Introduction
Pneumomediastinum and mediastinitis are disorders that involve the potential spaces and tissues of the mediastinum. Although both may be present at the same time, for example, after esophageal rupture, the term pneumomediastinum usually refers to the presence of aberrant air in the mediastinum without accompanying infection and mediastinitis refers to infection or inflammation regardless of the presence of air. These conditions are seen in a variety of unique clinical circumstances. Both tend to result from either breaches in the integrity of mediastinal structures or the spread to mediastinal structures from elsewhere in the body. Thus, anatomic considerations are similar for the two processes. However, the pathophysiology, clinical settings, management approaches, and clinical impact of pneumomediastinum and mediastinitis are quite different and are discussed separately.
General Anatomic Considerations
The mediastinum is traversed by the trachea and the esophagus, which are air-filled structures in direct communication with the outside world and may contain colonizing microorganisms. In addition, mediastinal lymph nodes drain the airways and lung parenchyma where inhaled organisms, antigens, and dusts are encountered. Consequently, entry of air or inflammatory and infectious materials into the mediastinum is readily accomplished by breaches in the integrity of these mediastinal structures or when materials deposited in the airways or distal lung are transported through the lymphatic system.
How air enters and moves through the mediastinum can be understood by referring to the soft tissue compartments of the neck, thorax, and abdomen, as illustrated in Figure 84-1 . The visceral space surrounding the trachea, esophagus, and great vessels in the neck continues into the chest to envelop the mediastinal viscera and passes through the diaphragm with the esophagus to communicate with the retroperitoneal space. This same space extends along the vessels and airways of the pulmonary hilum to merge with the distal bronchovascular sheaths (see Fig. 84-1 ). Thus, it is possible for air or inflammation that reaches these tissue planes to dissect and spread to involve any of the mediastinal structures.

Pneumomediastinum
Pneumomediastinum consists of air or other gas in the mediastinum. Also known as mediastinal emphysema , it is one of several forms of aberrant extra-alveolar air that include subcutaneous emphysema, pulmonary interstitial emphysema, pneumopericardium, pneumoperitoneum, pneumoretroperitoneum, pneumocephalus, and pneumorachis (air in the spinal canal). Pneumothorax, the most common life-threatening form of extra-alveolar air, is discussed elsewhere (see Chapter 81 ).
Pneumomediastinum and subcutaneous emphysema were first recognized during childbirth. In her “Observations” of 1617 (as cited by Gordon ), Louise Bourgeois, midwife to the queen of France, wrote, “I saw that she tried to stop crying out, and I implored her not to stop, for fear that her throat might swell.” The first formal medical documentation of pneumomediastinum and subcutaneous emphysema during labor was by Simmons in 1784, and this association was reported in more than 130 patients by 1927. Over the next 2 decades, Hamman had thoroughly established the clinical features of spontaneous pneumomediastinum and Macklin and Macklin had elegantly elucidated its pathophysiology. Little has been added to the pioneering descriptions of these investigators, but pneumomediastinum and other forms of extra-alveolar air are seen today in a greater variety of clinical circumstances. These include mechanical ventilation and other situations in critical care, decompression injury diving, chest trauma, and asthma.
Pathophysiology
The pathophysiology of pneumomediastinum depends on the clinical circumstances in which it is encountered. Pneumomediastinum most commonly results from alveolar rupture but can also result from air escaping from the upper respiratory tract, intrathoracic airways, or gastrointestinal tract. Gas can be generated by certain infections, and outside air can reach the mediastinum after trauma or surgery. These potential sources for mediastinal emphysema are summarized in Table 84-1 and discussed in the following sections.
UPPER RESPIRATORY TRACT | LUNG PARENCHYMA | EXTERNAL TO THE BODY |
Head and neck infection (odontogenic, salivary glands, cervical adenitis, tonsillitis, peritonsillar abscess, osteomyelitis of facial bones) | Direct disruption of alveoli (penetrating trauma, surgery, transbronchial biopsy, needle aspiration) | Penetrating trauma to neck or chest Surgical procedures (tracheotomy, mediastinoscopy, sternotomy) |
Fractures (involving paranasal sinuses, orbit, mandibles, other facial bones) | “Spontaneous” alveolar rupture (between alveolus and adjacent bronchovascular bundle) | Via subcutaneous emphysema in association with chest tube insertion |
Other mucosal disruption (trauma, surgery, attempted endotracheal intubation) | GASTROINTESTINAL TRACT | INFECTION WITH GAS-PRODUCING ORGANISMS |
Esophageal perforation Via pneumoperitoneum or pneumoretroperitoneum (gastric or intestinal perforation, diverticulitis, pneumatosis cystoides intestinalis, endoscopy, biopsy, infection) | ||
Dental procedures (extractions, air-turbine drilling) | Acute bacterial mediastinitis Head and neck infections | |
INTRATHORACIC AIRWAYS | ||
Blunt or penetrating chest trauma Foreign body | ||
Iatrogenic (bronchoscopy, bronchial brushing, transbronchial biopsy, needle aspiration) | ||
Neoplasm |
Air Originating from the Upper Respiratory Tract
Mediastinal air dissecting downward from the head and neck can originate in several ways. Retropharyngeal abscess is a long-established cause of gas in the neck, but other infections including dental abscess, cervical adenitis, salivary gland infection, tonsillitis, and osteomyelitis of the facial bones can also produce this finding. Odontogenic infections are said to be the most common of these.
