Pulmonary Physiologic Assessment of Operative Risk
Mark K. Ferguson
Complications occur frequently after major thoracic surgery. Their development is related to a variety of factors, including the type of operation performed, the approach to the operation, and the underlying condition of the patient. The occurrence of pulmonary complications is associated with prolonged hospitalization, a higher cost of hospital care, and an increase in operative mortality. Every effort should be made to reduce the incidence of such complications and to manage them expeditiously when they occur.
The ability to predict which patients are at higher risk for postoperative complications enables physicians and other caregivers to select appropriate patients for major surgery and to discuss relative risks with patients so that informed consent about a planned operation can be obtained. Risk stratification helps identify patients who might benefit from preoperative cardiopulmonary rehabilitation in an effort to reduce the incidence of complications and may direct patients to physicians or medical centers better able to manage higher-risk operations. Risk stratification also permits assignment of additional resources to the postoperative care of those patients deemed to be at increased risk for predictable complications.
The development of pulmonary complications—as well as the risk for operative mortality after pulmonary, esophageal, and upper abdominal operations—has been shown to be associated with the preoperative pulmonary status of the patient. Ensuring that an appropriate pulmonary physiologic assessment of patients has been performed before their operations is the responsibility of the treating surgeon. Historically, surgeons have been among the leaders in developing criteria for risk stratification based on pulmonary physiologic status. These efforts have led to algorithms that help treating physicians quantify the relative risk for complications based on a few important tests. However, numbers alone should not be used to make recommendations for individual patients; clinical judgment remains the most important factor in successful surgical care.
Effects of Surgery on Pulmonary Function
The type of operation and the incision used to perform an operation have varying deleterious effects on pulmonary function that have been well described with regard to the extent of functional decrement and the time course of recovery. Functional residual capacity (FRC) has been recognized for decades as the single most important lung volume measurement associated with the development of pulmonary complications after most types of operations. FRC is the lung volume that exists at the end of
normal expiration; its components are the expiratory reserve volume and the residual volume (Fig. 20-1). A number of factors are associated with a postoperative decrease in FRC, including general anesthesia, conditions that increase intra-abdominal pressure (such as obesity and ascites, the supine position), and, most important, the type and location of incisions used for an operation.
normal expiration; its components are the expiratory reserve volume and the residual volume (Fig. 20-1). A number of factors are associated with a postoperative decrease in FRC, including general anesthesia, conditions that increase intra-abdominal pressure (such as obesity and ascites, the supine position), and, most important, the type and location of incisions used for an operation.
Knowledge of the interaction of FRC with the closing volume (CV) of the lung is key in understanding postoperative pulmonary complications. The CV is the volume of the lung at which airflow from dependent parts of the lung stops during expiration owing to airway closure. Factors that promote an increase in CV include advancing age, tobacco use, fluid overload, bronchospasm, and the presence of airway secretions. Under normal circumstances, FRC is about 50% and CV is about 30% of total lung capacity. A reduction in FRC or an increase in CV results in premature airway closure and atelectasis.2,3 The resulting ventilation/perfusion mismatch causes hypoxia, and trapping of secretions contributes to pneumonitis, both of which may contribute to the development of respiratory insufficiency.
