Objectives
- 1.
Explain the nine types of acid–base conditions.
- 2.
Distinguish between acute and chronic acid–base conditions and compensatory mechanisms.
- 3.
Explain respiratory compensatory mechanisms.
- 4.
Explain renal compensatory mechanisms.
- 5.
Describe use of base excess and anion gap in understanding acid–base status.
- 6.
Develop an approach to the interpretation of arterial blood gases.
This chapter describes the clinical interpretation of Po 2 and Pco 2 levels in arterial blood. The partial pressures of O 2 , CO 2 , and pH in the blood can be measured readily. Their values show the net effect of disease on gas exchange, and thus in the assessment of lung disease the arterial blood gas measurement is the best overall test of lung function. Arterial blood gas values cannot be determined by clinical assessment alone, even by experienced clinicians. For example, cyanosis does not become apparent until the arterial oxygen falls to less than 50 torr or 80% saturation, and quality of air exchange determined by auscultation is a poor assessment of alveolar ventilation. Thus arterial blood gas measurements are an important and essential tool in caring for critically ill patients, guiding therapy, and monitoring the progression of chronic lung disease.
Principles of Interpreting Arterial Blood Gas Values
Just as other laboratory tests such as chest radiographs benefit from a careful, systematic approach, so does the interpretation of arterial blood gas values. Arterial blood gases should always be interpreted in light of the patient’s history and symptoms. Recall the Irish proverb: Normal values are normal in normal people. For example, if a patient’s respiratory rate is three times normal and the measured arterial Pa co 2 is 40 mm Hg (“normal”), it does not necessarily mean that this patient has normal lungs. Rather, this patient is maintaining normal alveolar ventilation (i.e., a normal Paco 2 ) by markedly increasing his or her respiratory rate. As we shall see, a number of mechanisms (such as an increase in minute ventilation) can compensate for abnormalities in gas exchange and can bring the body back toward homeostasis. Normal values for arterial blood gas measurements are shown in Table 9.1 .
pH Normal | 7.35–7.45 |
Acidosis | |
Mild | 7.30–7.35 |
Moderate | 7.25–7.30 |
Severe | <7.25 |
Alkalosis | |
Mild | 7.45–7.50 |
Moderate | 7.50–7.55 |
Severe | >7.55 |
Pao 2 Normal | >85 mm Hg |
Hypoxemia | |
Mild | 55–85 mm Hg |
Moderate | 40–55 mm Hg |
Severe | <40 mm Hg |
Pa co 2 Normal | 35–45 mm Hg |
Hypercapnia | |
Mild | 45–50 mm Hg |
Moderate | 50–60 mm Hg |
Severe | >60 mm Hg |
Hypocapnia | |
Mild | 30–45 mm Hg |
Moderate | 25–30 mm Hg |
Severe | <25 mm Hg |
HCO 3 – Normal | 22–28 mEq/L |
Depression | |
Mild | 19–22 mEq/L |
Moderate | 17–19 mEq/L |
Severe | <17 mEq/L |
Elevation | |
Mild | 28–31 mEq/L |
Moderate | 31–35 mEq/L |
Severe | >35 mEq/L |
Base Excess Normal | –3 to +4 mEq/L |
Depression | |
Mild | –4 to –7 mEq/L |
Moderate | –7 to –10 mEq/L |
Severe | > –10 mEq/L |
Elevation | |
Mild | +4 to +8 mEq/L |
Moderate | +8 to +12 mEq/L |
Severe | > +12 mEq/L |
Anion Gap | 5–11 mEq/L |
AaD o 2 † | 10–25 mm Hg (on room air) |
∗ For a 25-year-old adult at sea level on room air. Normal values can vary slightly in different laboratories.
† The alveolar–arterial oxygen difference (AaD o 2 ) widens with age.
