Indications for consideration of rib plating
Flail chest
1. Three or more unilateral segmental rib fractures
2. Three or more bilateral rib fractures
3. Three or more unilateral rib fractures combined with sternal fracture/dissociation
Severe deformity of chest wall
1. 100 % displacement of three or more ribs
2. Marked loss of thoracic volume/caved in chest (>25 % volume loss)
3. Overriding of three or more ribs (>15 mm each)
4. Displaced rib fractures associated with intraparenchymal injury
In general, the goal of surgery for a patient with a flail chest is to stabilize the flail segment so that paradoxical chest motion is eliminated. Stabilization of a certain minimum number of ribs and fracture sites is required to provide adequate stability to each particular chest injury. Unfortunately, clear guidelines from the literature do not exist to determine this number. Intraoperatively, I will assess the stability of the chest wall after each rib fracture is stabilized with simple manual pressure on the flail segment. If the previously unstable segment is moving as a unit with the rest of the chest wall, then we can consider the goal of surgery fulfilled. A common scenario results when the flail segment is badly displaced at one site, while hinged at the second fracture site. Stabilization of only the displaced fracture is almost always sufficient to provide stability to the segment, since the hinged fracture is locally stabilized by soft tissues which were not disrupted by the large initial displacement. I will generally stabilize a minimum of three ribs since this number is readily accessed through a single incision. With each subsequent rib fracture that is stabilized, there is less motion across the fractures and reduced stress borne by the plate. Stabilization of an inadequate number of ribs may result in lack of clinical improvement from persistent instability of the flail segment and possibly failure of the implants.
General Principles of Bone Healing
When a bone is fractured, several biologic processes are brought into action as the body attempts to stabilize the injured skeleton. Bone healing occurs through two distinct biologic mechanisms known as primary bone healing and secondary bone healing [1].
Primary bone healing uses the histologic unit of the cutting cone with osteoclasts moving directly across the fracture, to remove dead bone, after which new bone is directly laid down by osteoblasts. This biologic mechanism requires direct contact between the bone cells and a mechanically rigid environment (absolute stability) [2]. Fractures in many parts of the appendicular skeleton are typically treated with techniques that optimize primary bone healing such as lag screw application and compression plating. These techniques rely on an anatomic reduction (perfect apposition of the injured bone ends) and absolute stability with compression across fracture surfaces. Application of these absolute stability techniques to the treatment of rib fractures, however, is impractical and not recommended. Ribs are small, rather soft bones which are prone to relatively transverse fractures with fragmentation at the fracture site. As a result, perfect reduction and absolute stability are often technically impossible. The constant movement of the thoracic cage during respiration further complicates the ability to achieve absolute stability across a rib fracture.
Secondary bone healing is the other major biologic bone healing strategy available to mend fractured bones. This is the biologic strategy at work during the routine nonoperative healing of the injured skeleton, including rib fractures. Secondary bone healing proceeds in three phases. The first is the inflammatory phase which forms a fracture hematoma. This stops ongoing bleeding and recruits inflammatory and osteogenic cells to the injured area via immune mechanisms. Following the initial inflammatory phase, the reparative phase modifies the fracture hematoma into soft callus which is composed of woven bone and provides some initial stability. The third phase is known as remodeling where the soft callus is remodeled into lamellar bone with resulting hard callus. This provides a durable structural repair to the injured bone. The time course of this process varies, but, in general, the inflammatory phase occurs over the first 2–3 weeks following injury; the reparative phase predominantly from 2 to 8 weeks; and the remodeling phase for 8 weeks to years following the injury. The injured bone remodels in response to the forces that influence it (Wolf’s law); however, there will almost always be a permanent enlargement of hard callus noticeable following repair by secondary bone healing.
Successful bone healing requires a sufficient blood supply and a stable biomechanical environment. For callus to form, strain (a measure of stability) needs to be less than 10 % [3]. When a fracture has insufficient stability, the strain on the tissues will be too great to form bony callus. As a result, weak fibrous tissue forms between the bone ends rather than bridging callus and results in a nonunion. This occurs in the context of rib fractures when a fractured rib or multiple rib segments remain mobile and fail to heal. Nonunion is seen in flail chest patients and is often associated with pain, disability, and deformity. As a result of these issues, surgical stabilization of chest trauma is gaining renewed attention in recent years. Figure 9.1 demonstrates radiographs and CT scan of a patient with persistent, severe pain with chest wall deformity and nonunion of multiple rib fractures following a flail chest injury who was successfully treated with correction of deformity and plate fixation.
