Introduction
Pulmonary alveolar proteinosis (PAP) is a rare syndrome characterized by abnormal alveolar surfactant accumulation and hypoxemic respiratory insufficiency. It arises in a heterogeneous group of mechanistically distinct diseases usefully divided into disorders of surfactant production or surfactant clearance . Disorders of production include pulmonary surfactant metabolic dysfunction (PSMD) disorders caused by mutations in genes encoding surfactant proteins or genes encoding proteins involved in surfactant production. Disorders in clearance can be further divided into primary PAP caused by disruption of granulocyte/macrophage colony-stimulating factor (GM-CSF) signaling and secondary PAP caused by another disease or condition that disrupts alveolar macrophage surfactant clearance.
Recent advances including reports of several large cohorts have greatly improved our understanding of PAP pathogenesis, epidemiology, clinical subtypes, prognosis, and natural history and have resulted in new methods to diagnose, evaluate, and treat patients. Importantly, research on this rare and fascinating syndrome has identified the critical role of GM-CSF in alveolar macrophage ontogeny, pulmonary surfactant homeostasis, alveolar structural integrity, host defense, pulmonary and systemic inflammation, and autoimmunity. Further, studies involving GM-CSF–deficient mice identified new treatment approaches for common diseases such as asthma and rheumatoid arthritis. This chapter reviews the pathogenesis, classification, epidemiology, presentation, and therapy of PAP.
Pathogenesis
Surfactant Composition and Homeostasis
Surfactant is a complex mixture of 90% phospholipids and 10% surfactant proteins that functions at the air-liquid–alveolar wall interface to reduce surface tension, thereby preventing alveolar collapse. It is produced in type II alveolar epithelial cells, secreted into the alveolar space, and cleared by either recycling or catabolism by type II cells or uptake and catabolism by alveolar macrophages. Surfactant composition, production, and homeostasis are reviewed in Chapter 8 . Studies in animals and humans in which the pathogenesis of PAP has been elucidated have identified GM-CSF as a critical regulator of surfactant homeostasis ( Fig. 70-1 ).

Granulocyte/Macrophage Colony-Stimulating Factor
GM-CSF is a 23-kD cytokine that signals via cell surface receptors comprising a GM-CSF–binding, low-affinity α chain (CDw116) and a nonbinding, affinity-converting β chain (CD131). Although neither chain has intrinsic signaling activity, the β chain constitutively binds Janus kinase 2 (JAK2), a tyrosine kinase involved in cytokine signaling. Ligand binding causes the formation of αβJAK2 multimers and activation of JAK2, resulting in phosphorylation of α and β chains, activation of other kinases, and initiation of signaling via multiple pathways including the signal transducer of activation and transcription-5 (STAT5).
Murine Models of Disordered Surfactant Homeostasis
GM-CSF–deficient ( Csf2 KO ) mice provided the first clue to the pathogenesis of PAP. Although neither production of surfactant by type II cells nor its uptake by alveolar macrophages was altered, clearance of surfactant by alveolar macrophages was impaired. Pulmonary GM-CSF, acting via the transcription factor PU.1, coordinately regulated surfactant catabolism; cell adhesion; expression of Fc receptors, mannose receptors, and other receptors; phagocytosis; bacterial killing; and Toll-like receptor-4 signaling. GM-CSF was identified as a critical regulator of the terminal differentiation of alveolar macrophages in mice.
GM-CSF receptor β chain–deficient ( Csf2rb KO ) mice develop PAP indistinguishable from that of GM-CSF–deficient mice. Because transplantation of wild-type bone marrow corrected PAP in these mice, myeloid cells, and not lung resident epithelial cells, were identified as the cellular site of pathogenesis. A biomarker of PAP in these mice (increased serum GM-CSF) suggested the means that led to identification of hereditary PAP caused by CSF2RA and CSF2RB mutations in children. Importantly, Csf2rb KO mice and children with CSF2RA or CSF2RB mutations develop a lung disease that is identical in every respect including lung pathology (surfactant-filled alveoli, preserved architecture); cytopathology (oil red O–stained macrophages, cell debris); alveolar macrophage biomarkers ( reduced messenger RNA [mRNA] for PU.1, peroxisome proliferator–activated receptor -γ [PPAR-γ], ABCG1); and BAL biomarkers (increased surfactant proteins/lipids, turbidity, cytokines [GM-CSF, M-CSF, MCP-1]) and clinical course (progressive surfactant accumulation). Studies in these mice have identified pulmonary macrophage transplantation (PMT) as a novel therapeutic approach for hereditary PAP.
Mice deficient in ABCG1, a transmembrane protein mediating cellular cholesterol efflux, accumulate cholesterol in alveolar macrophages and type II cells and develop pulmonary lipidosis, indicating ABCG1 may be important in surfactant homeostasis. Importantly, ABCG1 expression is decreased in alveolar macrophages from GM-CSF–deficient mice, patients with autoimmune PAP, GM-CSF receptor–deficient mice, and patients with hereditary PAP (unpublished data). Conditional disruption of PPAR-γ (a known transcriptional regulator of ABCG1) specifically in alveolar macrophages also disrupts surfactant clearance.