No consistent changes in FRC occur after operations that do not involve the abdomen or thorax. In contrast, FRC decreases by 10% to 15% after lower abdominal operations, upper abdominal operations reduce FRC by 30%, and thoracotomies result in a 35% decrease in FRC. The reduction of FRC after abdominal surgery is attributed to both dysfunction of abdominal wall musculature and impaired diaphragmatic function. Open upper abdominal incisions cause a substantial decrease in the function of the diaphragm and lungs.66 Open operations cause greater perturbations in lung function than do laparoscopic operations,43,87,135 although adverse effects after laparoscopic operations are nevertheless substantial.158 Maximum diaphragmatic pressure decreases after laparoscopic upper abdominal procedures by >50% during the first 6 hours after surgery.58,140 Partial recovery is evident 24 hours after surgery. Laparoscopic lower abdominal procedures cause minimal reductions in diaphragmatic activity and lung function compared with those evident after upper abdominal laparoscopy, demonstrating that even minimally invasive procedures cause diaphragmatic and ventilatory dysfunction that is dependent on the location of the operation.85,140
Postoperative pain does not appear to be an adequate explanation for these changes, which develop despite adequate postoperative analgesia.141 Diaphragmatic contractility is not altered after upper abdominal operations. Instead, it has been suggested that reflexes inhibit phrenic nerve output.54 Extradural block has been shown to improve postoperative diaphragmatic function after upper abdominal surgery, supporting the contention that interruption of afferents that inhibit diaphragm activity may result in improved outcomes.100,125
The performance of a sternotomy has deleterious effects on chest wall mechanics and postoperative pulmonary function similar to those resulting from laparotomy, possibly leading to pulmonary complications.42 A restrictive pattern develops in the early postoperative period that is manifested by a decrease in FRC and impaired inspiratory and expiratory pressures.147 Respiratory pressures return to normal 6 weeks postoperatively, but FRC and other ventilatory parameters remain decreased. A restrictive ventilatory defect appears in the early postoperative period after median sternotomy owing to reduced and uncoordinated rib cage expansion; this is no longer evident 3 months postoperatively.98 Harvesting an internal mammary artery graft for coronary revascularization impairs chest wall mechanics more than when saphenous vein grafts are used.18 Overall, reports suggest that structural alterations in chest wall mechanics and decreased blood flow to intercostal muscles are responsible for restrictive ventilatory changes after sternotomy that may be related to the development of pulmonary complications.
Thoracotomy is the operative approach associated with the highest potential risk for postoperative pulmonary complications, owing to restricted chest wall motion, impaired diaphragm activity, and possible loss of pulmonary parenchyma. The development of diaphragmatic dysfunction after thoracotomy resembles the dysfunction that accompanies upper abdominal operations.68 Epidural analgesia does not reverse diaphragmatic dysfunction after thoracotomy, a situation analogous to upper abdominal surgery.68 A thoracotomy incision transiently reduces most ventilatory parameters postoperatively, with a precipitous drop occurring by the first postoperative day (Fig. 20-2). At the end of the second postoperative week, some recovery occurs, but the deleterious effects do not resolve for almost 3 months after surgery. Thoracoscopic operations cause a somewhat smaller decrement in pulmonary function during the immediate postoperative period.69,113,116 The advantage persists for the first postoperative week, after which both open thoracotomy and thoracoscopic approaches to major lung resection appear to have similar outcomes. A multitude of authors point out that major lung resection permanently reduces spirometric values and diffusing capacity by varying degrees, depending on the amount of lung that is resected. When measured 6 months to 1 year postoperatively, segmental or wedge resections reduce lung function
by <10%, lobectomy or bilobectomy results in decreases of 5% to 15%, and pneumonectomy reduces values by 20% to 40% (Fig. 20-3).5,15,22,28,67,111,118,120,121,128,134,145,148,157,163
by <10%, lobectomy or bilobectomy results in decreases of 5% to 15%, and pneumonectomy reduces values by 20% to 40% (Fig. 20-3).5,15,22,28,67,111,118,120,121,128,134,145,148,157,163
Predictive Factors for Pulmonary Morbidity and Mortality after Major Lung Resection
Many useful data have been generated regarding factors associated with postoperative morbidity for lobectomy, bilobectomy, and pneumonectomy (Table 20-1). As a result, a variety of algorithms have been suggested for the preoperative assessment of such patients.
Lung Volume Measurements
The measurement of lung volumes, including total lung capacity (TLC) and residual volume (RV), is useful in the evaluation of the lung resection candidate. Vital capacity is decreased when TLC is reduced by restrictive processes, when RV is increased owing to air trapping in obstructive disease, or by a combination of these factors (Fig. 20-4). Recognition of which elements contribute to a decreased vital capacity may help in assessing the preoperative patient. It is not possible to make this distinction without measurement of RV, which cannot be obtained by routine spirometry but requires helium equilibration, nitrogen washout, or body plethysmography techniques to determine.