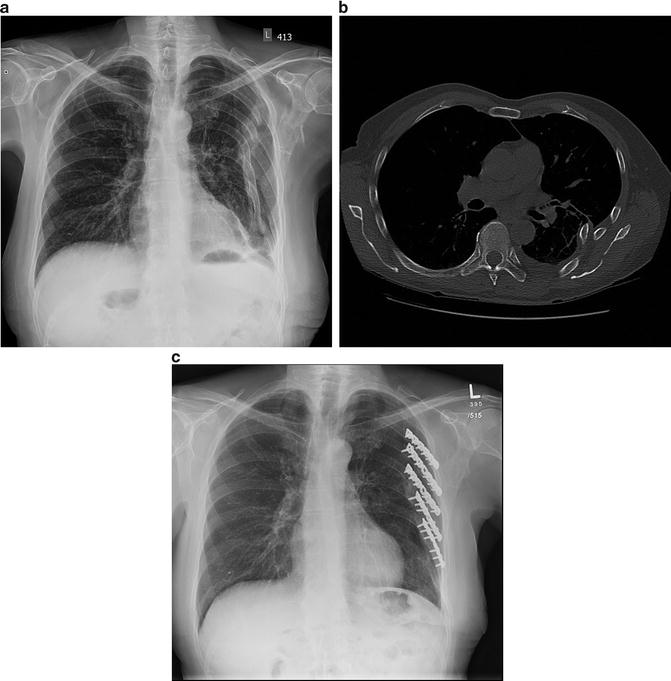
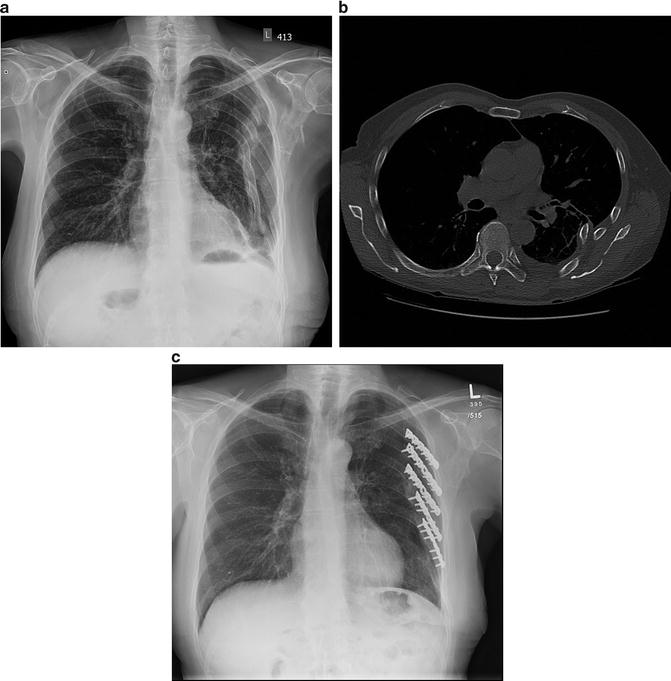
Fig. 9.1
(a) A patient sustained multiple rib fractures with a flail segment and deformity. After nonoperative treatment, persistent pain, deformity, and instability resulted. Radiographs (a) and a CT scan (b) demonstrated rib nonunions. The patient was treated successfully with open reduction, correction of deformity, and plate fixation with conventional 3.5 mm pelvic reconstruction plates (c)
Methods to Achieve Mechanical Stability of Fractures
Basic principles of treatment for fractured bones include realignment of the bone (reduction) and then maintenance of the reduced position. In the extremities, splints, functional braces, and cast immobilization can be used to maintain limb stability in a reduced position without operative stabilization. These external devices, however, are very challenging to use effectively in the chest wall. No splinting method has been shown to have any utility for multiple rib fracture patients.
