Programmability and Specialized Circuits
Permanent Pacemaker Programmability
Programmability often allows for noninvasive correction of pacemaker malfunction, optimizing battery life and adjustment of the pacemaker to the patient’s physiologic needs, which may change over time. Before external programming is performed, any problems must be appropriately diagnosed and the clinical status of the patient, especially the degree of the patient’s pacemaker dependency and the safety margin left after programming, must be considered.
Methods of External Programming
Early in the development of permanent pacemakers, the advantage of being able to change the rate of firing of the generator became obvious. One of the earliest efforts involved a screw on the generator that, when turned, would change the pacing rate. This method required making a skin incision and using a sterile screwdriver. Advances in technology now allow noninvasive programming of several pacemaker functions, including changing the pacing rate. To program a pacemaker externally, a signal, usually pulsed magnetic fields or a radiofrequency signal, is sent through the patient’s skin and received by the generator. If a pulsed magnetic field is transmitted, it can influence a piece of metal called a reed switch to complete an electrical circuit. The pacemaker must be protected against accidental or phantom programming resulting from bombardment of the pacemaker by environmental electromagnetic waves. Usually this protection requires that a very specific code be sensed by the pacemaker before it will respond to programming.
Rate Programmability
The pacemaker function most commonly programmed is rate. A typical programmable range is 30 to 120 beats per minute (bpm) and most pacemakers are
preset by the manufacturer at approximately 60 to 70 bpm. Many studies have attempted to determine the pacemaker rate that optimizes cardiac output and, in general, it has been found that cardiac output varies little over a fairly wide range of paced rates, even when an atrial pacemaker is used. The increase in cardiac output that occurs normally with exercise involves not only an increase in heart rate but also peripheral vasodilatation in areas of increased metabolic activity and an increase in cardiac contractility. Simply increasing the rate of the pacemaker does not duplicate these complex physiologic events. In some individual patients and in some clinical situations, however, an increase in pacemaker rate does increase cardiac output. For example, a pacemaker-dependent patient who is metabolically unstable after surgery may benefit from a faster-paced rate. If the clinical situation is critical, this can even be determined by use of thermodilution cardiac outputs. Rate programmability allows for flexibility in these instances. Another reason for increasing rate is to overdrive cardiac arrhythmias.
preset by the manufacturer at approximately 60 to 70 bpm. Many studies have attempted to determine the pacemaker rate that optimizes cardiac output and, in general, it has been found that cardiac output varies little over a fairly wide range of paced rates, even when an atrial pacemaker is used. The increase in cardiac output that occurs normally with exercise involves not only an increase in heart rate but also peripheral vasodilatation in areas of increased metabolic activity and an increase in cardiac contractility. Simply increasing the rate of the pacemaker does not duplicate these complex physiologic events. In some individual patients and in some clinical situations, however, an increase in pacemaker rate does increase cardiac output. For example, a pacemaker-dependent patient who is metabolically unstable after surgery may benefit from a faster-paced rate. If the clinical situation is critical, this can even be determined by use of thermodilution cardiac outputs. Rate programmability allows for flexibility in these instances. Another reason for increasing rate is to overdrive cardiac arrhythmias.
Pacemaker rate is decreased most commonly to allow the emergence of the patient’s spontaneous rhythm. Many patients with sinus bradycardia feel better with the atrial “kick” that increases cardiac output, rather than with a ventricularly paced rhythm at a faster rate. In addition, this slowing of the paced rate preserves battery life. Decreasing the paced rate is used also to determine the patient’s underlying rhythm or to observe the patient’s intrinsic electrocardiogram (ECG) to evaluate a possible myocardial infarction or assess drug effects on ECG intervals.
Recent evidence raises the possibility of a deleterious effect of chronic right ventricular pacing. Since many patients have a pacemaker only for intermittent bradyarrhythmias, then reducing the rate may allow contraction of the left ventricle through the normal His-Purkinje system. This potentially beneficial effect can also, sometimes, be accomplished by lengthening the AV interval in a dual-chamber pacemaker. This information comes from studies that do not necessarily apply to all patients and clearly right ventricular pacing is appropriately necessary in patients with complete heart block, for example. One theory is that the disordered contraction through the myocardium from the RV apex to the base of the heart, bypassing the His-Purkinje system, leads to a chronic myocardial dysfunction. Investigations are under way as to whether selective lead placement, for instance in the right ventricular outflow tract or direct pacing of the His-Purkinje system would eliminate this effect.
Table 4-1 summarizes the uses of rate programmability.
Pulse-Width Programmability
As discussed in Chapter 3, the width or duration of the pacemaker spike is of considerable clinical importance, even though it cannot be measured on a standard 12-lead ECG. The exact time duration of the pacemaker impulse is measured on the standard pacing system analyzer.
