CHAPTER 120 Pulmonary Atresia with Ventricular Septal Defect and Right Ventricle–to–Pulmonary Artery Conduits
PULMONARY ATRESIA WITH VENTRICULAR SEPTAL DEFECT
Pulmonary atresia with ventricular septal defect (PAVSD) is a congenital cardiac malformation characterized by discontinuity of blood flow from the right ventricle to the pulmonary arteries, a ventricular septal defect (VSD) resulting from anterior deviation of the infundibular (conal) septum, and an overriding aorta. Because it shares many attributes of tetralogy of Fallot (TOF), it is also referred to as TOF with pulmonary atresia. The incidence of PAVSD is estimated to be 1/10,000 live births.1 A right aortic arch may be seen in up to 45% of patients.2 PAVSD is also associated with major aortopulmonary collaterals (MAPCAs) that, in some cases, are the sole supply of pulmonary blood flow. The morphology of the pulmonary circulation, which varies significantly among patients, determines the management and prognosis of this malformation. PAVSD may also be associated with other intracardiac defects such as tricuspid atresia or stenosis, complete atrioventricular (AV) canal, complete or corrected transposition of the great arteries, left superior vena cava, anomalies of the coronary sinus, dextrocardia, and asplenia or polysplenia syndrome. These more complex forms of PAVSD are not discussed in this chapter. The most common associated genetic defect is a 22q11 microdeletion, found in up to 34% of patients with PAVSD, and up to 65% of patients with MAPCAs are found to have this abnormality.3 Other phenotypic abnormalities with the 22q11 microdeletion include submucosal cleft palate, abnormal facies, delayed development, and mental retardation. Neonates with trisomy 13 or 18 may have PAVSD as well, and the prognosis is extremely poor for these children.
Anatomy and Pathophysiology
Like TOF, PAVSD is associated with anterior deviation of the infundibular septum, a conoventricular VSD, and the resulting aortic override (Fig. 120-1). The spectrum of pulmonary atresia varies from purely valvular or subvalvular atresia (with intact main and branch pulmonary arteries) to complete absence of central pulmonary arteries. Pulmonary blood flow depends on the presence of an aortopulmonary communication, which may exist in the form of a patent ductus arteriosus (PDA), other major nonductal collaterals arising from the descending aorta that connect to the central native pulmonary arteries, or MAPCAs that directly enter the hilum and join the segmental pulmonary arteries. In utero, the pulmonary parenchyma is perfused by branches from the dorsal aorta as well as by the sixth aortic arch, which forms the basis for the central pulmonary arteries. Abnormal development of the central pulmonary arteries leads to persistence of the dorsal aortic branches, which ultimately develop into MAPCAs.
The lungs may be supplied by the native pulmonary arteries, by MAPCAs, or by both. If confluent central pulmonary arteries are present, the blood supply to the lung may arise from a PDA, a central “ductlike” collateral, or a MAPCA. The behaviors of these three sources of pulmonary blood flow differ in their predisposition to constrict over time, which has implications for the stability of pulmonary blood flow. Whereas MAPCAs or ductlike collaterals might remain patent beyond the neonatal period, the PDA is likely to constrict postnatally as a result of the presence of prostaglandin-responsive ductal tissue. Although the distinction may be difficult to establish by preoperative imaging studies, the location on the aorta from which the collateral originates is suggestive. A solitary collateral that arises just distal to the left subclavian artery (off the left aortic arch) and travels to a central pulmonary confluence is likely to be a PDA, whereas a collateral that arises from any other location off the aorta and supplies a central confluence is termed a ductlike collateral. A vessel that exits the aorta and travels directly to the pulmonary parenchyma is likely to be a MAPCA. There may be heterogeneity in the sources of pulmonary blood flow in a given patient, so one segment of lung may be supplied by the native pulmonary artery but another segment by a MAPCA. Some segments may have a dual supply (Fig. 120-2). Patients with confluent central pulmonary arteries that are supplied by a PDA are less likely to have significant MAPCAs, and those with nonconfluent central pulmonary arteries depend on MAPCAs for pulmonary blood flow.