Nonoperative treatment has long been the standard treatment of rib fractures and remains the most common method of treatment for traumatic chest injury [4]. When using this treatment strategy for chest wall fractures, splinting from neighboring ribs and/or the resilience of muscles and other soft tissues is anticipated to provide adequate stability for bony rib healing. Positive pressure ventilation can also be utilized in the hopes of providing an internal splint to the chest wall [5].
Surgical stabilization options include external or internal fixation. External fixation involves the insertion of pins into the bones with spanning, external connecting rings, or rods to provide support to the injured bone. This is not practical for injuries to the chest wall. Internal fixation involves stabilizing the injured bone with devices placed directly through an open surgical exposure. Pins, plates/screws, and intramedullary implants are the primary options available. Smooth pins have a distinct disadvantage in that they can migrate out of the bone potentially injuring intrathoracic structures [6–8]. Unsecured pins have no role in the modern surgical management of rib fractures. Intramedullary implants have been used successfully in long bones such as the femur and tibia. The rib has an intramedullary canal, and implants have been designed to act as an internal splint. To prevent migration, these devices have a screw and tab to prevent hardware migration [9] (Fig. 9.2).
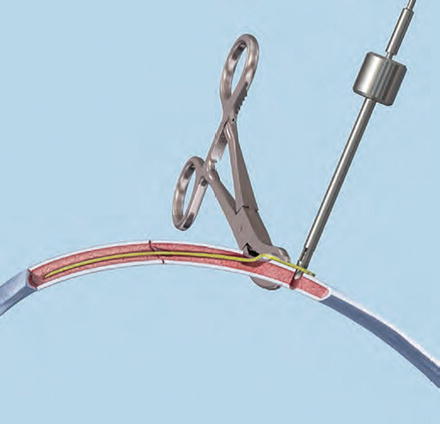
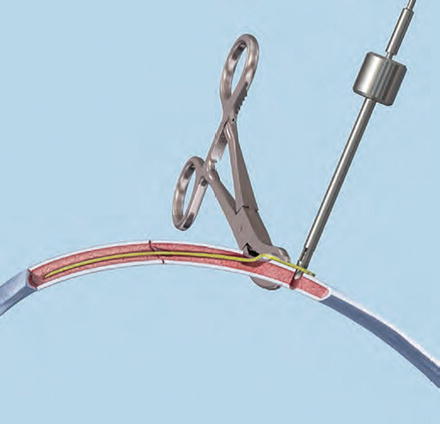
Fig. 9.2
The Matrix IM rib splint highlighting the set screw designed to prevent migration of the implant, as can be seen with smooth intramedullary pins or wires
Many options for stabilizing injured ribs have been described. However, internal fixation with plating is the most widespread option currently in use to surgically stabilize rib fractures. For the majority of rib fractures requiring surgical stabilization, plates provide the optimal implant to simultaneously provide stability and maintain reduction of the injured rib(s).
Plating System Options
Stabilizing plates have been intermittently used to treat fractured bones as early as the turn of the twentieth century. However, plate fixation of fractures was not popularized until the second half of the twentieth century through the pioneering work of the AO group [10]. Surgical stabilization of ribs with plates was described as early as 1961 [11]; however, early plates differed significantly from modern designs and never gained popularity, probably due to technical limitations which resulted in poor clinical outcomes.
The two main categories of plates that are currently in use today include conventional plates and locked plates. Conventional plates resemble the original plates of the 1960s and have smooth holes cut out to receive smoothed, rounded screw heads (Fig. 9.3). These plates rely on the associated screws to purchase into the underlying bone thus abutting the plate to the bone via friction (Fig. 9.4). Application of multiple screws into the plate splints the injured bone, providing stability to allow successful bone healing in the desired position.