Types of Acid–Base Abnormalities
There are nine fundamental acid–base conditions: normal, acute, and chronic respiratory acidosis; acute and chronic respiratory alkalosis; acute and chronic metabolic acidosis; and acute and chronic metabolic alkalosis. Acute conditions occur over a relatively short period of time; chronic conditions occur over longer periods (hours and days) and are associated with compensation, usually by the kidney but occasionally by the lung. Acidemia occurs when there is an excess of hydrogen ions in the blood, and alkalemia occurs when there are too few hydrogen ions in the blood. Values associated with various blood gas disturbances are listed in Table 9.1 .
Measuring Arterial Blood Gases
Obtaining an Arterial Blood Gas Sample
Arterial blood can be sampled by needle puncture of a radial artery ( Fig. 9.1 ). Other sites, used occasionally but associated with a greater potential risk of complications, are the brachial and femoral arteries. Blood is collected into a heparinized syringe to prevent clotting. Air bubbles are expelled to prevent equilibration of the sample with ambient air, and the sample is kept on ice until analyzed. Routinely three measurements are obtained: Pa o 2 , Pa co 2 , and pH. Base excess (or deficit) and HCO 3 – (using the Henderson-Hasselbach equation ) can be calculated from the measured values for P co 2 and pH. (HCO 3 − can also be directly measured as part of serum electrolytes.) When frequent sampling of arterial blood is needed, a catheter can be inserted, most often in the radial artery.

Pulse Oximetry
Arterial puncture is uncomfortable for patients and is associated with a small but finite risk. Pulse oximetry is a noninvasive method of assessing arterial oxygenation that is now widely used in hospitalized patients. The sensor device can be clipped on the patient’s finger and oxygen saturation can be displayed continuously on a monitor. Specific wavelengths of light are passed through the finger, and the oximeter measures the pulsatile absorption of light by arteriolar blood. Because oxygenated and deoxygenated hemoglobin have different patterns of light absorption, they produce different results. The major advantage of pulse oximetry is that it provides a continuous measurement.
However, this method has two major limitations: (1) the oximeter measures O 2 saturation, not P o 2 ; and (2) it provides no information about P co 2 and pH.
Arterial pH
pH is the negative log of the hydrogen ion concentration. Measurement of arterial pH is the only way to determine whether the blood is too alkaline or too acid. Low pH values (<7.35) indicate acidemia or an increase in hydrogen ions; pH values greater than 7.45 indicate alkalemia, a decrease in hydrogen ions. Acidemia and alkalemia refer to conditions in the blood; acidosis and alkalosis refer to the process that causes the abnormality. Maintenance of a normal or near-normal pH in the blood is important, because arrhythmias can result when the pH falls below 7.25, and seizures and vascular collapse can occur when the pH rises above 7.55.
The acid–base status of blood can be analyzed using the Henderson-Hasselbach equation for the bicarbonate buffer system. This equation states that the pH in blood is equal to a constant (pK) plus the log ratio of bicarbonate to P co 2 . That is,
pH=pK+log[HCO3−]0.3×PCO2
The pK is a constant (pK = 6.1) that is related to the dissociation of carbonic acid (H 2 CO 3 ). The bicarbonate concentration is determined by the kidney, whereas the lung determines the P co 2 . When the HCO 3 – is constant, increases in P co 2 result in decreases in pH, and decreases in P co 2 result in increases in pH. As long as the ratio of bicarbonate to 0.03 P co 2 is 20, the pH remains at 7.4. Compensatory mechanisms in the body are responsible for maintaining the ratio of HCO 3 – to 0.03 P co 2 at 20.
Obtaining an Arterial Blood Gas Sample
Arterial blood can be sampled by needle puncture of a radial artery ( Fig. 9.1 ). Other sites, used occasionally but associated with a greater potential risk of complications, are the brachial and femoral arteries. Blood is collected into a heparinized syringe to prevent clotting. Air bubbles are expelled to prevent equilibration of the sample with ambient air, and the sample is kept on ice until analyzed. Routinely three measurements are obtained: Pa o 2 , Pa co 2 , and pH. Base excess (or deficit) and HCO 3 – (using the Henderson-Hasselbach equation ) can be calculated from the measured values for P co 2 and pH. (HCO 3 − can also be directly measured as part of serum electrolytes.) When frequent sampling of arterial blood is needed, a catheter can be inserted, most often in the radial artery.

