CHAPTER 31 Pleural Tumors
PLEURAL EMBRYOLOGY AND ANATOMY
The pleural membrane is thin and translucent. It is composed of five separate layers: (1) the mesothelial layer (flattened mesothelial cells joined by tight junctions), (2) a thin submesothelial connective tissue layer, (3) a superficial elastic tissue layer, (4) a loose subpleural connective tissue layer in which run lymphatics, nerves, arteries, and veins, and (5) a fibroelastic layer that is adherent to the underlying structures (lung, mediastinum, diaphragm, chest wall). The mesothelial cell features long slender microvilli that extend into the pleural space and are believed to release hyaluronic acid into the pleural fluid.1
ASBESTOS-ASSOCIATED PLEURAL PATHOLOGY
Biologic Capabilities of Asbestos Fibers
Asbestos is divided into two main types based on fiber length and other physical properties that may account for its biopersistence in humans. The fibers of the first type are short and serpentine. Chrysotile (or white asbestos) is the predominant member. The second type, known as amphibole asbestos, has long, thin, straight fibers. It has several subtypes, including crocidolite, actinolyte, tremolite, anthophyllite, and amosite.2 Approximately 95% of the asbestos currently used worldwide is chrysotile. The remaining 5% is amosite and crocidolite, although these groups of fibers are commonly mixed together.3
Two conflicting theories have been advanced to explain the oncogenic potential of asbestos fibers based on their physical properties of dimension, shape, and length. The amphibole hypothesis proposes that amphibole fibers owe their durability and their biopersistence in the lung to their long length. Proponents of this hypothesis believe that the short serpentine chrysotile fibers are broken down and cleared too quickly to provoke cancer.4 The Stanton theory postulates that amphibole asbestos is carcinogenic because the long fibers are able to penetrate deeper into the pleura, where they come in contact with and incite mesothelial cell proliferation.5 Whatever the cause, it appears that chrysotile fibers are cleared from the body by macrophages much more quickly than amphibole fibers, which can remain in the lung for many years after exposure, accounting for their high degree of biopersistence.
There is evidence that the biopersistence of asbestos fibers is associated with malignancy in animals6,7 and in humans.8 Although consensus has not been reached on the precise carcinogenic potential of the different fiber types, or the level or length of exposure needed for the malignant transformation to mesothelioma, an expert panel (level 4 evidence) has recently published an opinion on this matter. The consensus decision of this panel suggests that the longer, biopersistent fibers associated with amphibole asbestos (particularly, crocidolite) have greater carcinogenic potential than the short fibers of the serpentine asbestos.9
Epidemiology of Mesothelioma
Malignant mesothelioma was first identified as a discrete diagnosis in 1870.10 The name was coined in the 1930s.11 Suspicion of an association between asbestos exposure and mesothelioma development was initially documented in the medical literature between 1920 and 1950.12–14 Despite the numerous case reports, it was not until 1960 that Wagner and colleagues reported a series of cases that demonstrated a strong association between mesothelioma and occupational exposure to asbestos in asbestos miners in South Africa.10 A clear link between asbestos exposure and pleural tumor development was documented in the United States by Selikoff and colleagues in the 1960s and 1970s.15,16 Further evidence of this association followed, with multiple case-control series from 1965 to 1975. These reports were summarized in an epidemiologic review of mesothelioma by Britton.17 In this review, the most notable risk of mesothelioma was associated with occupational exposure to asbestos, primarily in shipyards where crocidolite asbestos was used in naval insulation materials. Other defined exposure groups with varied relative risks of mesothelioma development included workers who installed insulation and workers in the asbestos product manufacturing industry or in heating and construction industries.17 After Wagner’s publication, further worldwide case series comparing amphibole and chrysotile fiber exposures from 1980 to 1999 confirmed the association of asbestos exposure (particularly amphibole fibers) and mesothelioma. Even chrysotile miners were identified to be at high risk for mesothelioma, although contamination of the chrysotile asbestos by amphibole fibers (particularly tremolite) has been questioned in these miners as the true cause.17,18
The latency period (20 to 50 years) between exposure to asbestos and mesothelioma development is substantial.19 Although the epidemiologic data linking asbestos exposure to mesothelioma are clear, the lifetime risk of mesothelioma development in workers exposed to asbestos ranges from 4.5% to 10.0%.20–23 Thus, the lack of a strong, direct, quantifiable association between asbestos exposure and pleural tumor development impedes the prediction of individual disease development, and future predictions are relevant only in broad demographic terms.