![]() Figure 20-3. Permanent changes in forced vital capacity (FVC), forced expiratory volume in 1 second (FEV1), and diffusing capacity of the lung for carbon monoxide (DLCO) after various types of lung resection. (Data from references 5, 15, 22, 28, 67, 111, 118, 120, 121, 128, 134, 145, 148, 157, and 163.) |
Restrictive physiology causes reductions in vital capacity as well as TLC, RV, and FRC. It is caused by pulmonary fibrosis, sarcoidosis, muscular weakness and disease, chest wall deformities, large acquired diaphragmatic hernias, and trapped lung owing to extensive pleural fibrosis. In the case of a trapped lung, surgery that includes a pulmonary decortication may help resolve the restrictive physiology, resulting in improved pulmonary dynamics postoperatively. Similar effects are produced by surgical correction of a large diaphragmatic hernia. None of the other conditions that cause a restrictive physiology are improved by thoracic surgery, highlighting the possible risk to these patients of major thoracic surgical procedures.
Obstructive physiology causes reductions in vital capacity, but there are associated increases in TLC, RV, and FRC. It is usually caused by emphysema, chronic bronchitis, and asthma. The use of bronchodilators and, in severe cases, inhaled or systemic steroids can dramatically improve obstructive physiology in patients with reactive airways disease, making thoracic surgery
much safer. In highly selected patients with heterogeneous emphysema, resection of the most affected regions of the lung can result in an increase in VC. In most other instances, the abnormal measurements are relatively fixed, and major lung surgery further reduces vital capacity.
much safer. In highly selected patients with heterogeneous emphysema, resection of the most affected regions of the lung can result in an increase in VC. In most other instances, the abnormal measurements are relatively fixed, and major lung surgery further reduces vital capacity.
Table 20-1 Tests of Cardiopulmonary Physiologic Status and the Factors They Assess | ||||||||||||||||||||||||||||||||||||||||||||||||||||||||||||||||
---|---|---|---|---|---|---|---|---|---|---|---|---|---|---|---|---|---|---|---|---|---|---|---|---|---|---|---|---|---|---|---|---|---|---|---|---|---|---|---|---|---|---|---|---|---|---|---|---|---|---|---|---|---|---|---|---|---|---|---|---|---|---|---|---|
|
![]() Figure 20-4. Relative changes in lung volumes associated with lung diseases. See Figure 20-1 for definitions. |
Spirometry
The relationship between vital capacity and mortality in the general population was identified in the mid-1800s. By the middle of the twentieth century, a variety of lung volume measurements were being used in the physiologic assessment of candidates for lung resection. The utility of timed volume measurements in assessing pulmonary sufficiency for surgery was first described in 1951.70 This work demonstrated that timed measurements—such as maximum breathing capacity (now known as maximum voluntary ventilation, or MVV) and the forced expiratory volume in 1 second (FEV1)—were more sensitive in assessing pulmonary sufficiency than was vital capacity. The report showed that reductions in vital capacity correlated with restrictive ventilatory defects, whereas decreases in MVV and FEV1 correlated with obstructive ventilatory abnormalities. Operative mortality and long-term postoperative respiratory insufficiency generally were subsequently shown to be related to VC, with mortality occurring primarily in patients with VC <60% to 70% of predicted.71 Mortality was found to be more clearly related to abnormalities of timed measurements such as MVV and FEV1.
FEV1 was first identified in 1973 as the best predictor of which patients would not tolerate major lung resection; this report suggested that an FEV1 of <2.0 L put patients into a high-risk group, particularly patients >60 years of age.26 Others subsequently described patients at increased risk as having an FEV1 of <1.64 L and those at very high risk for major lung resection as having an FEV1 of <1.20 L.99 The general guidelines of FEV1 of <2.0 L and MVV <50% of predicted as indicative of a potential increased risk for major lung surgery were confirmed in a 1975 report suggesting that additional physiologic studies be obtained in patients at increased risk.124 During the 1960s and 1970s, the use of timed measurements of expiratory volumes thus became standard in the evaluation of candidates for lung resection.