Classification
There is no standard classification system for PAVSD, but several have been proposed. Most classification schemes focus on the patterns of pulmonary blood flow. The classification system adopted by the Congenital Heart Surgery Nomenclature Project broadly divides pulmonary artery anatomy into the following categories: central pulmonary arteries without MAPCAs; a dual flow, with confluent central pulmonary arteries and MAPCAs; and MAPCAs, with nonconfluent or absent central pulmonary arteries.4
Another classification scheme divides patients further according to the specific patterns of pulmonary artery anatomy that are most commonly encountered (Fig. 120-3):
Natural History
The natural history of PAVSD varies significantly depending on the pulmonary artery morphology. Patients presenting with PAVSD that has ductal-dependent pulmonary blood flow experience profound fatal cyanosis in the neonatal period as the ductus begins to close. On the other hand, patients with MAPCAs with mild stenosis of collateral vessels and balanced pulmonary blood flow may exhibit only mild cyanosis. These patients may survive into adolescence or adulthood without repair, although their life expectancy is significantly shorter than that of the normal population. Most patients fall between these two extremes. Patients with aortopulmonary collaterals may exhibit balanced pulmonary circulation for a period of time; however, development of ostial stenosis in the collateral leads to progressive cyanosis. Alternatively, excessive pulmonary blood flow from an unrestrictive collateral results in congestive heart failure. The presence of long-standing unrestrictive blood flow in a MAPCA may result in irreversible elevation of pulmonary vascular resistance (PVR) in that segment. Pulmonary hypertension in a segment of the lung is seen commonly in patients who present at a later age. Heterogeneity in PVR in a lung segment may result in mismatch. Without timely intervention, the overall mortality is approximately 60% by 30 years of age, with most of the mortality occurring within the first year of life.1,5
Diagnostic Studies
Cardiac Catheterization
Cardiac catheterization is preferred for patients with PAVSD who have nonconfluent or hypoplastic central pulmonary arteries. These patients have the highest likelihood of having MAPCAs, which are best delineated by catheterization (Fig. 120-4). The goal of catheterization is to assist with surgical planning by evaluating the anatomy, size, degree of stenosis, and the presence of dual supply from native pulmonary arteries or collaterals. Vascular supply to all segments must be clearly determined. Native pulmonary arteries may require visualization by pulmonary venous wedge injection if there are no collaterals to the native pulmonary arteries. In older children and adults, measurements of individual pulmonary artery pressures are obtained to determine the risk of development of pulmonary hypertension in high-pressure collaterals. The pulmonary-to-systemic blood flow ratio (Qp/Qs) can be estimated by the Fick method if all pulmonary flow arises from collaterals (i.e., there is no previous right ventricle–to–pulmonary artery [RV-to-PA] conduit). Interventions that may be performed at catheterization include balloon dilation of native pulmonary vessels, stenting of highly restrictive MAPCAs, or coiling of redundant dual-supply collaterals.
Magnetic Resonance Imaging
Magnetic resonance imaging (MRI) is a useful adjunct to cardiac catheterization in patients with MAPCAs. The advantage of MRI is its ability to image native pulmonary arteries that may not be accessible by cardiac catheterization. It is superior to other imaging modalities at delineating the relationship of MAPCAs to other mediastinal structures (airway, esophagus).6 MRI is used to estimate RV volumes and the conduit regurgitation fraction in completely repaired patients, and it can be used to guide decision making regarding conduit replacement.
Radionuclide Lung Perfusion Scanning
In patients with stenosis of native pulmonary arteries after unifocalization, decreased blood flow to specific segments of lung may result in cyanosis and increased right ventricular afterload. A useful screening tool to detect segmental malperfusion is lung perfusion scanning. It can be used to screen patients for the presence of significant perfusion defects that may indicate stenosis of the native pulmonary arteries or MAPCAs.7
Treatment
Surgical Considerations
Unifocalization
Unifocalization is a technique in which MAPCAs are disconnected from the descending aorta and anastomosed to a common confluence that can receive blood supply from a stable source—usually the RV or a systemic shunt. Unifocalization facilitates eventual establishment of RV-to-PA continuity by providing a centrally located confluence to which a conduit may be sewn. Ideally, this confluence provides low resistance to right ventricular output and supplies all segments of the lung. To perform a successful unifocalization requires careful review of preoperative studies (catheterization and MRI) to delineate the anatomy and size of vessels, and a dual supply by MAPCAs and native pulmonary arteries. If central native pulmonary arteries are present, collaterals may be anastomosed to them. Unifocalization procedures may be performed via either a sternotomy or a thoracotomy approach. Unifocalized vessels may be supplied by a shunt from the systemic circulation or by a right ventricular conduit. If high vascular resistance is found by catheterization in segments supplied by an unrestrictive MAPCA (common in the older child or adult), unifocalization to a systemic shunt (rather than to the RV-to-PA conduit) may be preferable to provide the higher perfusion pressures capable of maintaining adequate blood flow and oxygen saturations.