Dental extractions or drilling can be followed by pneumomediastinum. Passage of air into the soft tissues from the socket of an extracted tooth can be increased dramatically if positive pressure is applied at the mouth—as illustrated by the case of a soldier whose neck swelled on resumption of his duties as a bugler immediately after a tooth extraction.
Injury to the paranasal sinuses, orbit, mandible, and other facial bones adjacent to the upper airways can provide access of air to the fascial planes of the neck, especially following nose blowing. Surgical procedures involving the upper respiratory tract may produce air in the neck via breaches in either the oropharyngeal mucosa or the trachea. For instance, pneumomediastinum and subcutaneous emphysema are common after tracheotomy; these findings were noted in 13% of cases in one prospective series in adults.
Pneumomediastinum can also arise following traumatic attempts at endotracheal intubation or after overinflation of the endotracheal tube cuff when the hypopharyngeal mucosa or membranous trachea is injured ( eFig. 84-1 ). Tracheal injury and esophageal rupture leading to pneumomediastinum can also happen when air is forced into the open mouth.
Air Originating from the Intrathoracic Airways
Blunt thoracic trauma, particularly deceleration injury as sustained in motor vehicle crashes, may lacerate or fracture the trachea or main bronchi and allow air to enter the mediastinum ( eFig. 84-2 and ; see eFig. 76-10 and ). Although the trachea may be damaged proximally, the majority of such injuries are within 3 cm of the carina, probably owing to the relative fixation of the airway at the carina and the development of a shearing force when the more mobile parts are displaced by the sudden impact.
Other potential sources of mediastinal air from the major intrathoracic airways include perforation by an aspirated foreign body and erosion by bronchogenic or esophageal neoplasm. Visceral perforation is associated more frequently with esophagoscopy than with bronchoscopy. However, the increasing use of bronchoscopic biopsy techniques and therapies for bronchial obstruction render this source for mediastinal air increasingly likely.
Air Originating in the Lung Parenchyma
Most instances of pneumomediastinum are the result of alveolar disruption. Alveoli may be disrupted after direct injury to the lung parenchyma, which allows air to escape from torn alveoli and terminal bronchioles, after surgery, when air can escape from cut surfaces, or when the lung is nicked in the course of central venous access procedures, or percutaneous or transbronchial lung biopsy. The majority of cases of alveolar disruption leading to pneumomediastinum, however, is from “spontaneous” alveolar rupture.
Mechanism of “Spontaneous” Alveolar Rupture.
Disruption of alveolar walls and entry of air into the bronchovascular bundle can result when a pressure gradient becomes sufficient to disrupt the alveolar walls at their bases and force air into the pulmonary interstitium, as depicted in Figure 84-2 . Although it has been speculated that an increase in intra-alveolar pressure alone was capable of producing alveolar rupture, animal experiments have demonstrated that an increase in alveolar volume, due to increased transpulmonary pressure, is a more important determinant of disruption of the alveolar walls. This finding may explain the rarity of alveolar rupture following coughing and sneezing; contraction of muscles of the chest and abdominal walls counter the transient high intra-alveolar pressures, and transpulmonary pressures and alveolar volume do not increase.

With decompression, when there are decreases in external pressure, high alveolar volumes undoubtedly contribute to alveolar rupture. The mechanism of alveolar rupture presumably relates to overdistention due to the expansion of gas trapped in the lungs during ascent.
Underlying disease of the lungs is probably also important. Pneumomediastinum and other forms of extra-alveolar air arising from the lung are almost always associated with parenchymal abnormalities in the affected lung both during mechanical ventilation and during spontaneous ventilation.
Spread of Air following Alveolar Rupture.
Entry of air into the bronchovascular bundle, as depicted schematically in Figure 84-2 , produces pulmonary interstitial emphysema ( eFig. 84-3 ). This is the initial consequence of alveolar rupture and may be the only overt manifestation of extra-alveolar air. According to Munsell, Laennec called this condition “interlobular emphysema.” Because the mean pressure in the mediastinum, like that in the pleural space, is always somewhat negative with respect to the pressure in the pulmonary parenchyma, the pressure difference causes air to move toward the mediastinum. This is illustrated in Figure 84-3 and convincingly described in the 1944 paper by Macklin and Macklin, which first confirmed the sequence in experimental animals:

The first step … was air passing through numerous minute ruptures in the strained bases of the alveoli of the overinflated region into the underlying vascular sheaths. The air bubbles, at the very first minute, moved along the vascular sheaths, coalescing and gaining in size. This streaming of air through the pulmonic interstitium reminded one of the flow of a river that ever increases in size by addition of new tributaries as it proceeds on its course. Reaching the root of the lung, the train of air bubbles passed into and distended the mediastinum. With continued insufflation an actual overflow into the retroperitoneum, anterior mediastinum and subcutaneous tissues of the root of the neck and axillae occurred. In extreme cases the mediastinal wall ruptured, producing a pneumothorax.