Pulse Oximetry
Arterial puncture is uncomfortable for patients and is associated with a small but finite risk. Pulse oximetry is a noninvasive method of assessing arterial oxygenation that is now widely used in hospitalized patients. The sensor device can be clipped on the patient’s finger and oxygen saturation can be displayed continuously on a monitor. Specific wavelengths of light are passed through the finger, and the oximeter measures the pulsatile absorption of light by arteriolar blood. Because oxygenated and deoxygenated hemoglobin have different patterns of light absorption, they produce different results. The major advantage of pulse oximetry is that it provides a continuous measurement.
However, this method has two major limitations: (1) the oximeter measures O 2 saturation, not P o 2 ; and (2) it provides no information about P co 2 and pH.
Arterial pH
pH is the negative log of the hydrogen ion concentration. Measurement of arterial pH is the only way to determine whether the blood is too alkaline or too acid. Low pH values (<7.35) indicate acidemia or an increase in hydrogen ions; pH values greater than 7.45 indicate alkalemia, a decrease in hydrogen ions. Acidemia and alkalemia refer to conditions in the blood; acidosis and alkalosis refer to the process that causes the abnormality. Maintenance of a normal or near-normal pH in the blood is important, because arrhythmias can result when the pH falls below 7.25, and seizures and vascular collapse can occur when the pH rises above 7.55.
The acid–base status of blood can be analyzed using the Henderson-Hasselbach equation for the bicarbonate buffer system. This equation states that the pH in blood is equal to a constant (pK) plus the log ratio of bicarbonate to P co 2 . That is,
pH=pK+log[HCO3−]0.3×PCO2
The pK is a constant (pK = 6.1) that is related to the dissociation of carbonic acid (H 2 CO 3 ). The bicarbonate concentration is determined by the kidney, whereas the lung determines the P co 2 . When the HCO 3 – is constant, increases in P co 2 result in decreases in pH, and decreases in P co 2 result in increases in pH. As long as the ratio of bicarbonate to 0.03 P co 2 is 20, the pH remains at 7.4. Compensatory mechanisms in the body are responsible for maintaining the ratio of HCO 3 – to 0.03 P co 2 at 20.
Respiratory Acidosis and Alkalosis
Respiratory effects on acid–base status center around the elimination of CO 2 . The two basic respiratory alterations are respiratory acidosis and respiratory alkalosis ( Table 9.2 ). Respiratory acidosis is associated with a decrease in pH secondary to an increase in Paco 2 and is due to a decrease in the elimination of CO 2 by the lungs. Increases in Paco 2 can be due to either increased dead-space ventilation or hypoventilation (see Chapter 7 ). In contrast, respiratory alkalosis is associated with an increase in pH secondary to a decrease in Paco 2 and is due to an increase in the elimination of CO 2 by the lungs. Excess removal of CO 2 from the blood by the lungs is called hyperventilation .
P co 2 | pH | HCO 3 – | |
---|---|---|---|
Respiratory Acidosis | |||
No compensation | ↑↑ | ↓↓ | NL |
Metabolic compensation | ↑↑ | ↓ | ↑↑ |
Respiratory Alkalosis | |||
No compensation | ↓↓ | ↑↑ | NL |
Metabolic compensation | ↓↓ | ↑ | ↓↓ |
Metabolic Acidosis | |||
No compensation | NL | ↓↓ | ↓↓ |
Respiratory compensation | ↓ | ↓ | ↓↓ |
Metabolic Alkalosis | |||
No compensation | NL | ↑↑ | ↑↑ |
Respiratory compensation | ↑ | ↑ | ↑↑ |
A respiratory acidosis can be either acute or chronic. In an individual who has been given a moderate dose of a narcotic, a respiratory depressant, minute ventilation can decrease significantly and if this occurs, arterial P co 2 levels rise. The increase in P co 2 occurs over minutes and is associated with an immediate decrease in pH (see Henderson-Hasselbach equation). There is not sufficient time for any compensatory mechanism to occur. This results in an acute, or uncompensated, respiratory acidosis.