Together, murine studies suggest the pathogenesis of primary PAP, both autoimmune and hereditary, may involve disruption of a pathway including GM-CSF, PU.1, PPAR-γ, and ABCG1 that is critical for surfactant clearance by alveolar macrophages.
In secondary PAP, several models have shed light on the pathogenesis. For example, reduction of alveolar macrophage numbers by chemical depletion increases surfactant pool size and inhalation of respirable silica results in alveolar proteinosis.
In addition to these models of surfactant clearance, murine models of abnormal surfactant production called PSMD disorders develop lung diseases faithfully recapitulating their respective human diseases. They are clinically, histologically, and biochemically distinct from PAP in GM-CSF–deficient mice and have improved the understanding of surfactant homeostasis. For example, SP-B–deficient mice develop respiratory failure at birth. Studies in these mice showed that SP-B is critical in the post-translational processing of SP-C, organization of surfactant phospholipids in lamellar bodies, formation of tubular myelin in alveoli, generation of surfactant films capable of reducing surface tension, and lung function during the early postnatal period. ABCA3-deficient mice develop respiratory failure at birth, and studies in these mice established ABCA3 as critical to lamellar body formation and pulmonary surfactant biogenesis.
Other murine models have been shown to develop PAP and are providing insights to surfactant homeostasis including murine models of severe combined immunodeficiency, pulmonary overexpression of interleukin (IL)-4 or IL-13, and SP-D–deficiency.
Role of GM-CSF Autoantibodies in Primary PAP
In 1999, Nakata’s group first identified neutralizing GM-CSF autoantibodies in the BAL fluid and serum of idiopathic, now called autoimmune, PAP patients. Multiple subsequent studies confirmed that high levels of GM-CSF autoantibodies are associated with idiopathic PAP but not with secondary PAP, other lung diseases, PSMD disorders, or healthy individuals ( Fig. 70-2 ). In one study, the concentration of GM-CSF autoantibodies in 158 idiopathic PAP patients was 113 ± 7 µg/mL, while levels were less than 1 µg/mL in patients with secondary PAP, PSMD disorders, or other lung diseases. GM-CSF autoantibodies are composed of IgG, predominantly of the IgG1 and IgG2 subclasses; are highly specific for GM-CSF; have a high binding affinity (20 ± 7.5 pM); and are capable of neutralizing GM-CSF at levels up to many thousands times higher than the physiologic concentrations of GM-CSF in vivo. Notwithstanding these important observations, GM-CSF autoantibodies have been reported at low frequency in healthy people and in patients with autoimmune diseases without evidence of PAP; in addition, GM-CSF comprises the dominant anti-cytokine activity in pharmaceutical immunoglobulin prepared from healthy individuals. In a more recent study utilizing multiple detection methods, GM-CSF autoantibodies were demonstrated in all of 72 healthy individuals with no prior exposure to exogenously administered GM-CSF, albeit at low levels (median [interquartile range] = 1.04 [0.63 to 1.7] µg/mL serum). Another confusing observation was that in patients with PAP, serum GM-CSF autoantibodies did not correlate with disease severity.

To prove that GM-CSF autoantibodies were critical to the pathogenesis of idiopathic PAP and not a related but unimportant epiphenomenon, GM-CSF autoantibodies from these patients were isolated in pure form (i.e., a single band on SDS gels) and used to immunize healthy nonhuman primates. Results demonstrated unequivocally that GM-CSF autoantibodies reproduced the cardinal molecular and cellular features of PAP, including surfactant-filled alveoli with preserved wall architecture, foamy alveolar macrophages, alveolar macrophage biomarkers (reduced PU.1, PPAR-γ, and ABCG1 mRNA), and BAL biomarkers (increased SP-D and turbidity). Evaluation of GM-CSF autoantibodies isolated from the immunized primates or directly from patients has similar bioactivity. Together with the clinical studies in humans, these data provided strong evidence that GM-CSF autoantibodies were in fact the pathogenic driver in idiopathic PAP, which is now recognized as autoimmune PAP. Neutrophils from the immunized primates also had impaired phagocytosis similar to defects reported in these patients, in GM-CSF deficient mice, and in normal human neutrophils incubated with purified GM-CSF autoantibodies in vitro. Using the CD11b stimulation index assay (see later), GM-CSF signaling in immunized primates was found to decrease inversely with GM-CSF autoantibody concentration at values below 5 mcg/mL and was zero at higher values, thus defining the critical threshold concentration needed to block GM-CSF signaling. In a translational study using this assay, a similar relationship and threshold were observed in humans. Thus seemingly discrepant observations (i.e., the critical role of GM-CSF autoantibodies in PAP pathogenesis and the lack of correlation between their concentration and disease severity) were reconciled by the hypothesis that a critical threshold level of GM-CSF autoantibodies was needed to reduce GM-CSF bioactivity sufficiently to impair surfactant clearance.
GM-CSF autoantibodies are polyclonal and directed at epitopes throughout the GM-CSF molecule. Evaluation of 19 monoclonal autoantibodies from 6 patients demonstrated that they use multiple immunoglobulin V genes and targeted at least four non-overlapping epitopes on GM-CSF, suggesting that GM-CSF is driving the autoantibodies and not a B-cell epitope on a pathogen cross-reacting with GM-CSF.