Western populations were exposed to asbestos as an environmental hazard when it was used in an uncontrolled fashion in the shipbuilding and construction industries between 1940 and 1970. Despite worldwide attention by environmental regulatory bodies, the latency of the disease suggests that the peak incidence of the disease in Europe, North America, and Australia may be in the next 10 to 15 years.24,25 Prediction risks for mesothelioma development suggest that the disease will continue to increase in Europe, to peak in 2020, and thereafter to rapidly decline, as the workforce exposure should affect only the population born before 1950 (assuming the commencement of work at age 20, when asbestos regulations were initiated).17,24 The prediction for a mesothelioma peak in the United States is 2004; Australia, 2015; and Japan, 2025. However, these assumptions do not include many unanticipated factors such as the attack on the World Trade Center in 2001, where an estimated 10 million New Yorkers were exposed to asbestos dust.26,27
Population Exposure
The first group comprises individuals with direct exposure either through work or by direct inadvertent exposure to asbestos fibers. An example of this exposure group is the miners of asbestos in Wittenoom, Australia. These miners were exposed at work, but because asbestos was used in lieu of grass to cover the schoolyards and playgrounds, their children also received direct exposure.25
The third group represents those who are passively exposed through other family members who work directly with asbestos and carry the fibers home on their clothing. This group includes persons in close contact with the first two groups (e.g., wives of asbestos workers).28
A small number of individuals have mesothelioma but no history of occupational exposure to asbestos. Some may have contacted asbestos fibers in their natural habitat,29 or they may have a genetic predisposition.30,31 It has been postulated that simian virus 40 (SV40) plays a synergistic role with asbestos in the development of mesothelioma, either as a clastogenic transformer (an inducer of chromosomal damage) or as a cytologic protector, which permits chromosomally damaged cells to survive and propagate rather than die (apoptosis).32,33 SV40 was identified as a contaminant in polio vaccines from 1950 to 1963, and the extent of its role in mesothelioma development is still both very controversial and undetermined.
Finally, 2% to 5% of mesothelioma cases arise in a pediatric population, which suggests a genetic rather than an environmental cause of mesothelioma in these individuals.34
Pathogenesis of Asbestos Fibers
Benign asbestos-related pleural disease is thought to manifest as either discrete parietal pleural plaques or as diffuse disease involving visceral pleural hyperplasia and fibrosis.35 The mechanism whereby asbestos fibers reach the parietal pleura to evoke pleural plaque formation is unknown. However, as described earlier, it is suspected that the inability of pulmonary macrophages to phagocytose these particles is a result of their length, and that the fibers burrow through the lung and eventually come into contact with the mesothelial cells in the pleural membranes. Several theories have been proposed to explain how the fibers pass from the visceral to the parietal mesothelial cells. There are several putative causes. The fibers may protrude through the visceral pleura, abrading and irritating the parietal pleural surface. The fibers may migrate across the pleura from the visceral to the parietal surface, or they may disseminate via lymphatic drainage to the parietal mesothelial cells.27,35
The effect of asbestos on mesothelial cells is complex, multifactorial, and difficult to explain. It has been shown, for example, that human mesothelial cells often die in vitro after contact with, or phagocytosis of, asbestos fibers.36,37 A possible explanation is that asbestos fibers may induce the proliferation of cellular cytokines that have cytoprotective effects and that allow asbestos-transformed mesothelial cells to survive and proliferate. TNFα expression has been shown to increase after mesothelial cells and macrophages are been exposed to asbestos, and this factor may enable the asbestos-damaged cells to survive.38 Fiber persistence may lead to chronic inflammation with increased cycles of cell growth that might induce cellular mitotic changes. These changes may gradually cause the mesothelial cells to acquire genetic and epigenetic changes that lead to neoplasia. Even without directly interfering with the DNA machinery of the cell, these fibers may secondarily select for cells with oncologic potential.