In individuals with chronic lung disease, the changes in gas exchange in the lung occur slowly, and as disease progresses, the arterial CO 2 levels begin to rise slowly. The CO 2 combines with water to form H 2 CO 3 , which dissociates to form H + and HCO 3 – . Prompted by the increase in P co 2 in the renal tubular cells, the kidney conserves HCO 3 – and excretes H + ions as H 2 PO 4 or NH 4 + . The increase in plasma HCO 3 – shifts the HCO 3 – /0.03 P co 2 ratio back toward normal levels. Renal compensation, however, like all other compensatory mechanisms, is not “perfect” or complete, and thus although the pH approaches 7.4, it remains slightly less than this value. In this example, although Pa co 2 rises, the change in pH is buffered by an increase in HCO 3 – ion. Some examples of diseases associated with respiratory acid–base balance disturbances are listed in Box 9.1 .
Respiratory Acidosis
- •
Central respiratory control center depression (narcotics, anesthetics, sedatives)
- •
Neuromuscular disorders (muscular dystrophy, myasthenia gravis, spinal cord injury)
- •
Chest wall restriction (kyphoscoliosis)
- •
Restrictive lung disease (pulmonary fibrosis, pneumothorax, pleural effusion, extreme obesity)
- •
Obstructive pulmonary disease (emphysema, chronic bronchitis, upper airway obstruction, cystic fibrosis)
Respiratory Alkalosis
- •
Hyperventilation (anxiety, encephalitis, tumors)
- •
Fever
- •
Acute asthma
- •
Pulmonary embolism
- •
Hypoxia, high altitude
- •
Salicylate ingestion
- •
Progesterone (hyperventilation of pregnancy)
Metabolic Acidosis and Alkalosis
Respiratory alterations in acid–base status are related to changes in CO 2 , and metabolic abnormalities are associated with either a gain or a loss of fixed acid or bicarbonate in the extracellular fluid. For example, with vomiting there is a loss of stomach acid; this results in a metabolic alkalosis due to loss of fixed acid. The lung is able to quickly compensate for these metabolic abnormalities by changing ventilation, resulting in either increased or decreased elimination of CO 2 . Thus a metabolic acidosis stimulates ventilation, CO 2 elimination, and a rise in pH toward normal levels, whereas a metabolic alkalosis suppresses ventilation and CO 2 elimination and the pH decreases toward the normal range. This is then followed by the slower elimination by the kidneys of excess acid or bicarbonate. For example, in individuals with uncontrolled diabetes, the increase in blood sugar is associated with an increase in ketones and the development of ketoacidosis. The pH in the blood decreases. This decrease in pH is buffered, however, by an increase in minute ventilation, resulting in a decrease in P co 2 followed by ketone elimination in the urine. Common diseases associated with metabolic acid–base balance disorders are listed in Box 9.2 .
