patient are suitable for further processing. Only frequencies around 100 kilohertz can be processed further, and these signals are often not strong enough. Therefore, a radiofrequency amplifier is used. Amplification is controlled by the operator and is also called receiver gain, or overall gain.
The echoes received from greater depth are more attenuated than those from shallower depth. However, they have lower amplitudes and do not appear as bright. The receiver will make special compensations to make all echoes arising from similar tissue appear with the same brightness. This process is also called:
Time gain compensation (TGC)
Depth gain compensation (DGC)
Swept gain compensation
TGC increases amplification of returning signals from increasing depths (Figure 2-7), in order to compensate for the progressive attenuation of the deeper echoes. The higher-frequency transducers usually need more compensation since they experience greater attenuation.
Some newer systems have lateral gain compensation (LGC, Figure 2-6). LGC amplifies the signals similar to TGC, but does so in the lateral aspect of the sector; hence, they become stronger and brighter. TGC and LGC are adjusted by the operator to optimize image quality. If compensation is properly used, all images of similar reflectors located at different depths will appear identical. Incorrect TGC settings affect only a portion of the image at a certain depth.
Ultrasound systems process a large range of the signals, with echo voltage amplitudes from smaller than 0.01 mV to greater than 1000 mV. Compression reduces the difference between the smallest and largest signals. This process is performed by special amplifiers called logarithmic amplifiers that do not alter the relationship between the signals. This means that weak inputs will be amplified more than strong ones, so that after compression (Figure 2-8) the largest signal is still the largest and the smallest signal is still the smallest. Dynamic range is the ratio of the largest to smallest amplitude that can be processed by an ultrasound system and is expressed in decibels. The dynamic range of an amplifier that is capable of processing between 0.01 mV to 1000 mV is 100 db. Although these amplifiers have a range of up to 160 dB, other electronic parts of the system might not have such a range. Typical displays have only about 20 dB of dynamic range, which means that the most powerful signal can only be 100 times larger than the smallest signal for display. Therefore, the compressor is generally used to change 50 dB, with an intensity ratio of 100,000, down to 20 db. This corresponds to an intensity ratio of 100, which is acceptable for the display.
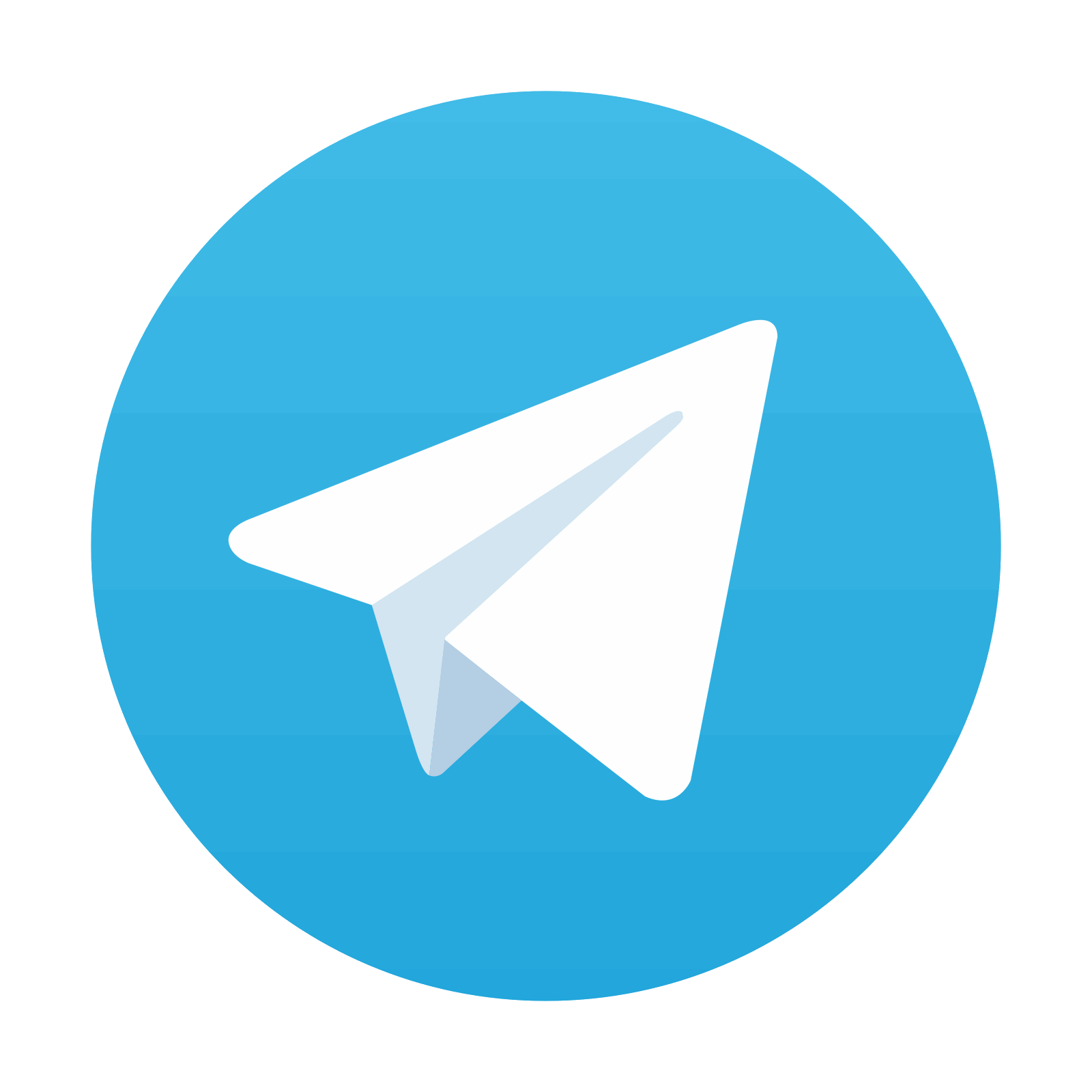
Stay updated, free articles. Join our Telegram channel

Full access? Get Clinical Tree
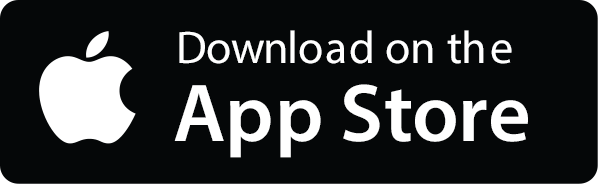
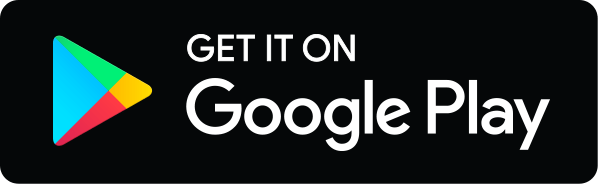