The observations that GM-CSF stimulation of neutrophil functions is impaired in autoimmune PAP and GM-CSF deficient mice provides a further explanation for the increased infection risk in PAP caused by disruption of GM-CSF signaling. Further, the inverse correlation between GM-CSF autoantibody levels and neutrophil function (phagocytosis) in healthy people suggests a potential physiologic role for these autoantibodies, such as scavenging free GM-CSF, which can function as a proinflammatory cytokine. Supporting this concept, using methods capable of detecting GM-CSF, whether bound by GM-CSF autoantibody or not, serum GM-CSF concentrations in healthy people (3048 ± 484 pg/mL serum, n = 11) are much higher than previously reported, and more than 99% is bound to GM-CSF autoantibodies both in healthy individuals and in PAP patients.
Genetic Factors
A series of reports have now established hereditary PAP as a newly described genetic disease caused by genetic mutations in CSF2RA or CSF2RB , which encode GM-CSF receptor α or β chains, respectively ( Fig. 70-3 ). In some cases, the genes have been cloned, the defects reproduced in vitro, the signaling abnormalities studied in detail, 23-,25 and the results used to develop several novel biomarker-based diagnostic tests (see later). One particularly useful test, serum GM-CSF, was used to identify a number of patients with hereditary PAP due to mutations in the GM-CSF receptors and is capable of identifying patients with defects in either chain of the receptor. Of importance to the pathogenesis of PAP, in families with two siblings carrying the same function-impairing GM-CSF receptor gene mutation, the older sibling had minimal disease while the younger sibling had more extensive disease, suggesting another factor besides loss of GM-CSF signaling is important in determining disease severity in hereditary PAP. Several genetic factors associated with hematologic disease and secondary PAP have been identified including mutations in the gene encoding the transcription factor GATA2, as well as in GMCSF receptor chain genes.

Mutations in three genes encoding surfactant proteins, SFTPB, SFTPC, and ABCA3, disrupt surfactant production and function and cause respiratory disease in neonates, children, and older individuals. See Chapter 8 for additional information on pulmonary surfactant. SP-B and SP-C are hydrophobic peptides that reside within the surfactant phospholipid layer, which functions to reduce surface tension at the alveolar air/liquid interface, whereas ABCA3 likely transports lipids, including phosphatidylcholine, cholesterol, sphingomyelin, and phosphatidylglycerol, into lamellar bodies in alveolar type II cells, where the surfactant complex is assembled, processed, and stored.
SFTPB is a small gene (9.7 kb) encoding a 79-amino acid, hydrophobic protein. Infants homozygous for recessive loss-of-function mutations in SFTPB develop respiratory failure and die shortly after birth. In contrast, individuals heterozygous for SP-B deficiency alleles have normal lung function. More than 30 mutations have been identified to date in fewer than 100 infants with SP-B deficiency. Two thirds have an insertional frameshift mutation caused by the replacement of a single nucleotide by three nucleotides at codon 121 (designated 121ins2). Among infants with SP-B deficiency, approximately 60% are homozygous for the 121ins2 mutation, 25% are heterozygous for this and another function-altering allele, and 15% have other mutations. SP-B deficiency is associated with the aberrant processing and secretion of an immature SP-C peptide, which may contribute to the respiratory failure associated with hereditary SP-B deficiency.
SFTPC is a small gene (3 kb) encoding a 35-amino acid hydrophobic protein. Known mutations in SFTPC are expressed in a dominant fashion and are associated with interstitial lung disease in neonates, children, and adults. Twenty-five percent of individuals with SP-C–associated lung disease have a point mutation in SFTPC causing the substitution of threonine for isoleucine at codon 73 (I73T). Immunohistochemical analysis of lung tissue from an infant with the I73T mutation demonstrated normal staining patterns for pro-SP-B, SP-B, and pro-SP-C. Although infants with this mutation can present with respiratory distress syndrome, most children with the mutation become symptomatic with interstitial lung disease beyond the newborn period.
ABCA3 is a large gene (80 kb) encoding a 1704-amino acid protein. Autosomal recessive mutations in ABCA3 can result in fatal surfactant deficiency in neonates and chronic respiratory insufficiency in older children. Function-altering mutations in ABCA3 result in surfactant that is deficient in phosphatidylcholine and has decreased function, suggesting that ABCA3 has an important role in pulmonary surfactant phospholipid homeostasis. A relatively common missense point mutation in ABCA3 causing the substitution of a valine for glutamic acid at codon 292 (E292V) is associated with chronic interstitial lung disease in older children who are heterozygous for this and a different function-altering mutation on the other ABCA3 allele.
NKX2.1 is essential for expression of SP-B, SP-C, and ABCA3. Haploinsufficiency of the gene encoding for the transcription factor NKX2.1 (also known as thyroid transcription factor 1 or TTF1) causes a complex phenotype in neonates that includes, albeit variably, hypothyroidism, brain abnormalities, and acute and chronic lung disease.