Predicted Postoperative Function
The utility of predicted postoperative function as a determinant of operability was recognized in the 1940s and 1950s. The original techniques used for calculating predicted postoperative function included bronchospirometry, unilateral pulmonary artery occlusion, and the lateral position test. All of these tests are now of historic interest because of the introduction of lung scintigraphy. Pulmonary scintigraphy was initially developed in the 1950s to study the regional distribution of lung ventilation using a number of radiolabeled gases. Similarities between differential lung function measurements made by broncho- spirometry and radiospirometry were described in 1968, which suggested that use of quantitative pulmonary scintigraphy in patients determined to be at increased risk for major lung resection on the basis of a decreased MVV should be routine.109 The utility of lung scintigraphy in estimating postoperative function was first reported in 1972.91 The technique was found to be particularly useful for patients undergoing pneumonectomy.4,124 The percentage of function attributed to the lung not being resected was multiplied by the preoperative measured value of lung function to achieve a predicted postoperative value for lung function (Fig. 20-5). A technique was subsequently developed for using lung scintigraphy to estimate postoperative function in patients undergoing lobectomy: the expected loss of function was calculated by multiplying the preoperative FEV1 by the percentage of function in the affected lung and by the percentage of segments of that lung that were to be resected.5
The superiority of perfusion over either ventilation alone or a combination of ventilation and perfusion for estimating postoperative lung function was demonstrated in 1980.156 The percentage of perfusion to the lung not being resected was multiplied by the preoperative spirometric value to estimate the resultant postoperative value. The correlation between calculated and actual measurements of postoperative lung function was high, and the error rate was <10% (Fig. 20-6). This accuracy has subsequently been confirmed by many other authors.108 It was subsequently suggested that patients who are candidates for pneumonectomy but who have a preoperative FEV1 of <2.0 L should undergo quantitative radionuclide lung perfusion scanning to assess relative distribution of function between the two lungs.133
Pulmonary scintigraphy as a means for estimating postoperative pulmonary function yielded specific cutoff values for
operability that differed among investigators. Various authors suggested that a postoperative predicted FEV1 of <800 or 1,000 mL precludes resection.91,124 Some recommendations were based on the incidence of hypercapnia in unoperated patients with an FEV1 of <800 mL.137 Despite the lack of conclusive evidence supporting this guideline, a postoperative predicted FEV1 of between 800 mL and 1 L is still used by many surgeons as a cutoff for distinguishing between normal- and high-risk candidates for major lung resection.
operability that differed among investigators. Various authors suggested that a postoperative predicted FEV1 of <800 or 1,000 mL precludes resection.91,124 Some recommendations were based on the incidence of hypercapnia in unoperated patients with an FEV1 of <800 mL.137 Despite the lack of conclusive evidence supporting this guideline, a postoperative predicted FEV1 of between 800 mL and 1 L is still used by many surgeons as a cutoff for distinguishing between normal- and high-risk candidates for major lung resection.
![]() Figure 20-5. Quantitative perfusion scan (anterior view) of a patient with a proximal right-upper-lobe lung cancer. Distribution of radiotracer is indicated according to upper and lower lung zones. |
The calculation of postoperative lung function using simple equations rather than physiologic tests was originally introduced in 1975:86 an equal value was assigned to each of the 19 lung segments in order to determine the amount of functioning lung remaining after resection. The preoperative spirometric value was multiplied by the percentage of lung segments remaining after resection, resulting in a predicted postoperative value. Despite the fact that all segments were used in the calculation of predicted postoperative values for FEV1 regardless of their functional status, the correlation between the predicted and actual postoperative values for FEV1 was high, and the error range was only 7%.156 Using this technique, an increase in postoperative morbidity was demonstrated in patients with a predicted postoperative FEV1 of <900 to 1,000 mL.88,130 A number of studies have confirmed the good correlation between predicted postoperative and measured postoperative FEV1 and FVC in patients undergoing major lung resection.133,157,159,163 However, postoperative predicted values after resection can underestimate or overestimate FEV1 by an average of 250 mL. Part of the explanation for the discrepancy between predicted and measured values in some studies might have been the inclusion of nonfunctioning segments in the estimation of postoperative function.164
More recent techniques for calculating predicted postoperative spirometric values use the number of functioning segments as the denominator and the number of functioning segments to be resected as the numerator for purposes of calculating predicted postoperative values.55 In this way, segments that are nonfunctional (owing to obstruction by a proximal cancer or consolidation or destruction due to an inflammatory process) are not included in the following calculation:

This calculation technique is superior to the use of all lung segments regardless of functional status.23
The use of quantitative computed tomography (CT) in estimating relative lung function as a means for calculating predicted postoperative function has been shown to be similar to lung perfusion scintigraphy and segmental percentage loss in the accuracy of predicting postoperative function.23,97,161 In general, quantitative CT or scintigraphic assessment of perfusion is similar to anatomic techniques for estimating postoperative function after segmentectomy or lobectomy, whereas anatomic techniques are not as accurate for estimating postoperative function after pneumonectomy.