In addition to inducing cellular proliferation, asbestos fibers have other cytologic and clastogenic properties that could lead to neoplasia. Incorporation of asbestos fibers into the mesothelial cell, for example, produces oxygen free radicals,39,40 cytokines, chemokines, and growth factors such as epidermal growth factor (EGF) and TGFα.41–43 These elements can promote the proliferation of mesothelial cells, rendering them more susceptible to DNA and genetic damage.44 Asbestos induces phosphorylation of mitogen-activated protein (MAP) kinases and of the extracellular signal–regulated kinases (ERKs) 1 and 2 with increased downstream expression of tumor proto-oncogenes.43
Asbestos fibers also have clastogenic properties—that is, they may act directly on the chromosomes.45 They may sever or disrupt the mitotic spindle of cells, causing chromosomal abnormalities such as aneuploidy to occur.46 Finally, they may induce epigenetic tumor suppressor silencing via promoter DNA CpG methylation, which permits tumorigenic cells to enter the cell cycle instead of halting them at the G1/S interphase of mitosis.47
Mesothelial Cell Neoplastic Changes Attributed to Asbestos Exposure
Chromosomal Abnormalities in Mesothelioma
Karyotypic alterations result from somatic mutations; however, gene deletions, gene silencing, and RNA editing are also common chromosomal aberrations in malignant mesothelioma. DNA sequencing techniques and comparative genomic hybridization permit discrimination between clonal proliferations of tumor cells.48,49 These techniques may also help in the histologic identification and sorting of mesothelioma subtypes. They may aid by distinguishing mesothelioma from similar-appearing tumors such as adenocarcinoma.50 Whole-transcriptome, deep sequencing of single mesothelioma tumors has revealed unique individual tumor profiles of chromosomal and mRNA expression.51
These chromosomal changes in malignant mesothelioma often are multiple, leading to clonal proliferations that show individual variation (losses are more common than gains).50 In mesothelioma, a common deletion occurs at the 9p21 locus. This occurs at a frequency of 70% in mesothelioma cell lines and in up to 22% of primary tumor specimens.52,53 This locus encodes two inhibitors of the cyclin-dependent kinases (CDKs)—namely, p16INK4a (CDKN2A) and P15INK4b (CDKN2B), which prevent the phosphorylation of retinoblastoma (Rb) protein. The Rb protein modulates cell entrance into the S phase from G1 of the cell cycle. Phosphorylation of this protein, which is prevented by CDK inhibitors, allows uncontrolled cellular entry into S phase. The 9p21 gene also codes for p14ARF, which acts as a regulator for an important tumor suppressor oncogene, p53. This gene encodes tumor protein p53, which responds to diverse cellular stresses such as hypoxia, DNA damage, and oncogene activation, to regulate target genes that induce cell cycle arrest, apoptosis, senescence, DNA repair, or changes in metabolism. P53 protein is expressed at a low level in normal cells and at a high level in a variety of transformed cell lines, where it is believed to contribute to transformation and malignancy. P53 is a DNA-binding protein containing transcription activation, DNA-binding, and oligomerization domains. It is postulated to bind to a p53-binding site and activate expression of downstream genes that inhibit growth and invasion, and thus function as a tumor suppressor by initiating the transcription of genes leading to downstream effects on cell cycle arrest in G0 and apoptosis. A downstream target of p53 is p21, a multifunctional CDK inhibitor. Loss of p21 expression is associated with decreased patient survival.54 Also, there is a frequent loss of chromosome 22 in malignant mesothelioma.55 The tumor suppressor gene NF2 is located on this chromosome.
Tumor Suppressor Genes in Mesothelioma
Tumor Suppressor Genes and CDK Inhibitor Loss
Ironically, malignant mesothelial cells lack mutation of the two most common genetic mutations found in most cancers—namely, tumor suppressor genes p53 and pRb.56 The tumor suppressor gene p53 is important for cell cycle arrest, as it prevents propagation of DNA-damaged cells or genetically unstable cells. It acts by stimulating the expression of p21 which, in turn, acts as a CDK inhibitor. In an equivalent manner, the retinoblastoma gene (Rb), as noted earlier, also halts chromosomal synthesis and therefore tumor proliferation.
However, the chromosomal aberrations of malignant mesothelioma cells ultimately adversely affect these two tumor suppressor genes by upstream regulation of their gene products. Mutational deletion of the 9p21 locus in mesothelioma cells affects p14ARF, an upstream regulator of p53. Deletional interruptions of this gene inactivate TP53-MDM2, which is extremely important for intrinsic cellular control of chromosomally damaged cells. Inactivation of this pathway by decreasing p21 gene products allows CDK to phosphorylate Rb protein, which then allows damaged cells to enter the S phase of the cell cycle.57 Viral transduction of p14ARF into mesothelioma cells in culture leads to increased production of p53 and p21, resulting in an increase in dephosphorylated Rb and G1 arrest and an inhibition of mesothelioma cell growth.58
In an equivalent manner, the loss of p16INK4a leads to loss of CDK 4 and 6, leading to phosphorylation of Rb gene by the lack of CDK inhibition.57 Deletion of p16/CDKN2A has been reported in greater than 70% of mesothelioma cells.50,59 As seen with p53, mesothelioma cells transfected with adenovirus expressing p16INK4a demonstrate decreased phosphorylation of Rb,60 leading to cell cycle arrest, cell death, and tumor regression.