Although the lung diseases associated with mutations in SP-B, SP-C, ABCA3, and NKX2.1 disrupt surfactant homeostasis, cause varying degrees of surfactant accumulation (i.e., PAP), and are often cited as hereditary forms of PAP in the medical literature, they are distinguished from disorders of surfactant clearance by their surfactant dysfunction (i.e., functionally abnormal surfactant), histopathologic abnormalities (gross parenchymal derangement and interstitial disease), and clinical course. Consequently, the term pulmonary surfactant metabolic dysfunction (PSMD) disorders has been proposed for this group of genetic disorders.
Disease Associations
PAP has been reported in association with various medical conditions believed to cause the disorder, resulting in use of the term secondary PAP. Systemic disorders associated with secondary PAP include malignant and other hematologic diseases, nonhematologic malignancies, immune deficiency syndromes, chronic inflammatory syndromes, and chronic infections ( Table 70-1 ). In the past, chronic myelogenous leukemia and myelodysplastic syndrome were the most frequently reported hematologic disorders associated with PAP. In one single-institution study, PAP was reported in 5.3% of patients with hematologic malignancies and in 8.8% of those who were neutropenic. Of possible pathogenic significance, 9 of 10 patients in one study were neutropenic when PAP developed and, in most, PAP recovered spontaneously after bone marrow engraftment. In another, PAP developed during a period of neutropenia that resolved after treatment with granulocyte colony-stimulating factor. In a third report, PAP developed in three patients with acute myeloid leukemia with GM-CSF receptor abnormalities, including reduced levels of the β chain (three of three) and undetectable α chain (two of three). PAP has been found in about 10% of patients with lysinuric protein intolerance, an ultra-rare inherited disorder caused by defective cationic amino acid transport. Secondary PAP has been reported in association with various forms of immunodeficiency, including thymic alymphoplasia, immunoglobulin A deficiency, solid organ transplantation, and acquired immunodeficiency syndrome.
Disease Category/Underlying Disease | Reference |
---|---|
HEMATOLOGIC DISORDERS | |
Acute lymphocytic leukemia | |
Acute myeloid leukemia | |
Aplastic anemia | |
Chronic lymphocytic leukemia | |
Chronic myeloid leukemia | |
Myelodysplastic syndromes | |
Multiple myeloma | |
Lymphoma | |
Waldenstrom macroglobulinemia | |
NONHEMATOLOGIC MALIGNANCIES | |
Adenocarcinoma | |
Glioblastoma | |
Melanoma | |
IMMUNE DEFICIENCY AND CHRONIC INFLAMMATORY SYNDROMES | |
Acquired immunodeficiency syndrome | |
Amyloidosis | |
Agammaglobulinemia | |
Fanconi syndrome | |
Juvenile dermatomyositis | |
Lysinuric protein intolerance | |
Renal tubular acidosis | |
Severe combined immunodeficiency disease | |
CHRONIC INFECTIONS | |
Cytomegalovirus | |
Mycobacterium tuberculosis | |
Nocardia | |
Pneumocystis jirovecii (formerly carinii ) |
Although the mechanism(s) responsible for secondary PAP are poorly studied, two common themes have emerged when considered in the context of the critical role of alveolar macrophages in surfactant homeostasis. Conditions reducing either the numbers or functions of alveolar macrophages would be expected to reduce the capacity of resident alveolar macrophages to clear surfactant from the lung surface.
Environmental Factors
PAP has been associated with inhalation exposure to environmental agents including cigarette smoke, a wide variety of organic and inorganic dusts, fumes, and gases ( Table 70-2 ). Epidemiologic studies (see later) document that PAP is more common in smokers. Five of the 27 original patients reported by Rosen and colleagues had been exposed to wood dust. Most reports implicating other environmental exposures are single cases or small retrospective series and, in general, have not established a causal relationship between the exposure and development of PAP. One early report on 138 PAP patients found that half had significant exposure to dust and fumes, of which the most common exposure was to inhaled silica, noted in 10 individuals. With the advent of occupational protective equipment in the workplace, this form of “acute silicoproteinosis” is rare. Other case reports document the association of PAP with exposure to aluminum dust, cellulose fibers, cement dust, titanium dioxide, indium-tin oxide, and nitrogen dioxide. The link between silica and PAP is supported by studies in rats demonstrating that inhalation of silica particles disrupts surfactant homeostasis, resulting in increased surfactant accumulation. In five separate large series of patients with PAP, a history of dust exposure, when data were available, was present in 26% to 54% of patients. Further research is necessary to determine what role environmental inhalation exposure plays in the pathogenesis of PAP.
Exposure Category/Agent | Reference |
---|---|
DUSTS (INORGANIC) | |
Aluminum | , |
Cement | |
Silica | , |
Titanium | |
Indium | |
ORGANIC DUSTS | |
Agricultural | |
Bakery flour | |
Fertilizer | |
Sawdust | |
FUMES | |
Chlorine | |
Cleaning products | |
Gasoline/petroleum | |
Nitrogen dioxide | |
Paint | |
Synthetic plastic fumes | |
Varnish |
Mechanisms of Disruption of Surfactant Homeostasis
In mice, a series of observations has established GM-CSF as a critical regulator of pulmonary surfactant homeostasis, including the following: (1) surfactant homeostasis requires the presence of GM-CSF in the lung, and PAP develops in the absence of either pulmonary GM-CSF or its receptor; (2) surfactant accumulation in GM-CSF–deficient mice is caused by decreased catabolism by alveolar macrophages and not by increased production; (3) pulmonary GM-CSF is required for normal expression of transcription factor PU.1 in alveolar macrophages, and enforced (i.e., retroviral-mediated) expression of PU.1 restores surfactant catabolism in alveolar macrophages from GM-CSF knockout mice; and (4) surfactant homeostasis can be restored in GM-CSF receptor β-deficient mice by transplantation of bone marrow from a wild-type mouse.