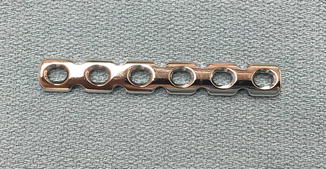
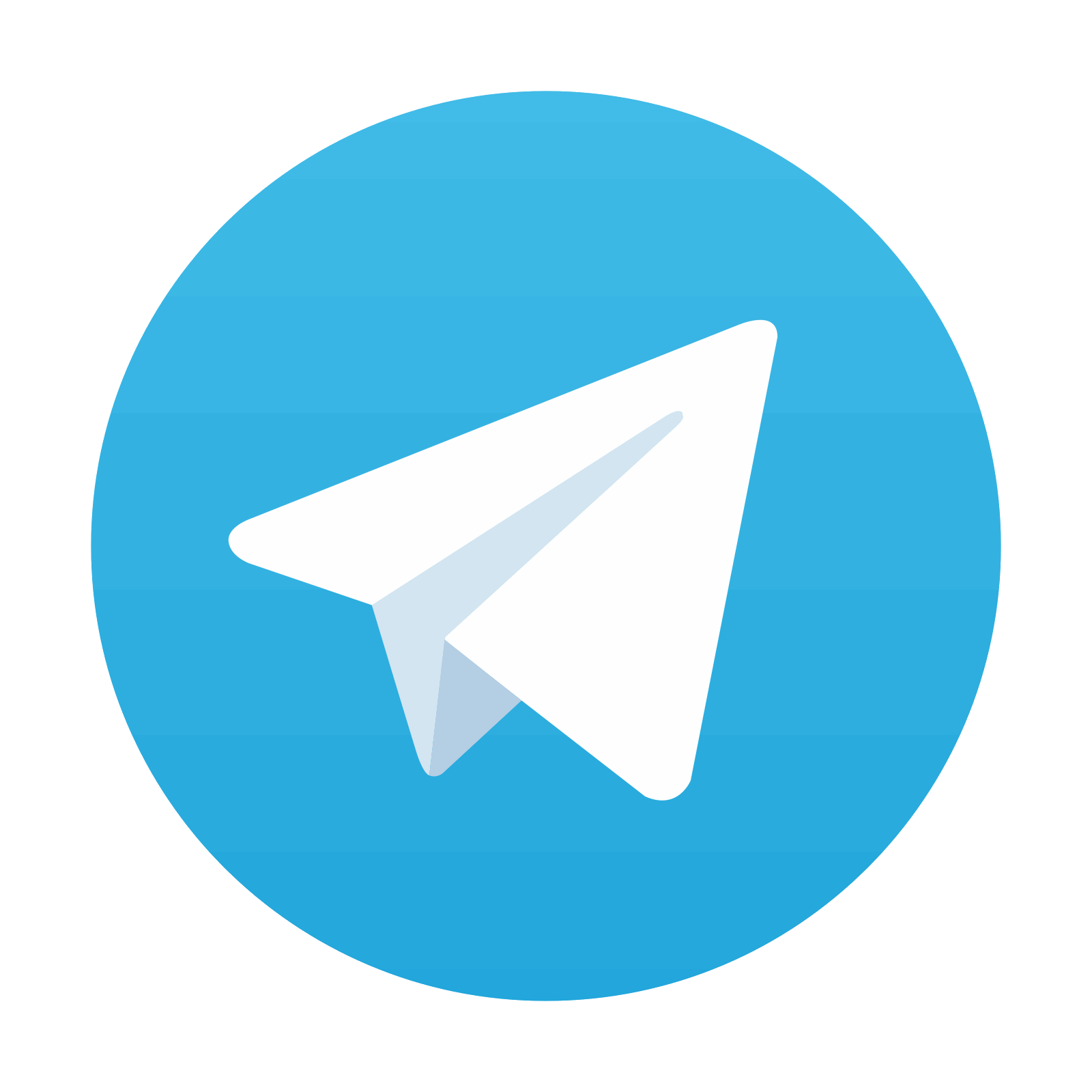
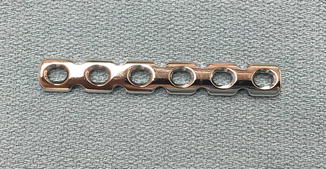
Fig. 9.3
A conventional 3.5 mm pelvic reconstruction plate. Note the smooth holes for screw insertion and the notches cut into the side of