These classic observations have since been confirmed in more recent studies but, conceptually, little has been added.
Entry of Air into the Pleural Space.
Once in the mediastinum, air follows the path of least resistance and may rupture through the delicate mediastinal fascia and overlying pleura into the pleural space. This model is supported by observations that, in animal experiments, lower sustained inflation-hold pressures were required to produce pulmonary interstitial emphysema than to create a pneumomediastinum or pneumothorax and that, in most mechanically ventilated patients in one clinical series, pneumomediastinum preceded pneumothorax. Another proposed mechanism for pneumothorax suggests that air dissects toward the periphery of the lung rather than toward the mediastinum and ruptures via subpleural blebs through the visceral surface of the lung. Which of these mechanisms most often accounts for “spontaneous” pneumothorax is uncertain.
Entry of Air into the Abdomen.
Extra-alveolar air originating from disrupted alveoli may appear below the diaphragm as either pneumoperitoneum or pneumoretroperitoneum ( eFig. 84-4 ). To reach the retroperitoneal space, air from the mediastinum usually travels via the loosely packed paraesophageal connective tissue across the diaphragm. The retroperitoneal air may then break through the peritoneum to enter the peritoneal space.
Air Originating in the Gastrointestinal Tract
Mediastinal air can also originate from the gastrointestinal tract, either above or below the diaphragm. In Boerhaave syndrome , rupture of the esophagus allows air and other material to enter the mediastinum ( eFig. 84-5 ). In this circumstance, pneumomediastinum is accompanied by acute mediastinitis, as discussed later in this chapter. Pneumomediastinum can also result from esophageal perforation during upper gastrointestinal endoscopy or following the ingestion of a caustic such as paraquat or lye (sodium hydroxide). Spontaneous pneumomediastinum is a rare presentation of achalasia.
Rarely, air or other gas originating in the retroperitoneal space can spread to the mediastinum. Retroperitoneal causes of pneumomediastinum include perforation of a duodenal ulcer, ulcerative colitis, colonic diverticulitis (see eFig. 84-4 ), pneumatosis cystoides intestinalis, “rectal barotrauma” and procedures such as sigmoidoscopy, colonoscopy, and barium enema.
Air Originating from External and Miscellaneous Sources
Pneumomediastinum can arise from air entering the body from external sources, particularly if positive pressure is applied at the subcutaneous tissue planes. Air can reach the mediastinum via the cervical soft tissues during tracheotomy, via the chest wall during arthroscopic shoulder surgery, or via the extremities as a result of industrial accidents. Mediastinal air can also arise from a pneumothorax by an unusual mechanism: air in the pleural space can reach the subcutaneous tissues via a tube thoracostomy tract, can dissect to the neck, and can then penetrate down into the mediastinum. Pneumomediastinum has also been observed following the insufflation of air into the peritoneal cavity for tuberculosis treatment, during the course of modern era laparoscopy, and via the female genital tract during pelvic examination, douche, postpartum exercise, or blowing into the vagina, especially during pregnancy.
Gas can form in the mediastinum during acute bacterial mediastinitis caused by gas-producing microbes. More commonly, however, pneumomediastinum associated with mediastinitis is due to communication with the gastrointestinal tract (as in Boerhaave syndrome), respiratory tract (as in necrotizing pneumonia), or outside air (as in traumatic or poststernotomy mediastinitis). These conditions are described in more detail later in this chapter.
Clinical Settings and Syndromes
Pneumomediastinum can be categorized as spontaneous or secondary. Spontaneous pneumomediastinum develops without an obvious etiology but may nonetheless have a predisposing condition or precipitating factor. Spontaneous pneumomediastinum is most frequently seen in young adults and is usually a benign, self-limited condition. In contrast, secondary pneumomediastinum arises from a specific pathologic event such as barotrauma from mechanical ventilation, intrathoracic or iatrogenic trauma, infection, or other acute conditions. Compared to spontaneous pneumomediastinum, secondary pneumomediastinum carries higher morbidity and mortality, likely due to the underlying etiology. Thus, in cases without an obvious precipitant, it is important to investigate for possible underlying causes of secondary pneumomediastinum ( Table 84-2 ).
Spontaneous | Secondary |
---|---|
VOLUNTARY ALTERATION IN BREATHING PATTERN | BAROTRAUMA |
Marijuana smoking and cocaine inhalationPulmonary function testing Mountain climbing—“voluntary pressure breathing” Wind instrument playing Yelling, shouting, and singing INVOLUNTARY ALTERATION IN BREATHING PATTERN Childbirth Vomiting Seizures; status epilepticus Violent coughing; sneezing; hiccupping Heavy lifting; athletic competition Straining at stool | Mechanical ventilation Noninvasive mechanical ventilation Manual ventilation during resuscitation, anesthesia, or transport Equipment malfunction or misconnection in anesthesia or oxygen therapy Heimlich maneuver Deceleration injury |
INVASIVE PROCEDURES | |
Esophageal perforation | |
Endobronchial needle aspiration | |
INTRINSIC AIRWAY AND LUNG DISEASE | |
Asthma | |
Atelectasis | |
Bronchiolitis | |
Pneumonia | |
Influenza | |
Measles | |
Tuberculosis | |
Silicosis | |
Foreign body | |
Tumor |
Spontaneous Pneumomediastinum
Predisposing Conditions.