Spirometry Expressed as a Percentage of Predicted Normal Values
Despite the apparent utility of preoperative and predicted postoperative FVC and FEV1 in assessing a patient’s risk for major lung resection, these absolute rather than relative values did not allow for the known variation of lung function related to sex, age, and height. Normal spirometric values based on age, sex, and body height were published as early as 1948,10 and reference values currently are readily available.77 The problem of using absolute rather than relative criteria for predicting risk is easily illustrated: a postoperative FEV1 of 800 mL represents 48% of the predicted normal for a short, elderly woman but only 21% of the predicted normal for a tall, middle-aged man.72 A significant increase in pulmonary complications was first reported to occur among patients with a preoperative FEV1 of
<67% of predicted.89 Subsequent work suggested that a preoperative FEV1 of <60% of predicted was associated with a 2.5-fold increase in the incidence of pulmonary complications after lung resection50 and that a preoperative FEV1 of <70% of predicted also was associated with a significant increase in the risk for pulmonary complications.138
<67% of predicted.89 Subsequent work suggested that a preoperative FEV1 of <60% of predicted was associated with a 2.5-fold increase in the incidence of pulmonary complications after lung resection50 and that a preoperative FEV1 of <70% of predicted also was associated with a significant increase in the risk for pulmonary complications.138
The concepts of spirometry expressed as a percentage of predicted and calculation of predicted postoperative spirometric values were subsequently combined, yielding a calculated postoperative value of 30% of predicted as a potentially useful guideline for identifying patients at high risk for major lung resection.72 This was similar to the values of 33% and 30% identified by others as suitable limits6,115 for determining excess risk based on retrospective clinical studies. Other authors suggested a postoperative predicted value for FEV1 of 40% of normal as a means for selecting patients who are at high risk for lung resection.126,136 A 50% mortality rate was described among patients with a postoperative predicted FEV1 of <40% of normal after lung resection, whereas patients with a postoperative predicted FEV1 of >40% suffered no operative mortality.101 Others described a fourfold increase in operative mortality using a postoperative predicted FEV1 of <40% of normal to separate risk groups.129 More leeway is perhaps appropriate for patients undergoing pneumonectomy, for whom a predicted postoperative FEV1 of <34% of normal is predictive of increased operative mortality.130
Care must be taken in estimating postoperative spirometric values as a means for selecting patients for major lung resection, especially at the lower limits of lung function. Patients with more severe obstructive lung disease—as defined by worse FEV1, lower FEV1/FVC ratio, or lower diffusing capacity—experience less percentage loss of lung function as measured by spirometry than do patients with normal lung function9,36,139,150 (Fig. 20-7). A substantial proportion of these patients, likely those with heterogeneous disease who undergo resection of the most diseased portions of their lungs, experience improvement in measured lung function and respiratory symptoms postoperatively. The use of absolute limits for determining which patients should be excluded from consideration for major lung resection should be tempered by the relative inaccuracy of predicting postoperative function in higher-risk patients.