Merlin and RAS-ERK Pathway
Changes in chromosome 22 may lead to altered expression of NF2, or somatic mutational changes may lead to NF2 alterations, which occur in approximately 50% of patients with mesothelioma.61 NF2 encodes for a protein named merlin (alternatively called schwannomin). This protein is important in the connection between the plasma membrane and the cytoskeleton of the cell and may act as a tumor suppressor gene by inhibiting the RAS-ERK pathway and inducing cyclin D1 expression. Inhibition or abrogation of NF2 function and its downstream product merlin may lead to a lack of contact-dependent growth arrest,62–64 leading to tumor proliferation at the expense of neighboring normal cells.
Oncogene Activation in Mesothelioma
The transcription factors AP-1 and β-catenin are often upregulated in malignant mesothelioma; AP-1 is upregulated by Fra-1 which is a member of the Fos family of transcription factors. Fra-1 expression is increased in malignant mesothelioma cells, in response to asbestos or EGF and reduced with inhibitors of ERK. Mesothelioma cells transfected with either an ERK inhibitor or a dominant negative fra-1 reverse their transformed phenotype.65
The transcription factor β-catenin is controlled by Wnt signaling, so that the presence of Wnt inhibits phosphorylation of adenomatous polyposis coli (APC) and axin, allowing persistence of β-catenin. Increased presence of β-catenin leads to nuclear binding of T-cell factor/lymphoid-enhancer factor (Tcf/Lef) proteins with downstream activation of transcription factors. Mesothelioma cells have been shown to have altered APC expression,66 and they may express upstream negative inhibitors of the Wnt pathway (Wnt inhibitory factor [WIF-1] and secreted frizzled-related protein [sFRP]).67,68 Extracellular signaling, through the overexpression of membrane disheveled proteins with downstream activation of β-catenin, may play an important role in malignant mesothelioma.57
Epigenetic Maneuvers
Promoter methylation has been shown to be effective in inactivating WIF-1 and sFRP.69,70 Hypermethylation of DNA is a common epigenetic method of inhibition of tumor suppressor gene function.71,72 In the context of asbestos exposure, however, there may be a dose-dependent increased induction of methylation as a result of asbestos fibers.47 The precise mechanism for hypermethylation of this phenotypically important pathway is still not established. It may also be directly attributable to the clastogenic properties of asbestos fibers, or alternatively to repeated inflammatory insults of asbestos-generating reactive oxygen species, which may lead to increased mitotic stimulation and promoter methylation. Acquired generations of accrued genetic and epigenetic chromosomal changes leading to malignancy may thus be induced.47
Growth Factors in Mesothelioma
Platelet-derived growth factor (PDGF) chains A and B increase in response to asbestos exposure; they have been implicated in the development and progression of malignant mesothelioma.50,73 Asbestos also induces autophosphorylation of EGF receptor (EGFR). This may result in the activation of the MAP kinase cascade and ERK1 and ERK2 kinases, leading to expression of proto-oncogenes that encode members of the fos-jun and activator protein 1 families.43 Hepatocyte growth factor (HGF) can promote cell growth and migration mediated via downstream AKT and ERK pathways by binding to its receptor c-met. Circulating levels of this growth factor are elevated in mesothelioma patients, so inhibition of HGF receptor c-met may be a therapeutic option.74 EGFR (member of the erbB family of tyrosine kinase receptors) is over-expressed in malignant mesothelioma in association with angiogenesis and proliferation.75 There are multiple ligands which can bind to and activate this receptor, leading to intracellular tyrosine kinase autophosphorylation and signaling pathways of cell proliferation, differentiation, and immortality. The two most important ligands are EGFR and TGFα, both of which are increased by mesothelial asbestos exposure. Inhibition of EGFR and VEGF pathways by tyrosine kinase inhibitors76 and inhibitors to VEGF production or VEGF receptors77 will decrease or inhibit mesothelioma cell growth. VEGF production is increased by SV40 infection of mesothelioma cells.78 Inhibitors of VEGF79,80 and EGFR pathways76 in mesothelioma patients are currently used in clinical studies.