Underlying lung diseases are present in 25% to 44% of patients with spontaneous pneumomediastinum, as shown in two cohorts. Of those with lung disease, more than half had evidence of interstitial lung disease ( eFig. 84-6 ). Other parenchymal diseases associated with pneumomediastinum include atelectasis, bronchiolitis, pneumonia, influenza, measles, and hematogenous tumor metastases.
Obstructive lung diseases, especially acute asthma, are a well described predisposing factor leading to pneumomediastinum. On rare occasions, pneumomediastinum during asthma exacerbations can lead to life-threatening respiratory and hemodynamic compromise. Although chest radiographs are not routinely obtained during attacks of asthma, pneumomediastinum was noted in 5.4% of 479 radiographs of children admitted with asthma attacks.
Cigarette smoking has been implicated as a predisposing factor in spontaneous pneumomediastinum, although the mechanism is unclear.
Precipitating Factors.
Voluntary manipulations of the breathing pattern can sometimes lead to alveolar rupture. “Voluntary pressure breathing,” a practice originally proposed for fliers during World War II, consisted of slow, deep inhalations followed by forced exhalation through tightly pursed lips. This maneuver was also advocated by some mountain climbers as a means of getting more oxygen into the blood. However, this maneuver may lead to alveolar rupture. Patients with pneumomediastinum reported by Vosk and Houston were members of the same climbing party who had both practiced “voluntary pressure breathing” during ascent.
Inhalational drug use is commonly cited as a precipitating factor in spontaneous pneumomediastinum. A typical example is pneumomediastinum after vigorous straining against a closed glottis during marijuana smoking or after inhaling free-base cocaine, during which users sometimes add additional external airway pressure by having an accomplice blow vigorously into their mouths through a cardboard tube. Of note, given the direct toxic effect of cocaine on lung parenchyma, some authors argue that pneumomediastinum following cocaine inhalation should be considered secondary. Recreational inhalation of nitrous oxide from disposable canisters was also reported as a cause of pneumomediastinum in two college fraternity brothers.
Involuntary manipulations of the breathing pattern are more common precipitating factors than voluntary maneuvers. Such causes of pneumomediastinum include straining or exertion such as during childbirth ; vomiting in association with bulimia, hyperemesis gravidarum, and diabetic ketoacidosis ; and prolonged straining during convulsive seizures, athletic competition, and violent coughing, sneezing, hiccupping; and straining at stool. It is reasonable to expect that any activity producing large momentary swings in intrathoracic pressure could result in alveolar rupture leading to pneumomediastinum.
No Predisposing Condition or Precipitating Factor.
Although a history of a potential predisposing event or disease process is elicited in most patients with “spontaneous” pneumomediastinum, a few cases have been described without such associations and are known as Hamman syndrome. Hamman’s original patient was a 51-year-old physician in whom no predisposing factor could be identified. Hamman later reported several additional cases of truly “idiopathic” pneumomediastinum; in modern series, cases have been reported despite normal lung parenchyma as determined by chest computed tomography (CT).
Secondary Pneumomediastinum
Mechanisms for secondary pneumomediastinum include blunt thoracic trauma, perforation of the esophagus or other viscus from invasive procedures, or unusual cases due to pathology in the neck, thorax, or abdomen, as described previously.
Barotrauma.
Barotrauma during mechanical ventilation accounts for up to one third or more of cases of secondary pneumomediastinum in recent studies. Alveolar rupture during positive-pressure ventilation is thought to result from overdistention in an area with abnormal airways or lung parenchyma. Subsequent dissection of air proximally toward the mediastinum results in pneumomediastinum, and rupture through the mediastinal pleura leads to the development of pneumothorax. Before the era of low tidal volume ventilation, barotrauma developed in almost a one fourth of mechanically ventilated patients and was associated with high mortality. Historically, large delivered tidal volumes and high levels of positive end-expiratory pressure were thought to predispose mechanically ventilated patients to barotrauma, but these associations were likely related to the severity of the underlying lung disease. Gammon and others have shown the acute respiratory distress syndrome (ARDS) was the condition most commonly associated with barotrauma and, among ventilated patients, was the only independently associated risk factor for barotrauma. Ventilator settings, including tidal volume, peak and plateau airway pressures, have not been found to be associated with barotrauma in a heterogeneous population of mechanically ventilated patients. Although rates of barotrauma in low tidal volume (6 mL/kg) lung-protective ventilation strategies are reported to be the same as in traditional tidal volumes (12 mL/kg) in large clinical trials, the overall rate of barotrauma appears to be lower when compared with historical references and clinician experiences.
Noninvasive forms of positive-pressure ventilation are less often associated with barotrauma than is conventional mechanical ventilation. This is probably because of the lower airway pressures used and the less severe lung diseases being treated. For instance, continuous positive airway pressure applied by mask was associated with pneumomediastinum in only 1 of 331 applications of this therapy, as cited in one review.