Primary PAP (Autoimmune and Hereditary)
In humans, studies elucidating the pathogenesis of autoimmune and hereditary PAP show that GM-CSF is also critical for surfactant homeostasis in humans. Supportive data include (1) GM-CSF autoantibodies (a) are present at high levels only in autoimmune PAP, (b) can be isolated in pure form, (c) cause PAP after injection into healthy primates, and (d) retain biologic activity after injection ; (2) GM-CSF autoantibodies neutralize vastly higher amounts of GM-CSF than is present physiologically ; (3) in PAP patients, PU.1 expression is reduced in alveolar macrophages and increased by GM-CSF therapy ; (4) disruption of GM-CSF signaling by CSF2RA or CSF2RB mutations causes lung disease histopathology indistinguishable from that of GM-CSF knockout mice and autoimmune PAP in humans ; (5) reduced expression of GM-CSF receptor β on leukocytes is associated with PAP in children and with development of PAP in leukemia patients ; and (6) disorders that transiently reduce numbers and/or functions of alveolar macrophages are temporally related to the development and resolution of secondary PAP in humans.
Available evidence suggests that GM-CSF regulates myeloid cell functions similarly in humans and mice. GM-CSF knockout mice and autoimmune PAP patients have remarkable similarity in (1) pulmonary histopathologic appearance of the lung; (2) cytologic and ultrastructural appearance of alveolar macrophages; (3) elevation of certain cytokines (monocyte chemotactic protein-1, macrophage colony-stimulating factor); (4) the pattern of differential impairment of various neutrophil functions; and (5) the increased incidence of pulmonary and extrathoracic secondary infections.
The pathogenic mechanism in primary PAP is (1) disruption of GM-CSF signaling to alveolar macrophages, either by the absence of GM-CSF (observed in mice, but not yet in humans); (2) disruption of GM-CSF signaling by mutations in the GM-CSF receptor proteins (in mice and humans), or (3) disruption of GM-CSF by an autoimmune attack that neutralizes GM-CSF, eliminating GM-CSF bioactivity and myeloid cell stimulation in vivo (in humans and nonhuman primates). GM-CSF, via PU.1, regulates catabolism of surfactant in alveolar macrophages (in mice and possibly humans). Surfactant accumulation in PAP is due to reduced clearance by alveolar macrophages, but the precise mechanism is unknown. Because pulmonary surfactant is internalized into macrophages by endocytosis and catabolized in phagolysosomes, it is possible that interruption of GM-CSF signaling blocks the translocation of endocytosed surfactant to lysosomes. Such a mechanism has been demonstrated for adenovirus internalized by macrophages from GM-CSF–deficient mice, in which PU.1 redirects adenovirus-containing endosomes to lysosomes for destruction. Alternatively, GM-CSF deficiency may result in a key enzyme deficiency. Potentially supporting this possibility are data related to the peroxisome proliferator–activated receptor-γ (PPAR-γ), a ligand-activated transcription factor regulating genes involved in lipid metabolism and other pathways. PPAR-γ messenger RNA and protein are expressed in alveolar macrophages in healthy individuals, decreased in PAP patients, and increased by GM-CSF therapy of PAP patients. CD36, a lipid scavenger receptor regulated by PPAR-γ, is regulated in a similar pattern. However, PPAR-γ deficiency has been noted in several other disorders, including sarcoidosis, acute respiratory distress syndrome, and asthma, where there have been reports of surfactant abnormalities. Further studies are required to determine the mechanism by which interruption of GM-CSF signaling leads to reduced catabolism of surfactant by alveolar macrophages.
Secondary PAP
The mechanisms involved in secondary PAP, although less well supported by experimental data, likely involve reduced numbers or functions of alveolar macrophages. This would decrease the surfactant clearance capacity of resident alveolar macrophages and is supported by data demonstrating a temporal relationship of the onset and resolution of PAP with the suppression and restoration of myeloid cells in hematologic disorders. Other secondary mechanisms of PAP—for example, due to inhalation of toxic fumes and dusts—are less clear. Presumably, they would involve a reduction in the ability of alveolar macrophages to clear surfactant. However, because overexpression of certain cytokines (IL-4, IL-13) or decreased expression of some surfactant proteins (SP-D) also increases surfactant production in mice, other potential mechanisms appear possible.