Closure of Ventricular Septal Defect
Preoperative imaging and tools are available to help guide management of the VSD. On the basis of angiography and MRI, a surgeon must determine if the number and caliber of existing pulmonary vascular segments are sufficient to support a full cardiac output. Recruitment of pulmonary vasculature supplying greater than 12 lung segments is a prerequisite for attaining low pulmonary resistance. Several indices of pulmonary artery caliber have been used to predict postoperative right ventricular pressures and successful VSD closure. The McGoon index is the ratio of the sum of the diameters of the proximal extrapericardial right and left pulmonary arteries to the diameter of the descending aorta at the level of the diaphragm. A McGoon index of 1 predicts an RV/LV pressure ratio of 0.7. The Nakata index is the normalized sum of the cross-sectional areas of the right and left pulmonary arteries. The Birmingham formula estimates the RV/LV pressure ratio as 0.484 × (RPA/Ao + LPA/Ao) + 0.2007, where RPA is the diameter of right pulmonary artery at the hilum, Ao is the diameter of the descending aorta, and LPA is the diameter of the left pulmonary artery at the hilum. Such indices have been variably successful in guiding intraoperative management, but they are useful tools to assist the surgeon with preoperative planning. A tool to assist with intraoperative decision making regarding VSD closure is functional assessment of vascular resistance after single-stage unifocalization. Reddy and Hanley have championed determination of static vascular resistance by measuring the pressure generated by perfusing the unifocalized PAs with constant flow perfusate, and this method has been shown to predict postoperative right ventricular pressures.8
Transanular Patch
In patients with pulmonary valvular atresia, the presence of an imperforate membrane at the valve level is the primary abnormality. Infundibular muscular stenosis may also be present, and the main pulmonary artery is variably hypoplastic. This anatomy is amenable to augmentation of the RVOT with a transanular patch that extends from the infundibulum to the bifurcation of the main pulmonary artery. This approach reduces the need for RVOT reoperation, but it creates pulmonary regurgitation. Use of a monocusp transannular patch may prevent early pulmonary regurgitation with this approach.9,10
Maintenance of Patent Foramen Ovale
Generally, following neonatal repair of PAVSD, a low compliance RV leads to elevated right atrial pressures. Postoperative right ventricular dysfunction and hypertension may further elevate the right atrial pressures. Without an atrial septal defect or a patent foramen ovale (PFO), elevated right atrial pressures lead to hepatic congestion, anasarca, and low cardiac output. A small atrial-level defect (between 3 and 4 mm) allows right-to-left shunting when right ventricular dysfunction, hypertension, or poor compliance is expected. In this setting, the right-to-left shunting allows maintenance of cardiac output at the expense of mild desaturation, which is well tolerated in neonates and infants.
Systemic-to-Pulmonary Artery Shunt
Systemic-to-pulmonary artery shunts may be employed under certain circumstances. Some centers advocate this as the initial palliative procedure in all patients with TOF and PAVSD with confluent central pulmonary arteries. As a part of this procedure, the PDA or other major collateral may be ligated or left open. The advantages of this approach when compared with complete repair include avoidance of the upfront mortality of a neonatal repair while promoting growth of the pulmonary arteries.11 The major disadvantages include distortion of the pulmonary arteries and the risk of shunt thrombosis and fatal hypoxic event until a stable source of pulmonary blood flow is established.12,13
Transplantation
Lung or heart–lung transplantation may be applied selectively for certain patients, generally adolescents or adults, with irreversible pulmonary hypertension. For patients with normal ventricular function, lung transplantation, with or without cardiac repair (VSD closure or conduit change), may be performed. Heart–lung transplantation must be considered for patients with myocardial dysfunction or complex intracardiac defects.14
Cardiopulmonary Bypass Strategy
Cardiopulmonary bypass is required for procedures involving VSD closure or RV-to-PA conduit placement. Thus, single-stage unifocalization with an RV-to-PA conduit via a sternotomy approach requires cardiopulmonary bypass, whereas unifocalization to a systemic shunt through a thoracotomy does not. The bypass strategy must be tailored to patients with MAPCAs, because runoff into the pulmonary circulation may result in systemic hypoperfusion as well as poor visualization during intracardiac repair or pulmonary artery reconstruction as a result of flooding of the field with collateral blood flow. Control of all accessible collaterals after initiation of bypass is recommended, and higher flows (cardiac index of up to 4.0 L/min/m2, if necessary) are used to maintain systemic perfusion. Hypothermia reduces metabolic demand and provides protection of end organs when there is reduced systemic perfusion. It allows the surgeon to reduce bypass flows or enter brief periods of circulatory arrest to improve visualization of the surgical field during critical portions of the operation. When appropriate, maintenance of cardiac contractions and pulsatile flow (by not completely emptying the heart) during bypass may provide superior systemic perfusion in the presence of collateral runoff. Cardioplegic arrest is required for repair of VSD, but it may also be used during placement of the RV-to-PA conduit.