the plate to facilitate contouring in multiple planes
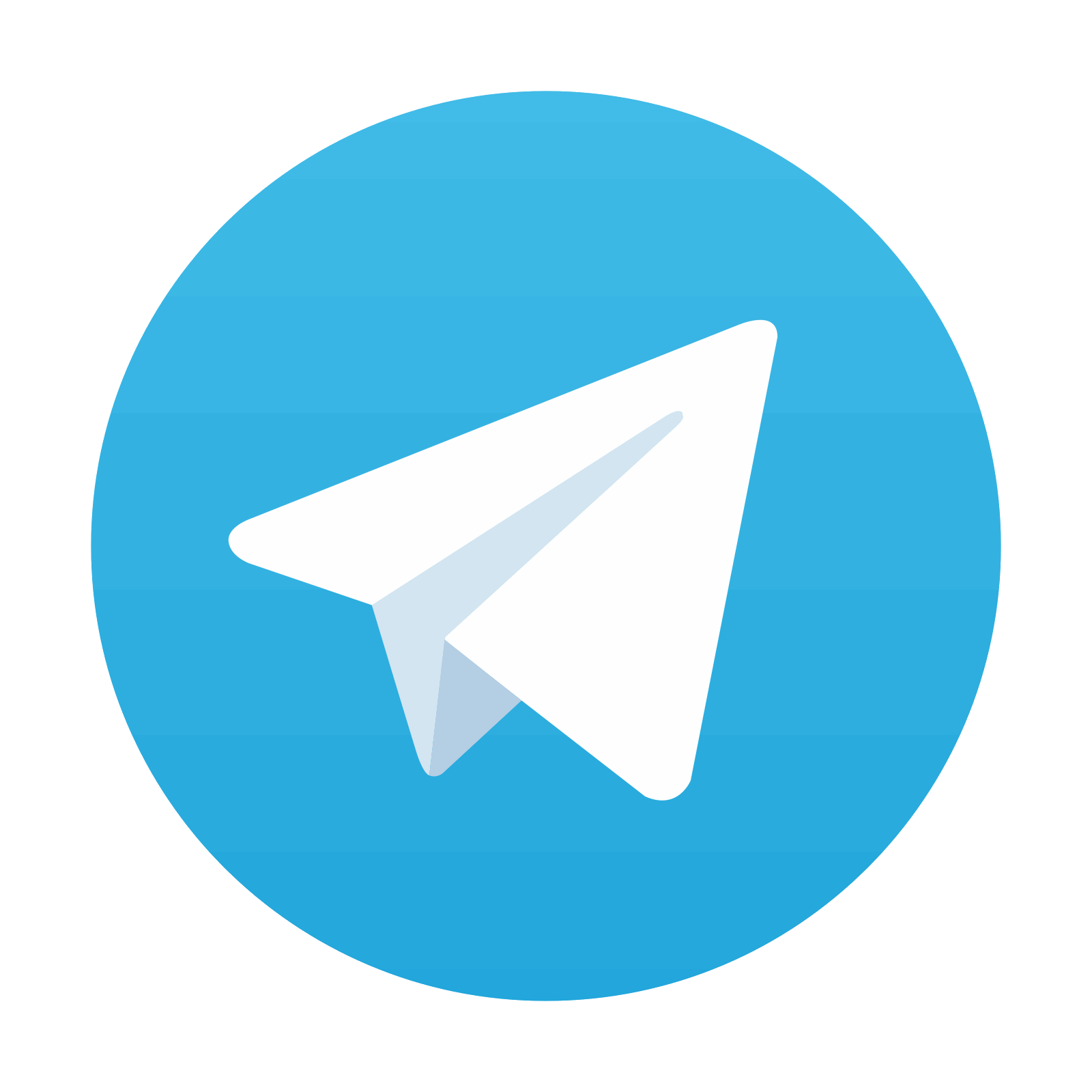
Stay updated, free articles. Join our Telegram channel

Full access? Get Clinical Tree
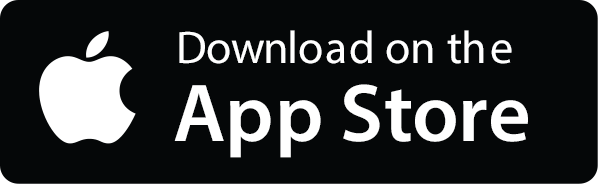
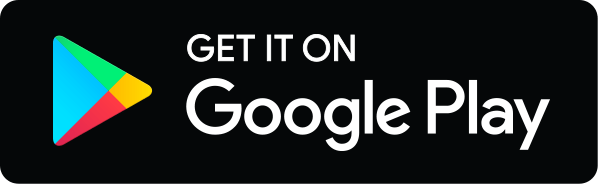