Pulmonary Surfactant Metabolic Dysfunction
Mechanisms of PSMD involve mutations in genes of the surfactant synthetic pathway, including SFTPB, SFTPC, ABCA3, NKX2.1 , and likely others, and result in the production of biochemically and functionally abnormal surfactant. These disorders have a lung histopathology and clinical course that is markedly different from PAP caused by interruption of GM-CSF signaling. Further, the pathophysiology arises from surfactant deficiency rather than surfactant excess as in PAP. Thus it may be appropriate to consider these disorders as distinct from PAP and not part of a spectrum of PAP.
Nomenclature and Classification
Since PAP was first described as a lung disease “of the filling of the alveoli by a PAS-positive proteinaceous material, rich in lipid,” it has become recognized as a syndrome caused by distinct diseases and is reported in the medical literature using numerous terms, resulting in a nomenclature that is redundant, inconsistent, and confusing. Terms have been based on the biochemical characteristics of the accumulated material (e.g., alveolar proteinosis, phospholipidosis); pathogenesis (e.g., silicoproteinosis, idiopathic); disease category (e.g., primary vs. secondary); and timing of symptom onset (e.g., congenital, acquired) and are redundant (e.g., idiopathic PAP, acquired PAP, pulmonary alveolar lipoproteinosis, phospholipoproteinosis, alveolar lipoproteinosis, alveolar proteinosis, alveolar phospholipidosis, and alveolar lipophosphoproteinosis). Confusion arises from use of the same term for different diseases. For example, the term congenital PAP has been used for the disease caused by mutations in SFTPB , which is uniformly fatal at birth and caused by surfactant deficiency, and also for the disease caused by GM-CSF receptor deficiency that presents in children, is caused by surfactant accumulation, and can be treated successfully.
The foregoing discussion underscores the need for a classification and nomenclature that will facilitate clear communication about disorders of homeostasis necessary for clinical training, disease management, and research. On the basis of initial discussions of a global task force assembled by the Rare Lung Diseases Consortium, a simplified classified scheme is proposed ( Table 70-3 ).
CLINICAL CATEGORY/DISEASE |
Primary PAP |
|
Secondary PAP |
|
Pulmonary Surfactant Metabolic Dysfunction Disorders |
|
Disorders of surfactant homeostasis are separated into disorders of reduced clearance (PAP) or abnormal production (PSMD). PAP is further subdivided into primary and secondary categories, depending on whether it is due to a primary disturbance of GM-CSF signaling or secondary to another disease, respectively. Primary PAP is composed of several specific disease mechanisms: an autoimmune disease caused by GM-CSF autoantibodies (autoimmune PAP) or a genetic disease caused by mutations in CSF2RA or CSFR2B. A third disease, caused by GM-CSF deficiency, exists in mice and is predicted but not yet observed in humans. Secondary PAP arises in association with several disease categories, including hematologic disorders, malignancy, immune deficiency syndromes, chronic inflammatory disorders, and chronic infections (see Table 70-1 ), as well as in association with certain inhalation exposures, especially silica (sandblasters) (see Table 70-2 ). PSMD disorders are those arising from mutations in genes of the surfactant synthesis pathway, including SFTPB, SFTPC, ABCA3, NKX2.1, and likely others that have yet to be identified. These disorders are included in a new classification of interstitial lung disease in young children.
The plethora of terms used to report on the PAP syndrome are not recommended for continued use and should be replaced with the term pulmonary alveolar proteinosis. Terms referring to clinical presentation (i.e., acquired PAP, congenital PAP) should not be used when the underlying disease is known. For example, “SP-B deficiency” is far more informative than “congenital PAP” and more consistent with its pathogenesis as a disorder of surfactant deficiency (PSMD) rather than excessive surfactant accumulation (PAP). When the cause is unknown, the term unclassified PAP is preferred over “idiopathic PAP” because the latter is currently understood to be the disorder caused by high levels of GM-CSF autoantibodies.
Epidemiology
Disorders of surfactant homeostasis are found in worldwide distribution but are rare. Since the initial description of PAP, more than 1000 cases of primary or secondary PAP and substantially fewer cases of PSMD have been reported in the medical literature ( Table 70-4 ). A comprehensive meta-analysis of 410 separate cases of PAP representing all clinical subtypes found that patients were more likely to be male (male-to-female ratio = 2.65:1.0) and that males predominated among smokers (male-to-female ratio = 2.78:1.0) but not among nonsmokers (male-to-female ratio = 0.69:1.0). These results suggested that the high proportion of males among PAP patients may be explained by their higher frequency of tobacco use. This study also found the median age at diagnosis to be 39 years (39 in men and 35 in women).