Air or oxygen inadvertently insufflated under high pressure, as for example during manual ventilation during cardiopulmonary resuscitation, is especially likely to cause barotrauma. Improperly connected oxygen tubing preventing exhalation has resulted in life-threatening barotrauma, as have similar errors following anesthesia and surgery.
Deceleration injury, even when there is no open chest wound or displaced rib fracture, can cause barotrauma. Although such deceleration injuries most commonly result from motor vehicle accidents, they can also follow blows to the chest or falls into water with the glottis closed. Pneumomediastinum has also been caused by resuscitation using the Heimlich maneuver.
Infections.
Infectious etiologies of secondary pneumomediastinum include mediastinitis with gas-forming organisms, Pneumocystis jirovecii pneumonia patients infected with human immunodeficiency virus ( eFig. 84-7 ), or pneumonia with cavitary lesions.
Clinical Manifestations and Diagnosis
Symptoms, Physical Signs, and Laboratory Findings
Chest pain is the most common symptom reported by patients with pneumomediastinum. It has been reported in the majority of patients with “spontaneous” pneumomediastinum and may account for some cases of unexplained acute chest pain in otherwise healthy young people. The pain is characteristically substernal in location and aggravated by movement, breathing, and position changes. It often radiates to the back, shoulder, or arm. Discomfort may extend into the neck, and retropharyngeal or perilaryngeal air dissection may give rise to dysphagia or dysphonia, with characteristic muffled voice. Dyspnea and cough are also common symptoms. In half of the cases, physical examination reveals palpable crepitation in the neck and supraclavicular area, and cyanosis and neck vein distention have also been observed. Hamman sign, or Hamman’s crunch, is a crunching or clicking sound synchronous with the heartbeat, heard over the precordium, and increased in intensity during inspiration and in the left lateral decubitus position. It has been described as similar to the noise produced by rubbing two rubber balloons together. Although considered by Hamman to be pathognomonic for the presence of mediastinal air, it can occasionally be heard in other circumstances such as with pneumothorax without radiographic evidence for pneumomediastinum.
Low-grade fever, along with mild to moderate leukocytosis, likely results from reactive inflammation associated with air dissecting in the mediastinum. In one series, leukocyte counts were reported to be greater than 10,000/mm 3 in 16 of 23 patients and greater than 20,000/mm 3 in 5 of 23, returning to normal without treatment within 1 to 2 days.
Electrocardiographic changes are seen in some cases of pneumomediastinum without evidence of other cardiac abnormalities. The electrocardiogram may show diffusely low voltage, nonspecific axis shifts, ST-T wave changes, and ST segment elevation in the lateral precordial leads. These changes are also observed in association with pneumothorax and may be in part related to physical displacement of mediastinal structures.
Radiographic Features
Pneumomediastinum is most often detected by chest radiography and the finding of pneumomediastinum may be the first manifestation of extra-alveolar air. The diagnosis is usually made by demonstrating a thin line of radiolucency, best seen along the left heart border ( Fig. 84-4 and eFig. 84-8A ; see eFig. 84-5A ). Other common signs are the highlighting of the aortic arch, which is surrounded by increased radiolucency, and the “continuous diaphragm” sign, an unbroken radiolucent line extending from one hemidiaphragm to the other beneath the heart ( Fig. 84-5 and see eFig. 84-8B ). Mediastinal air may be easier to see on films obtained with the patient in lateral projection, which may demonstrate retrosternal air or vertical lucent streaks outlining the aorta, pulmonary artery (the so-called ring-around-the-artery sign; see eFig. 84-8C and D ), or other mediastinal structures. Less commonly encountered chest radiographic findings of pneumomediastinum include the “extrapleural air” sign (see eFig. 84-8E and F ), Naclerio’s “V” sign (see eFig. 84-8G ), elevation of the residual thymus (typically in younger patients, referred to as the thymic spinnaker-sail sign ; see eFig. 84-8H-J ), and air or gas trapped within the pulmonary ligament (see eFig. 84-8K ). Distinguishing between mediastinal emphysema and a small pneumothorax may be difficult. Film obtained with the patient in the lateral decubitus position may show air rising to the most elevated portion of the thorax if it is free to move in the pleural space, whereas mediastinal air moves little with changes in position. Other chest radiographic findings that may mimic the appearance of pneumomediastinum include an unusual appearance of the major (oblique) fissure ( eFig. 84-9 ), medial bullae, and Mach bands ( eFig. 84-10 ).


Subcutaneous emphysema is readily detected as streaks or pockets of air outlining the tissue planes in the neck or chest. It often outlines the tissue compartments of the chest wall, clearly identifying the pectoral muscles. Both subcutaneous emphysema and pneumomediastinum are common findings in patients on mechanical ventilators, being present in 7% of cases in one early series ( Fig. 84-6 ). These signs of alveolar rupture during positive-pressure ventilation are important clinical danger signals, because pneumothorax may develop in at least half of these patients.

Chest CT is more sensitive than chest radiographs for detecting aberrant air in all its forms and should be considered when important management decisions rest on a given distinction or when the underlying cause is not evident. This is especially true in patients with pneumomediastinum due to blunt trauma, in which only 15% could be detected by chest radiographs.