Metabolic Acidosis
- •
Drug ingestion (methanol, ethanol, ethylene glycol, ammonium chloride)
- •
Diarrhea
- •
Renal dysfunction
- •
Lactic acidosis (shock, acute respiratory distress syndrome, carbon monoxide)
- •
Ketoacidosis (diabetes, starvation, alcoholism)
Metabolic Alkalosis
- •
Vomiting (nasogastric suctioning)
- •
Diuretics
- •
Antacid ingestion
Respiratory and Renal Compensatory Mechanisms
Homeostasis is a process of control mechanisms that helps stabilize body systems by returning them to a more normal state. Because cells are unable to function outside of a relatively narrow pH range, homeostatic or compensatory mechanisms are important for cell and organ survival. Compensatory mechanisms are quickly activated to offset disturbances in acid–base balance; as a result, it is unusual to see uncompensated primary acid-base abnormalities. Changes in function in the respiratory and renal systems are the major acid–base compensatory mechanisms. The respiratory system compensates for metabolic acidosis or alkalosis by altering alveolar ventilation. The kidneys compensate for a respiratory acidosis or metabolic acidosis of nonrenal origin by excreting fixed acids and by retaining filtered bicarbonate; they compensate for a respiratory alkalosis and metabolic alkalosis of nonrenal origin by decreasing hydrogen ion excretion and bicarbonate.
Most acid–base disorders are complex, with elements of both acute (uncompensated) and chronic (compensated) changes present. In examining acid–base abnormalities, then, the question frequently arises about what is the primary abnormality and what is the compensatory response. How is it possible to sort out the primary abnormality from the compensatory response? There are two important principles to remember. The first is that compensation is rarely complete; the second is that compensatory acid–base responses rarely overcompensate.
Respiratory Compensation
The respiratory system compensates for metabolic acid-base balance disorders by altering alveolar ventilation. When CO 2 production is constant, alveolar P co 2 is inversely proportional to alveolar ventilation (see Fig. 5.3 ). When a metabolic acidosis is present, the increased blood H + concentration stimulates chemoreceptors, which in turn stimulate alveolar ventilation and decrease arterial Pco 2 . Respiratory compensation for a metabolic alkalosis is to decrease alveolar ventilation, resulting in an increase in alveolar Pco 2 .
The relation between arterial levels of CO 2 and pH with and without renal compensation is demonstrated by the following two rules. For each increase of 10 mm Hg in Pa co 2 , the pH will fall 0.03 units if time is allowed for renal compensation, and 0.08 units if the process is acute and insufficient time for renal compensation has occurred. (Renal compensation takes around 48 hours to occur.) For example, is the following elevation in Pa co 2 acute or chronic?
pH7.20;PaCO265mmHg
If the increase in Pa co 2 is acute, an increase in Pa co 2 from 40 to 65 mm Hg will result in a decrease in pH; that is,
0.08×25/10mmHg=0.20units
7.40−0.20=7.20
If the increase in Pa co 2 is chronic, an increase in Pa co 2 from 40 to 65 mm Hg will result in a decrease in pH; that is,
0.03×25/10mmHg=0.075units
7.40−0.075=7.32
Therefore in this example, the elevation in Pa co 2 is entirely acute. Note that if the process had occurred over days, renal compensation would have occurred and the change in pH would have been smaller—that is, closer to 7.32 instead of 7.20.
Although it is useful to think about acid–base changes as either acute or chronic, with few exceptions (such as the acute administration of a respiratory depressant in the earlier example), compensation for changes in P co 2 begins immediately. Examine now the following pH and P co 2 combination.