Seymour ( n = 410) | Inoue ( n = 233) | Xu ( n = 241) | Bonella ( n = 70) | Campo ( n = 81) | |
---|---|---|---|---|---|
Age at Diagnosis (mean, range) | 39 (30–46) | 51 (41–58) | 42 (na) | 43 (18–78) | 40 (26–54) |
Ratio Male/Female | 2.6 | 2.0 | 2.2 | 1.3 | 2.0 |
Primary PAP (%) | na | 90 | na | 91 | 90 |
Secondary PAP (%) | na | 10 | na | 9 | 4 |
Time to diagnosis (mo) | 7 (3–19) | 10 (4–36) | na | 9 (1–36) | 11 (0–27) |
Spontaneous remitters (%) | 6 | 5 | na | 5 | 7 |
Smoking habits (%) | |||||
Never | 28 | 43 | — | 21 | 36 |
Previous | na | 29 | — | 30 | 42 |
Current | na | 29 | — | 49 | 22 |
Dust exposure (%) | na | 26 | na | 54 | 32 |
Autoimmune PAP has been studied in several ways to establish its epidemiology. A large national, multicenter, registry-based study of PAP was recently conducted in Japan and identified 223 patients with autoimmune PAP. This study reported a male predominance (male-to-female ratio = 2.1:1.0) and found that males predominated among smokers (male-to-female ratio = 9.3:1.0) but not among nonsmokers (male-to-female ratio = 0.6:1.0). The median age at the time of diagnosis was 53 years (52 in men and 55 in women). The prevalence was similar in nine nonoverlapping geographic regions encompassing the entirety of Japan, and the case rate correlated closely with the regional population size. This result suggests the absence of an effect of climate or geography on the prevalence. Further supporting this, autoimmune PAP is seen in a worldwide distribution. An intensive screening within the Niigata prefecture in Japan (population 2.41 million) estimated the incidence and prevalence of autoimmune PAP to be 0.49 ± 0.13 and 6.2 per million, respectively. This registry found that, among noncongenital cases of PAP, 89.9% of cases were autoimmune, 9.7% were secondary, and 0.4% were unclassified. In a meta-analysis of 1045 primarily adult PAP patients in five recent series, the mean age at diagnosis was similar in 4 out of 5 series (ranging from 39 to 43) and slightly older in the Japanese series (mean age of 51). In all series, males prevailed over females, with a ratio ranging from 1.3:1 to 2.6:1. The interval between onset of symptoms and diagnosis was similar (ranging from 7 to 11 months). Importantly, between 21% and 43% of PAP patients were never-smokers, indicating that PAP is not simply a smoking-related disease.
Hereditary PAP is caused by various genetic mutations in CSF2RA or CSF2RB genes (see Fig. 70-3 ) and usually presents in children between the ages of 1.5 and 11 years, although it has been observed to present in adults aged 29 and 35 years.
Secondary PAP accounts for not more than 10% of all PAP cases in adults and develops as a consequence of an underlying disease (see Table 70-1 ) or environmental exposure (see Table 70-2 ). Thus the prevalence of secondary PAP is estimated to be approximately 0.34 per 100,000 individuals in the general population. Historically, hematologic malignancy was the most common cause of secondary PAP, accounting for up to 5.3% of cases. However, in a recent study, myelodysplastic syndrome accounted for 65% of cases. Secondary PAP arises as a function of the underlying clinical disorder and, in some cases, can resolve with successful treatment of the underlying disorder.
PSMD disorders develop in individuals with mutations in the genes involved in surfactant synthesis: SFTPB, SFTPC, ABCA3, and NKX2.1, as discussed earlier. The incidence of SP-B deficiency is estimated to be approximately 1 per 1.5 million births. Among infants with respiratory distress syndrome of unknown etiology, mutations appear more commonly in ABCA3 than in SFTPB . The prevalence of the common disease-associated mutations in the SFTPB (121ins2), SFTPC (I73T), and ABCA3 (E292V) genes are not well established. However, a recent study in ethnically diverse cohorts from Missouri, Norway, South Korea, and South Africa ( n = 420) found the population-based frequencies of these mutations to be rare (<0.4%). Further, E292V was present in 3.8% of newborns with respiratory distress syndrome, suggesting it imparts an increased genetic risk for this syndrome.
Clinical Presentation
Autoimmune PAP presents as progressive dyspnea of insidious onset unless secondary infection is also present, in which case fever, cough, and rarely hemoptysis may also be present. The frequency of various symptoms was recently evaluated in a large contemporaneous cohort in Japan ( Table 70-5 ). This nonspecific presentation may lead to months or years of misdiagnosis as “asthma” or “chronic bronchitis.” The symptoms are frequently milder than expected from the radiographic findings, which should raise the suspicion of PAP. The physical examination is nonspecific and is frequently normal despite a grossly abnormal chest radiograph but may include basilar crackles. Cyanosis is seen in severe cases; hemoptysis and fever are rare. Clubbing is not a feature of autoimmune PAP or of hereditary PAP. In the Japanese national PAP registry cohort, 31% of patients with marked radiologic manifestations were asymptomatic.
Symptom | Frequency (%) |
---|---|
Dyspnea | 54 |
Cough | 23 |
Sputum production | 4 |
Other | 4 |
None (asymptomatic) | 31 |
* Data for a contemporaneous group composed of 220 patients.
Hereditary PAP caused by mutations in GM-CSF receptor genes ( CSF2RA or B ) presents as insidious, progressive, dyspnea-like autoimmune PAP, usually in children. Thus, whereas this disease is newly described, rare, and necessarily less well studied, it appears similar to autoimmune PAP, except that it is a disease of children instead of adults.
Secondary PAP usually arises in an individual with an underlying systemic disorder such as a hematologic malignancy/disease. It can be seen in the context of neutropenia or myeloid cell dysfunction related to the underlying disorder and can resolve with successful treatment of the underlying disorder.