Viral Activation of Mesothelioma Cells
SV40 is a double-stranded circular polyomavirus that contaminated polio vaccines in the United States, Canada, Europe, Asia, and Africa between 1955 and 1963. This virus has the potential, based on molecular, pathologic, and clinical evidence, to cause cancer.81 It has two major chromosomal regions, one of which harbors latent oncogenic potential. The early region encodes for SV40Tag (large tumor antigen), SV40tag (small tumor antigen), and 17 KT; the late region encodes for structural proteins of the virus.
It has been postulated that SV40 infection may increase the cellular production of growth factors, such as VEGF82 and HGF, through autocrine and paracrine mechanisms.78 It may also protect cells from apoptosis by the ectopic production of telomerase, which prevents chromosomal shortening with successive cell divison.83
SV40Tag can bind to and inactivate the tumor suppressor genes p53 and Rb, unleashing uncontrolled tumor growth. Small tumor antigen (SV40tag) may inhibit PP2A, leading to dephosphorylation of members of the MAPK family and deactivation of Wnt and ERK, which may lead to increased cellular transcription.84
Despite the powerful putative oncologic attributes of SV40 virus, however, there is much debate in the literature as to the role of SV40 in cellular transformation; the oncologic potential of SV40 is very controversial and current opinion discounts its oncologic potential.85,86 The counter-argument to the role of SV40 in inducing neoplasia in mesothelial cells is supported by the lack of viral particles identified within mesothelioma cells.87 Further support for this counter-argument is empirically derived from the knowledge that p14ARF and p16INK4a cause mesothelioma mutational changes, with upstream deactivation of p53 and Rb, thus rendering SV40 inactivation of these tumor suppressor genes redundant and therefore obsolete.
Apoptotic Processes
Extracellular ligands of the caspase pathway, such as TNF, TNF-related apoptosis-inducing ligand (TRAIL), and Fas ligand, induce programmed cell death in normal cells. Intracellular mediators of apoptosis, including telomerase (see Viral Activation of Mesothelioma Cells, earlier) and proteins from the bcl-2 family, which may have proapoptotic and antiapoptotic properties, are increased in mesothelioma patients. Protein expression from this family may have a diagnostic and prognostic role in clinical medicine.75,88 Proapoptotic Bcl-2 proteins induce apoptosis by increasing mitochondrial membrane permeability, in turn leading to caspase pathway activation. Antiapoptotic Bcl-xl expression is increased in mesothelioma. This can also be targeted by inhibitors of antisense oligonucleotides89 or viral vector transfection of mesothelioma cells.90
CLINICAL ASPECTS OF PLEURAL TUMORS
It is often difficult to distinguish benign from malignant pleural reactions (Box 31-1). As with other benign pleural conditions, the mesothelial cell also may release mediators such as cytokines, chemokines, oxidants, and proteases. These mediators may induce an exudative pleural reaction that gives rise to a proliferative hyperplasia of the mesothelial and submesothelial layers.1,35 This reaction can resemble a malignant process. Thus, pleural thickening can be attributable to multiple causes, ranging from benign inflammation to malignancy, as summarized in Box 31-2.
Box 31–1 Benign Causes of Pleural Hyperplasia
Modified from Cagle PT, Churg A. Arch Pathol Lab Med 2005;129:1421-7 95; and King J, Thatcher N, Pickering C, Hasleton P. Histopathology 2006;49:561-8.96
Box 31–2 Causes of Pleural Masses
Inflammatory Pleural Reactions
The pleura reacts to pleural injury either by development of a reactive mesothelial hyperplasia or organizing pleuritis (as opposed to atypical mesothelial hyperplasia, described later) or by the formation of nodular pleural plaques. Plaques are acellular deposits on the parietal pleura that often calcify. They are usually associated with asbestos exposure.91,92 These benign lesions are often multiple and occur bilaterally. Their presence raises the possibility of mesothelioma or lung cancer, either on presentation or in the future.