pH7.28;PCO270mmHg
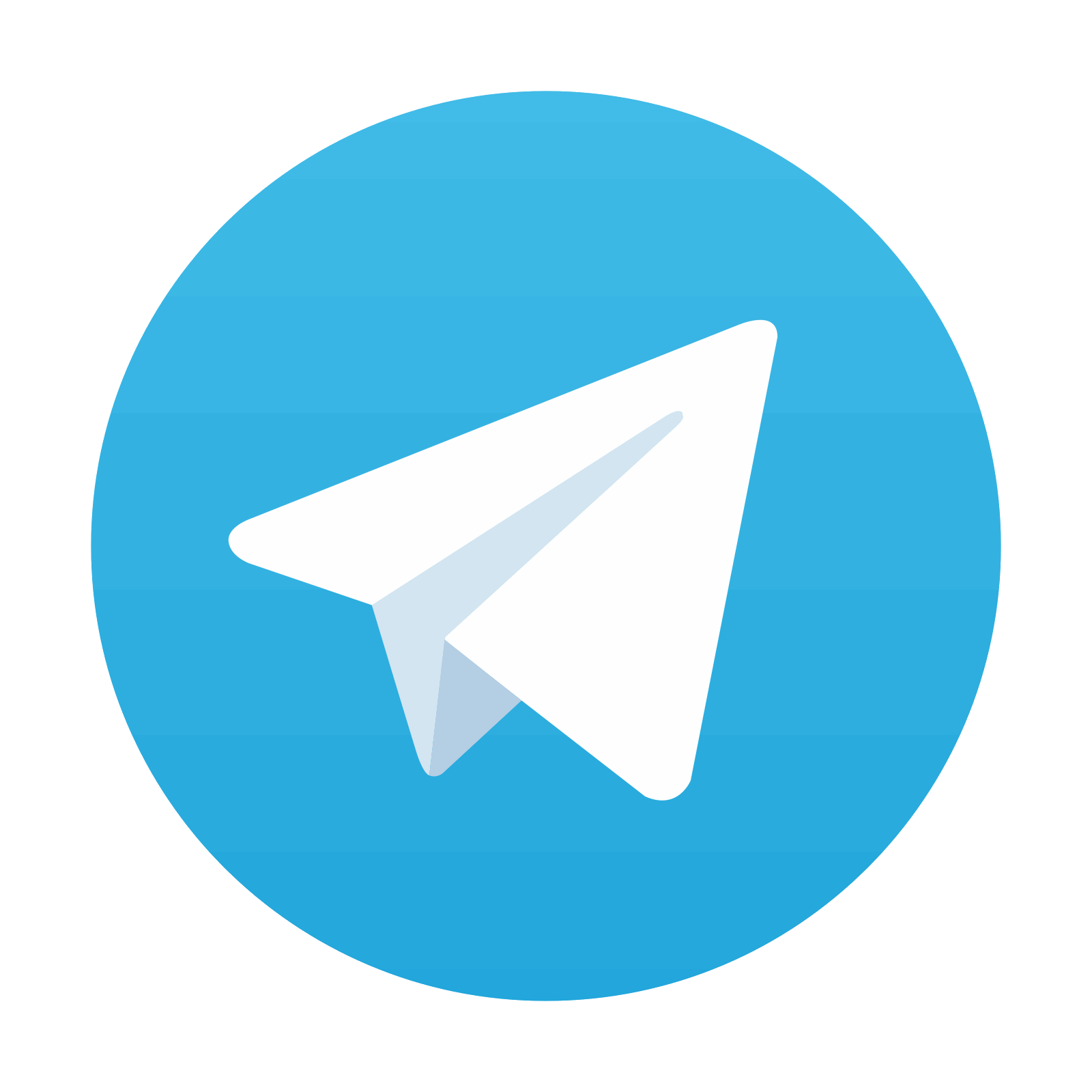
Stay updated, free articles. Join our Telegram channel

Full access? Get Clinical Tree
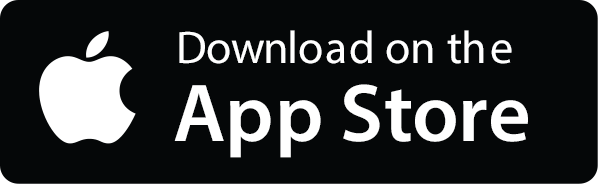
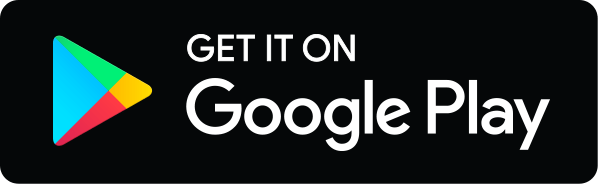