Widening the pulse width improves the ability of the pacemaker to stimulate the heart, but as predicted by the strength–duration curve, this
improvement is only a modest one at greater widths. An example of widening the pulse width to allow capture of the heart rate is shown in Figure 4-1. At the time of pacemaker implantation, the patient had a good acute threshold of 0.6 V when the pulse width was 0.6 msec; however, after 4 weeks, the threshold rose to approximately 5 V with a pulse width of 0.6 msec, and the patient was intermittently losing capture. Slight widening of the pulse width caused consistent capture. To allow a reasonable safety factor, the pulse width in the example was left at 1.8 msec.
improvement is only a modest one at greater widths. An example of widening the pulse width to allow capture of the heart rate is shown in Figure 4-1. At the time of pacemaker implantation, the patient had a good acute threshold of 0.6 V when the pulse width was 0.6 msec; however, after 4 weeks, the threshold rose to approximately 5 V with a pulse width of 0.6 msec, and the patient was intermittently losing capture. Slight widening of the pulse width caused consistent capture. To allow a reasonable safety factor, the pulse width in the example was left at 1.8 msec.
TABLE 4-1 Uses of rate programmability | |||||||
---|---|---|---|---|---|---|---|
|
We are using an example in which capture is improved, but often the strength–duration curve is so flat beyond 1.5 msec that a widened pulse width may not improve capture safely and effectively. Also, we are assuming that no other evidence of pacemaker malfunction, such as electrode displacement, is present and that the patient is not so pacemaker dependent that any further threshold rise causing transient loss of capture would be a risk to the patient. Another approach to the problem would be to raise the voltage of the pacemaker above 5 V, but this is not technically possible with all pacing devices.
If the clinician believes that widening the pulse width is reasonably safe in such a situation, then doing so may save the patient a surgical procedure; in fact, the threshold may improve with time. The tradeoff with the widened pulse width is a shorter battery life, but with the long-lived lithium batteries, battery life often is not a major consideration. The relationship between pulse width and battery depletion is not linear: Doubling the pulse width shortens battery life by less than half. This happens because the first half of the pacemaker spike generates more current than the second half (see Fig. 3-3 in Chapter 3).
Another use of programming pulse width is to preserve battery life. In the example depicted in Figure 4-2, a patient has a low chronic threshold of 5 V at 0.1 msec pulse width. Because the battery is fixed at 5 V, energy is wasted. By narrowing the pulse width from the usual 0.5 msec to 0.3 msec, energy is preserved (i.e., fewer electrons are expended per pacing spike, and therefore battery life is prolonged) and a reasonable safety margin still exists. The patient still should be monitored periodically to ensure that the threshold is not rising. The current approach to preserving battery life in this situation is to lower voltage (although voltage cannot be raised easily above 5 V, it can be lowered easily in most pacemakers). Narrowing the pulse width is left in this text as a teaching point.
Programming pulse width can be used to estimate threshold. Figures 4-1 and 4-2 are drawn as if we knew the shape of the strength–duration curve in both patients; this is done for the sake of explaining concepts. In practice, however, the exact shape of the strength–duration curve in an individual patient often is unknown. Therefore, we can obtain an estimate of the threshold as determined by pulse width at a fixed voltage (i.e., one point on the
strength–duration curve) by narrowing pulse width until capture is lost. This information is sometimes clinically useful. By programming voltage, additional points on the strength–duration curve can be obtained.
strength–duration curve) by narrowing pulse width until capture is lost. This information is sometimes clinically useful. By programming voltage, additional points on the strength–duration curve can be obtained.
TABLE 4-2 Uses of pulse width programmability | |||||
---|---|---|---|---|---|
|
Narrowing pulse width also can be used to reduce annoying electric complications of pacing, such as pectoral muscle twitching or diaphragmatic stimulation, that occur in an occasional patient, although this approach is often not successful; lowering voltage is a better approach. Before programming is done, the pacing system should be checked to ensure that no malfunction requiring another method of correction is causing the difficulty. Table 4-2 summarizes the use of pulse width programmability.
Voltage Programmability
Modern pacemakers can be programmed only to lower settings below their maximum of 5 to 7 volts. A high voltage threshold suggesting a need for a higher voltage indicates a very poor threshold that needs attention such as new lead. Increasing voltage with a chronically poor threshold would maintain capture in a patient, but at the expense of rapid battery depletion. High-voltage units consisting of two lithium iodine batteries in series have been available in the past, but are rarely, if ever, used now.
Being able to lower the voltage setting by programming allows conservation of battery life, as demonstrated in Figure 4-3. In this example, the patient has a low chronic threshold of 1.3 V at 0.6 msec pulse width. By leaving the voltage at 2.5 V and pulse width at 0.6 msec, fewer electrons are used per paced beat and battery life is preserved. It should be emphasized that this approach is useful only if the chronic threshold is low and if battery life is a significant clinical consideration in the patient. Another approach to this patient would be to narrow pulse width, but within a range of pulse width of about 0.4 to 0.6 msec, voltage drop would be the more appropriate approach.