Pulmonary Tumors That May Resemble Pleural Tumors
Inflammatory pseudotumor of the lung is a rare pulmonary tumor in adults, but it is the most common primary pulmonary tumor in children.93 These tumors may be associated with a pleural thickening that responds to steroid therapy.94
The specific histologic features of a benign reactive mesothelial hyperplasia that mimics malignancy include high cellularity, cytologic mitoses, necrosis, papillary excrescences, and entrapment of mesothelial cells in organizing pleuritis. Immunohistochemistry may be of limited help in differentiating benign from malignant pleural pathology. Its primary value may be in the demonstration of invasion by immunostaining of invasive cells. Invasion is a key marker for malignancy and must be differentiated pathologically from entrapment, pseudo-invasion, or sequestration.95,96
Indeterminate lesions may occur on pleural biopsy. These are revealed by a proliferation of atypical mesothelial cells, either in a monolayer or piled up with cellular accumulations. These lesions may demonstrate increased cytologic atypia without evidence of true invasion; they may remain benign or degenerate into malignancy.95,97
Benign Pleural Tumors
Benign pleural tumors comprise less than 5% of all pleural tumors. Far more commonly, they represent either metastatic cancer or a diffuse malignant pleural mesothelioma. These malignant tumors share common properties with benign pleural tumors, which can make it difficult to establish the diagnosis, particularly with small biopsies of minimal amounts of tissue. The common properties of benign and malignant pleural tumors are that both originate from or metastasize to mesothelial or submesothelial surfaces, both may recur after surgical removal, and both are difficult to identify with limited tissue biopsy material because of patterns of heterogeneous cellularity.98
A radiograph of a patient with benign tumors of the pleura reveals a thickened parietal layer with underlying lung compression. Occasionally, these tumors have the appearance of lung invasion (particularly if they are visceral pleura based), as in the inverted fibroma form of solitary fibrous tumors. These pleural abnormalities are identified by a variable thickening of either the visceral or parietal pleura. This imposes a diagnostic dilemma because of the many possible etiologies for pleural masses in the differential diagnosis. Furthermore, fine-needle aspirates of these abnormalities are often unreliable for confirming a diagnosis as a benign pleural tumor.96,99–101
From a clinical perspective, primary pleural tumors span a pathologic spectrum ranging from benign tumors, to benign tumors with some malignant features, to frankly malignant tumors. The most common pleural tumor (solitary fibrous tumor of the pleura) can occur in either a benign or a malignant form; and they may also recur in either a benign or a malignant form.98,102
Benign Pleural Tumors That May Remain Benign
Solitary Fibrous Tumors
The solitary fibrous tumor is the most common benign pleural tumor, and it is rare (by 2002, only 800 cases had been reported).98 (In contrast, 3000 new cases of diffuse mesothelioma are diagnosed yearly in the United States.103) This tumor arises from the submesothelial layer of the mesothelioma and it is usually solitary, although it may occur in multiple sites.104,105 It is associated with hypertrophic pulmonary osteoarthropathy in up to 22% of cases, and with severe hypoglycemia in 3% to 4% of cases.106 The latter syndrome (Doege-Potter syndrome) is attributable to tumor-derived growth factor (insulin-like growth factor II).107
These tumors are often (>80%) attached to the visceral pleura, and they are often pedunculated (80%). In the remaining 20% of cases, they originate from the parietal pleura on the diaphragmatic or mediastinal surface, and parietal-based tumors are more likely to be sessile than pedunculated.105 Pedunculated tumors have a 2% recurrence rate, whereas sessile tumors have an 8% recurrence rate.98
Because the solitary fibrous tumor is often pedunculated, it is amenable to resection by video assisted thoracic surgery (VATS). However, resection should be complete and without tumor disruption to prevent tumor recurrence from either incomplete resection or microscopic seeding of the resected bed. It is important to remove not only the tumor but also the stalk with a 1-cm base of underlying lung. If the tumor is too large for VATS surgery, a thoracotomy may be required.98,100 Tumors originating from the parietal pleura require at least a partial pleurectomy with the tumor resection. Occasionally, chest wall resection along with tumor removal is required if there is concern about recurrence or invasion from possible malignant transformation.
Sessile lesions larger than 5 cm should probably be removed by thoracotomy to prevent recurrences.100 These tumors may appear to invade the lung if visceral growth (inverted fibroma) is seen, so lung resection may be required. Peritumoral adhesions, in which microscopic tumor deposits may hide, are common (60%), so a wide resection is needed. This can include lung, diaphragm, chest wall, and pericardium with frozen section determination that all margins are free of tumor at resection.100 Recurrent tumors may be multifocal and may undergo malignant transformation.102 Histologic characteristics do not always predict the potential for recurrence, necessitating long-term follow-up.