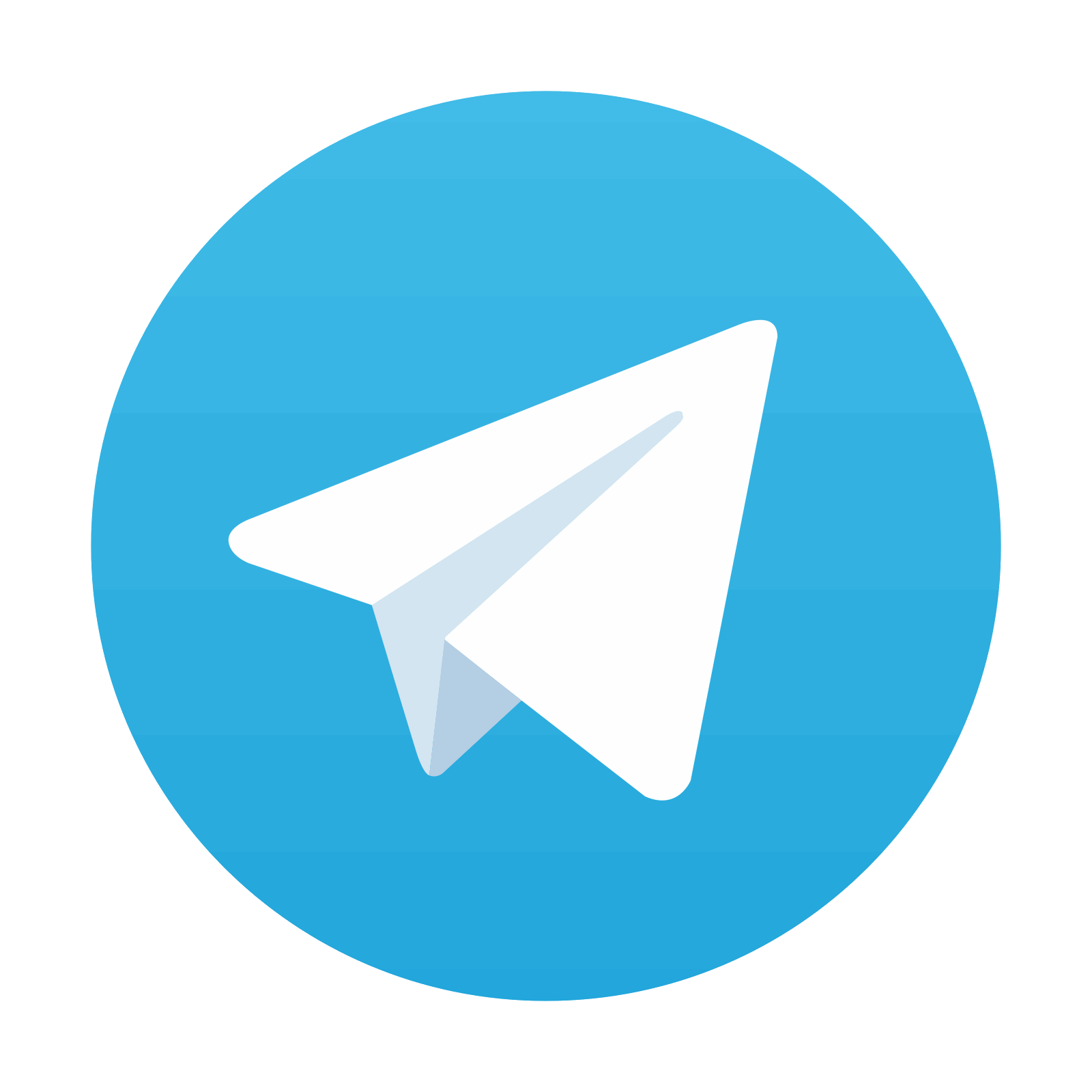
Stay updated, free articles. Join our Telegram channel

Full access? Get Clinical Tree
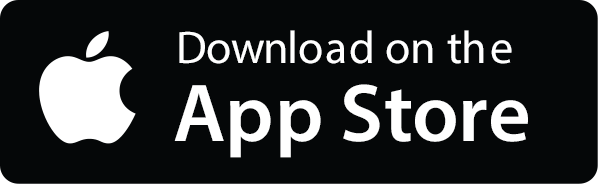
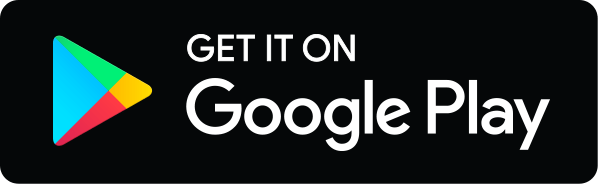