Approach to Management
Natural History
In most cases of pneumomediastinum, air spreads throughout the mediastinum and vents out of the thorax into the subcutaneous tissue planes. Subcutaneous emphysema can become massive and prove distressing to both patient and physician, but it is seldom dangerous. Subcutaneous air collections will resolve spontaneously without surgical decompression once the primary air leak has sealed. Complete resolution of subcutaneous emphysema usually requires a couple of weeks, and hence counseling patients who are clinically stable is an important component of its management.
Management of Spontaneous Pneumomediastinum
Treatment of predisposing or precipitating factors of spontaneous pneumomediastinum is usually followed by gradual resolution of the aberrant air. Supplemental oxygen may hasten reabsorption, but specific therapy for pneumomediastinum is rarely needed. Most cases resolve with conservative management alone.
In rare instances pneumomediastinum can produce life-threatening cardiovascular collapse. An early, well-documented incident was described by Laënnec more than 150 years ago, as related by Munsell. The great French clinician was called to see a small boy who had been run over by a dung cart: Laënnec describes how his 4-year-old patient was placed in a tent with a candle for light. With the boy in extremis, Laënnec inserted sharp sticks into the boy’s neck, supraclavicular areas, and anterior portion of the chest; a rapid, large outpouring of air extinguished the candle and the boy recovered. Modern medicine can add little more to this emergency decompression regimen except for the substitution of needles for sticks.
Most experienced clinicians reserve invasive therapy for cases of increasing airway impingement or cardiovascular compromise ( eFig. 84-11 ). In these instances, small infraclavicular venting incisions seem to be the most prudent initial approach.
Management of Pneumomediastinum During Positive-Pressure Ventilation
The appearance of mediastinal or subcutaneous air in a patient receiving positive-pressure ventilation should prompt an assessment of the patient’s physiologic response as well as the ventilator status. Immediate discontinuation of positive-pressure ventilation is usually not feasible, but adjustments to the ventilator can diminish the tendency of air to enter the mediastinum and reduce the risk of developing pneumothorax. Delivered tidal volumes should be reduced if possible. Positive end-expiratory pressure should be reduced or discontinued. Occult positive end-expiratory pressure, or “auto-PEEP,” can augment air leakage and, if present, should be reduced. Finally, bronchospasm and other potentially reversible contributors to air trapping during mechanical ventilation should be treated. Cough suppression should be considered.
Tracheobronchial disruption causing extra-alveolar air requires prompt diagnosis and surgical repair. This condition should be considered in a patient with blunt thoracic trauma in the presence of extensive soft tissue emphysema, airway bleeding, or nonresolving pneumothorax (see eFig. 84-2 ). Tracheobronchial disruption is certain if, on chest radiography, the collapsed lung tissue appears to have fallen away from the hilum (see Fig. 76-10 ). When these findings are present, the patient should be taken to the operating room, where the first step is emergent bronchoscopy to confirm the diagnosis. Performing the bronchoscopy in the operating room allows for immediate surgical intervention once confirmed. An exception can be made in the scenario of a trauma patient who is intubated and clinically stable with a pneumomediastinum found on CT imaging that could represent either an artifact or a small tear in the tracheobronchial tree. In these stable patients, one can do a quick bedside bronchoscopy to confirm the diagnosis which, if confirmed, would necessitate immediate operating room availability. However, these are situations which must be closely coordinated with thoracic surgery to ensure there is no delay in intervention.
Mediastinitis
The term mediastinitis encompasses a number of loosely related processes that induce inflammation of mediastinal structures. Most forms of mediastinitis are infectious, and variations in their clinical presentation depend largely on the chronicity of the underlying process rather than on the specific microbial cause. Acute mediastinitis is usually dramatic in presentation and requires prompt recognition and treatment. Chronic mediastinitis encompasses a spectrum that ranges from active granulomatous infection to end-stage fibrosis. The paucity of recent literature on these subjects reflects the relative rarity of mediastinitis. Nonetheless, recognition of mediastinitis and its forms is important because of its devastating morbidity and mortality.
Acute Mediastinitis
Acute mediastinitis was once a rare and usually fatal condition seen after rupture of the esophagus from forceful vomiting (see eFig. 84-11 ) or in conjunction with penetrating trauma. With the advent of endoscopic procedures and cardiac surgery via median sternotomy, acute mediastinitis is now most commonly encountered as an iatrogenic complication and may vary in clinical presentation. Relatively indolent infections may be termed “suppurative” rather than “acute.” In either case, a distinction is drawn between these disorders and chronic “granulomatous” and “fibrosing” mediastinitis.
The following discussion characterizes the clinical picture and management of acute mediastinitis according to the mechanism of access to the mediastinum ( Table 84-3 ).