Lipomas and Lipoblastomas
Chest wall lipoma is a common incidental finding, occurring in 0.1% of all computed tomography (CT) scans of the chest.108 Lipoblastoma is a tumor of embryonic white fat that typically occurs in infancy or early childhood.109 Both lipomas and lipoblastomas are benign and are best treated by surgical excision of the involved area.
Adenomatoid Tumors
Adenomatoid tumors are very rare incidental findings appearing as solitary circumscribed lesions on either the parietal or visceral pleura. They are usually identified at the time of pulmonary resection for unrelated pathology. They occur more commonly in other sites (most commonly the genital tract) and resection is performed to exclude other malignant pleural tumors.110
Calcifying Fibrous Tumors
Calcifying fibrous tumors are rare and are characterized by dense collagenous tissue with psammoma bodies containing dystrophic calcifications. They commonly occur in subcutaneous tissue or in deep soft tissue, but rarely in the pleura (there have been nine reports of this tumor since the initial report in 1996). They can occur as solitary tumors or in multiple sites; calcifications are typically not seen on plain chest films but are seen on a CT scan.111–118
Simple Mesothelial Cysts
The pleura is an uncommon site for the development of a mesothelial cyst, which is thought to arise from persistence of the ventral pericardial recess after embryonic development. These lesions often occur in the anterior right cardiophrenic angle. The cysts that retain a connection to the pericardium are called pleuropericardial cysts, and if the neck to the pericardium is pinched off they are classified as mesothelial cysts. They can be treated with surveillance unless symptoms develop, and then they can be treated by either aspiration or excision by VATS.119,120
Multicystic Mesothelial Cysts
Multicystic inclusion cysts (multicystic mesothelioma or multilocular inclusion cysts) appear as multilocular fluid-filled cysts spread along the serosal surface of the pleura. There are extremely rare abnormalities occurring in asymptomatic individuals, and their removal to exclude other pathologies can be accomplished by VATS surgery.121
Schwannoma
Schwannomas are peripheral nerve sheath tumors that may occur spontaneously or after radiotherapy.122 They may arise in paraspinal intrathoracic locations and may mimic loculated fibrous deposits or localized pleural tumors. The key to the removal of this tumor is to exclude the possibility of spinal extension to prevent paraplegia from compressive spinal cord hematoma formation caused by bleeding into the peritumoral area.
Pleural Tumors with Low Malignant Potential
Well-Differentiated Papillary Mesothelioma
Well-differentiated papillary mesothelioma typically occurs with a diffuse nodular growth over the mesothelial surface, although there is only superficial invasion without deep invasion except in recurrent or long-standing lesions. Diagnosing this lesion is difficult because it looks similar to invasive mesothelioma (diffuse malignant mesothelioma) on small biopsy specimens. These lesions have an indolent course in the absence of invasive features, and in most instances they do not shorten life expectancy.123
Desmoid Tumors
Desmoid tumors, which usually arise from facial or musculoaponeurotic structures, commonly arise from the chest wall with visceral invasion, although pleural desmoid tumors have been documented.124,125 They may be associated with aggressive fibromatosis via the APC tumor suppressor gene or the β-catenin oncogene acting through the main intracellular effector pathway of the Wingless/Wnt signaling pathway.126,127
The treatment of these tumors requires complete resection with negative margins,124,125,128 although recurrence may occur even with negative margins.125 However, surgical resection of recurrences has excellent potential for long-term cure.128
Pleural Thymoma
Pleural thymomas may arise from the pleura and can appear in either a localized or diffuse form. They probably arise from ectopic thymic tissue in the pleura, although this occurrence is rare (only 15 cases reported by 2002).129 The localized form can be surgically removed for cure; the diffuse form may require radiotherapy.123,130
Primary Malignant Pleural Tumors That May Look Like Benign Tumors
Malignant Solitary Fibrous Tumor
Patients with this tumor, which arises from mesenchymal cells in the submesothelial layer with malignant degeneration, are more likely to present with larger, symptomatic, sessile tumors than their benign pedunculated counterparts.98,99,131 Visceral-based tumors are less likely to be malignant,132 and they are more often pedunculated than the parietal-based tumors.98,100 Malignancy is also more often associated with CT heterogeneity of these tumors, pleural effusions, chest wall invasion, and positivity with positron emission tomography (PET).99,100 CT-guided aspiration to determine malignancy is notoriously inaccurate,99,100 possibly because the tumor heterogeneity results in sampling error.