PSMD disorders can present as a spectrum of lung disease ranging from respiratory distress syndrome in neonates and infants to interstitial lung disease in children, adolescents, and adults, depending on the exact nature of the underlying genetic mutations. SP-B deficiency typically presents as respiratory distress immediately after birth. ABCA3 mutations can result in fatal respiratory distress syndrome in neonates and interstitial lung disease in infants, children, and adolescents. SP-C mutations can present as interstitial lung disease in children and adults.
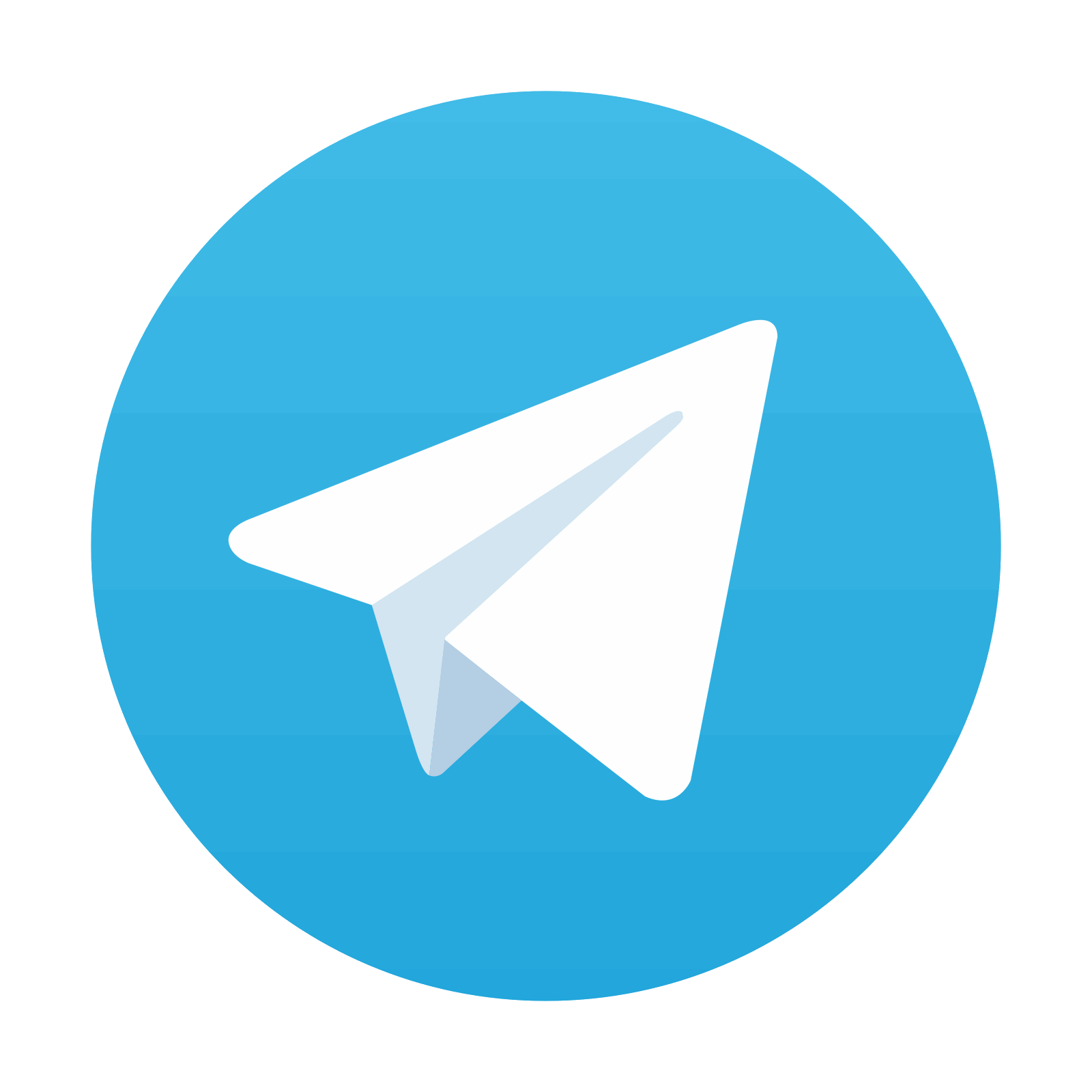
Stay updated, free articles. Join our Telegram channel

Full access? Get Clinical Tree
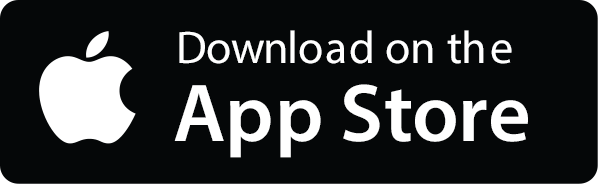
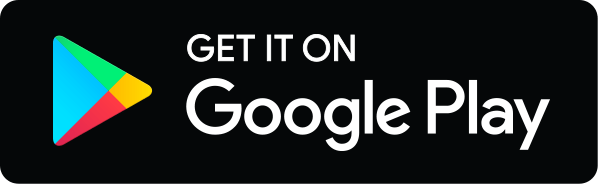