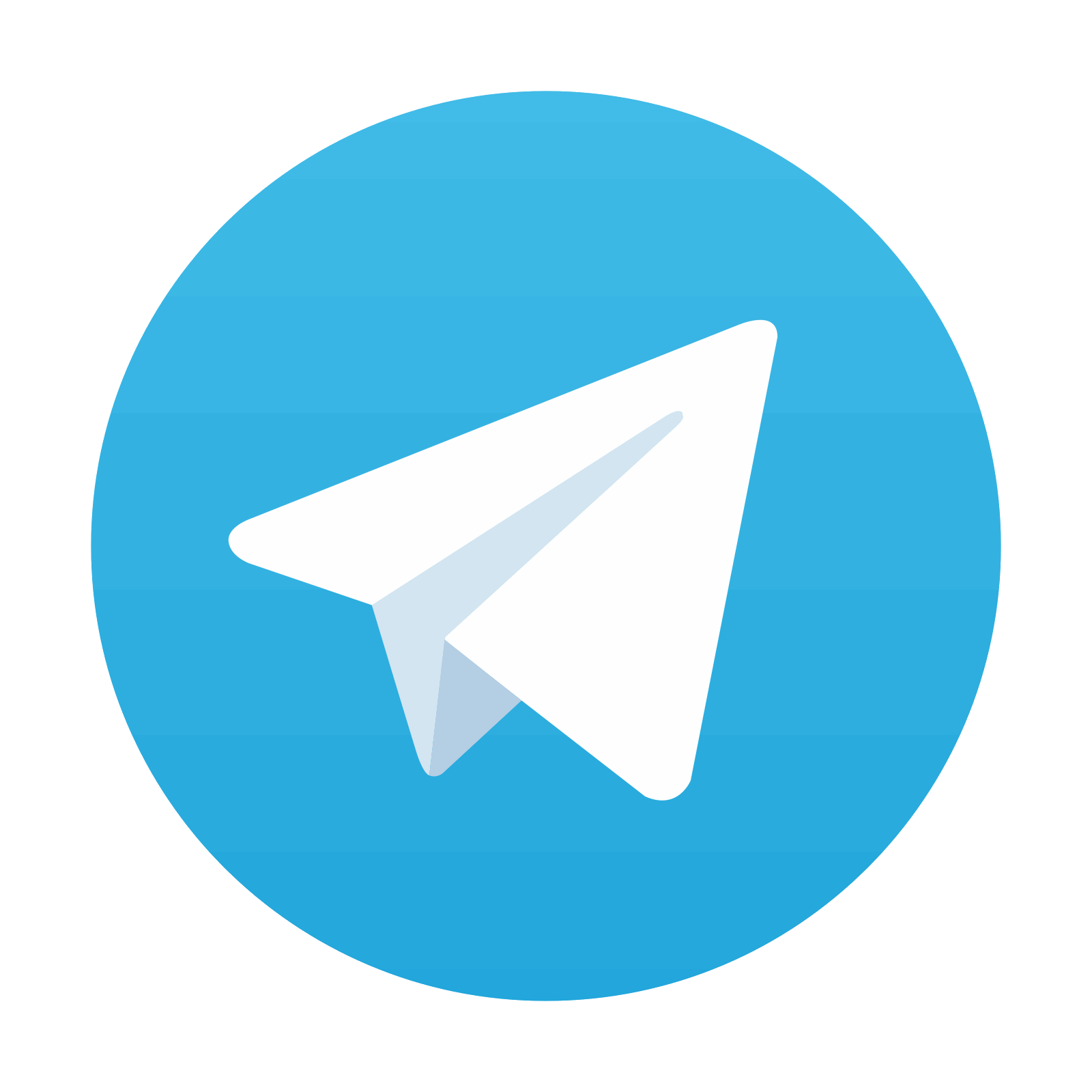
Stay updated, free articles. Join our Telegram channel

Full access? Get Clinical Tree
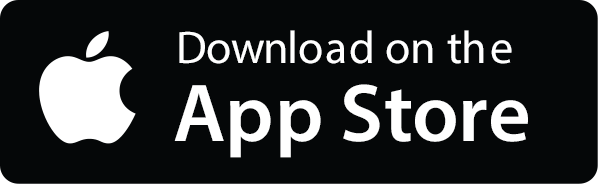
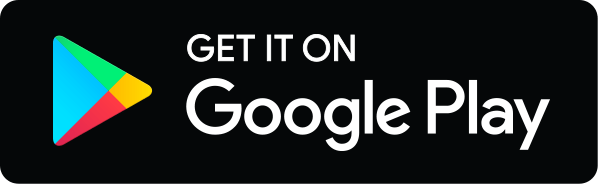