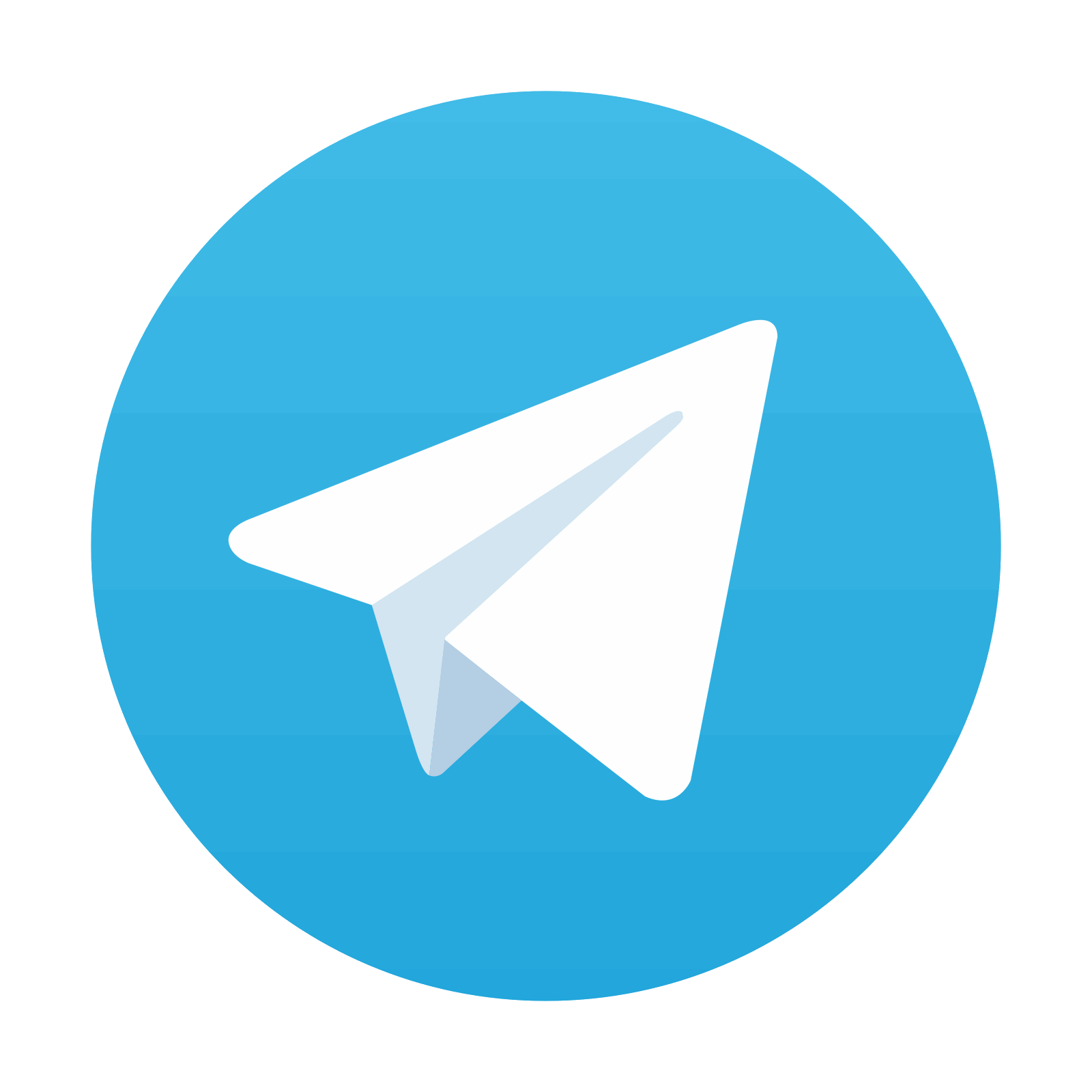
Stay updated, free articles. Join our Telegram channel

Full access? Get Clinical Tree
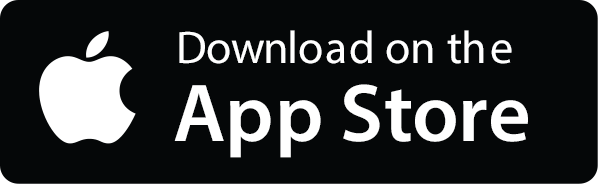
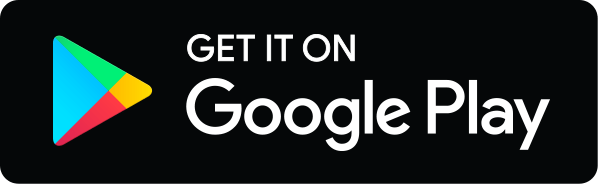