PERFORATION OF A THORACIC VISCUS |
Esophagus |
Forceful vomiting (Boerhaave syndrome) |
Direct penetrating trauma |
Impacted foreign body |
Instrumentation: esophagoscopy; sclerotherapy; esophageal obturator airway |
Erosion: carcinoma; necrotizing infection |
Trachea or Main Bronchi |
Direct penetrating trauma |
Instrumentation: diagnostic bronchoscopy; therapeutic bronchoscopy; intubation |
Foreign body |
Erosion of carcinoma |
DIRECT EXTENSION OF INFECTION FROM OUTSIDE MEDIASTINUM |
Retropharyngeal space; odontogenic |
Pancreatitis |
Lung; pleura; pericardium |
Lymph node |
Paraspinous abscess |
MEDIASTINITIS AFTER CARDIOTHORACIC SURGERY |
INHALATIONAL ANTHRAX |
Mediastinitis Resulting from Visceral Perforation
Boerhaave syndrome refers to esophageal rupture associated with forceful vomiting (see eFigs. 84-5 and 84-11 ), classically after overeating or excessive drinking. It is the most familiar example of acute mediastinitis, although no longer the most common. Unilateral or bilateral hydropneumothorax is common and quickly progresses to empyema ( Fig. 84-7 ). Spontaneous esophageal rupture can be difficult to diagnose and may be mistaken for an abdominal catastrophe especially in the patient who may not be able to relate a clear history. During a violent vomiting episode, the pressure in the esophagus can increase to the point at which it overcomes the tensile strength of the normal esophageal wall. The most common location of perforation is the lower left posterolateral wall, where the muscle bundles in the longitudinal layer of the esophagus can separate, allowing a bubble of mucosa to protrude into the mediastinum and burst.

In the classic description of acute mediastinitis related to esophageal rupture, the onset is sudden and dramatic. Patients complain of severe, unrelenting substernal chest pain which is worsened by breathing or coughing, and may report a sense of impending doom. The pain may be referred into the neck and ear if there is involvement of the superior mediastinum. Radicular pain that radiates around the chest and between the scapulae suggests involvement of the posterior or inferior mediastinum. Symptoms and signs of systemic toxicity—high fevers, chills, tachycardia, and tachypnea—are prominent. Examination may reveal supraclavicular fullness, tenderness over the sternum or sternoclavicular joints, crepitus, and other signs of mediastinal and subcutaneous emphysema. Hamman sign is characteristic but not always present. Tracheal deviation, jugular venous distention, and other signs of compression of mediastinal structures may appear later in the clinical course. These features are typical after spontaneous esophageal rupture as seen in Boerhaave syndrome, but may not be present in less acute settings.
While still rare, esophageal perforation during diagnostic or therapeutic endoscopic procedures is an important cause of acute mediastinitis in the contemporary era. Diagnostic upper endoscopy causes a perforation at a rate of less than 0.03%. Therapeutic maneuvers, such as dilation of strictures ( eFig. 84-12 ) and endoscopic mucosal resection, have a much higher risk of perforation, from 2% to 6%. Additionally, therapy of malignant lesions is more likely to be complicated by perforation than that of benign lesions.
The esophageal obturator airway and swallowed foreign bodies can also perforate the esophagus, particularly if their use is intended to induce injury. One series describes six prison inmates who swallowed hypodermic needles fashioned in the shape of “stars” to win temporary reprieves from incarceration. All required surgical management. Accidental or intentional ingestion of caustic solutions such as paraquat or lye also can lead to esophageal perforation.
The diagnosis of esophageal perforation depends on an appropriate degree of clinical suspicion. On chest radiograph, the hallmarks are diffuse mediastinal widening and the presence of air in the mediastinum and adjacent soft tissues. Mediastinal air-fluid levels may be seen, and pneumothorax or hydropneumothorax may be present. CT can delineate these abnormalities more clearly. Passage of ingested contrast material into the periesophageal or pleural space may be observed.
Frank uncontained esophageal perforation requires prompt surgical repair, drainage of the mediastinum and often the pleural spaces, and administration of appropriate antibiotics. Percutaneous catheter drainage of mediastinal abscesses, under CT guidance, has been used when the infection is localized and the clinical setting is less urgent (see eFig. 84-12E-H ). In a series describing 51 patients 3 died before intervention could be accomplished, 31 underwent standard thoracotomy and repair leading to 11 deaths, and 17 highly selected patients with minimal contamination were managed successfully with targeted drainage and antibiotic therapy.
If esophageal perforation due to endoscopy is detected early, morbidity and mortality can be avoided with prompt treatment. Clips may be used to repair small perforations endoscopically if detected immediately. In selected cases, esophageal perforation can be contained using endoscopically placed stents. In addition, a surgeon should be involved as soon as a perforation is detected and broad-spectrum antibiotics should be administered. The rate of recovery is high when perforations are repaired within 24 hours.
Complications of acute mediastinitis after esophageal rupture may include localized abscess formation, extensive pleural empyema, and persistent esophagocutaneous fistulas. A prolonged course is usual, and reexploration may be necessary to ensure adequate drainage. Reported mortality has varied due to differences in patient selection and management approaches. Timing of surgical drainage has been of prime importance in determining the clinical outcome. Modern series reporting the use of consistently aggressive surgical management cite up to 90% survival after spontaneous esophageal rupture.
Bronchoscopic airway perforation and migration of indwelling central venous catheters are other iatrogenic causes of mediastinitis. Bronchoscopy is a much less frequently reported cause of mediastinitis than esophagoscopy. However, increasing use of laser and mechanical endobronchial procedures, often performed in the setting of malignancy, with chronic airway colonization or postobstructive pneumonia, add to the likelihood of potential mediastinal complications. Mediastinitis has been reported after endobronchial ultrasound-guided transbronchial needle aspiration, but is overall a rare event. Intravascular catheters may be another source of acute mediastinitis when the catheter tip erodes through the vessel wall into the mediastinum ( Fig. 84-8 and ). Instillation of hyperosmotic, vesicant, or vasoactive substances via these catheters may induce a chemical, rather than an infectious, inflammation.








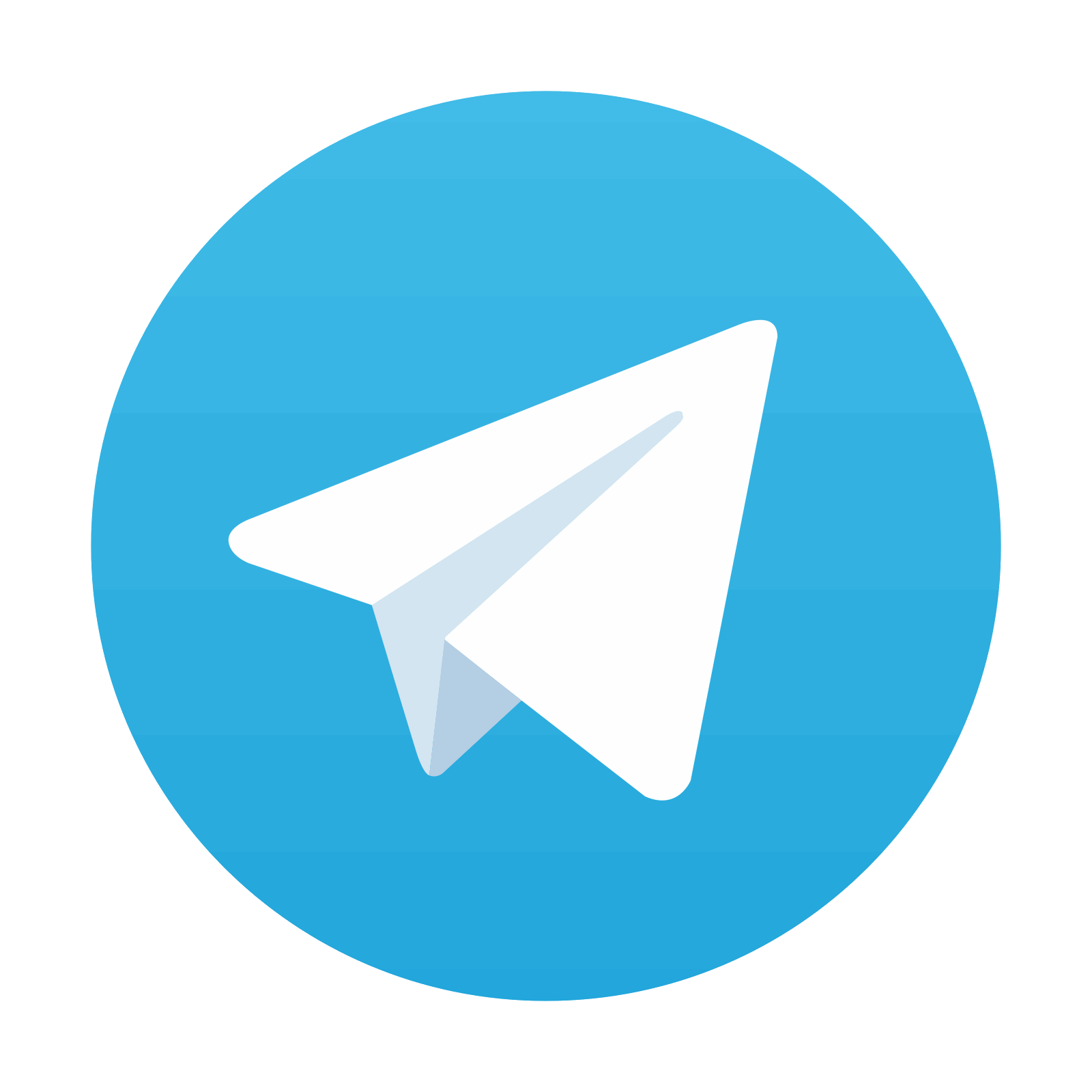
Stay updated, free articles. Join our Telegram channel

Full access? Get Clinical Tree
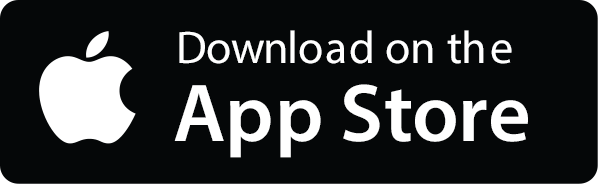
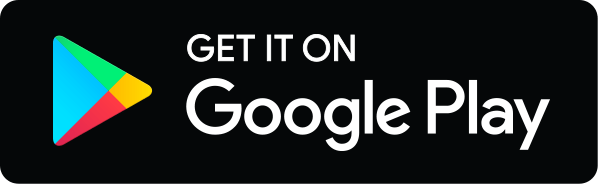