After tumor removal, malignancy is determined on the basis of cellularity and high mitotic counts, nuclear pleomorphism, presence of areas of tumor necrosis, and stromal or vascular invasion. Malignancy occurs in approximately 37% of case series of solitary fibrous tumors (range, 7% to 60%).100 Recurrence is usually local and strongly associated with malignancy. It occurs with the following frequencies: benign pedunculated, 2%; benign sessile, 8%; malignant pedunculated, 14%; malignant sessile, 63%.98
Benign tumors may also recur as malignant tumors,102,133 indicating the need for long-term follow-up. All tumors and recurrences are best treated with aggressive surgical resection or extended resection if necessary.98,100 The value of adjuvant therapy for malignant tumors is debated.98,100,131
Pleuropulmonary Blastoma
Pleuropulmonary blastoma (PPB) arises from either the lung or the visceral pleural lining and affects children less than 6 years of age. This tumor is unique in its progression from a cystic stage (type I, multilocular cystic tumors), to a more aggressive stage (type II, mixed cystic and solid tumors), and finally to the most aggressive stage (type III, solid tumor). Age correlates with the type of PBB: younger patients (median age, 9 months) tend to have type I, whereas older patients (median age, 42 months) tend to have type III.134 There is strong evidence for sequential progression of tumor from type I to type II to type III, and recurrences after surgical removal of type I tumors always arise as type II or III tumors, with a low salvage rate.135 The survival rate after successful resection of type I tumors is 85% to 90%, but it drops to 60% for type II and 45% for type III tumors.134,136 Important treatment points concerning this tumor are a thorough histologic review of any multiloculated pediatric lung cyst, continued CT surveillance of these patients, and consideration of adjuvant chemotherapy for resected type I tumors.136
Localized Malignant Mesothelioma
Localized malignant mesothelioma is a rare tumor: by 2007, only 46 cases had been reported in the English literature.137 By all histologic, immunohistochemical, and ultrastructural criteria, these tumors are similar to diffuse malignant mesothelioma.138 Their diagnosis depends on the following factors: (1) radiologic, surgical, or pathologic evidence of a localized serosal or subserosal tumor mass without evidence of diffuse serosal spread, and (2) a microscopic pattern identical to that of diffuse malignant mesothelioma.139 The small number of case series in the literature do not show it to have a strong association with asbestos exposure.137 Recurrence after surgical resection may occur either locally or with distant metastases, although only rarely does diffuse pleural spread occur with recurrence, and, in contradistinction to diffuse malignant mesothelioma, many cases are cured by surgical excision.137,138
Vascular Sarcoma
The two types of pleural vascular sarcomas are epithelioid hemangioendothelioma and angiosarcoma, and the former has a lesser histologic grade. Both may demonstrate smooth to nodular pleural thickening resembling diffuse malignant mesothelioma. Differentiating these vascular sarcomas from mesothelioma can be done by immunostaining for vascular markers (CD34, CD31, factor VIII).123,138 Both types are very aggressive, and the outcome is often poor despite aggressive treatment.140
Liposarcoma
Liposarcomas are rare (15 cases in the English literature to 2007). They are thought to be derived from residual nests of primitive mesenchymal cells in the pleural cavity. Surgical resection with consideration of postoperative chemotherapy is recommended for these tumors.123,141
Pleuropulmonary Synovial Sarcoma
Pleuropulmonary synovial sarcoma is a distinct histologic subtype of sarcoma that is identified as a mesenchymal spindle cell tumor with a specific chromosomal translocation of t(X;18)(p11.2;q11.2). The name is a misnomer, as these tumors have no relationship to synovial tissue. They are thought to derive from totipotential mesenchymal cells with epithelial differentiation. The majority of these tumors are large and pleural based with heterogeneous areas of necrosis or hemorrhage; it is important to differentiate these rare primary pleural tumors from the more frequently seen metastatic synovial sarcoma to the lungs.142 Only a few anecdotal reports appear in the literature with respect to the treatment of this tumor. All include surgical resection and adjuvant therapy. Chemotherapy or radiotherapy may be added to the treatment regimen, based on soft tissue synovial sarcoma experiences and poor anecdotal results with this tumor in thoracic locations.142,143
Askin Tumor or Primitive Neuroectodermal Tumor (PNET)
The Askin tumor, or primitive neuroectodermal tumor (PNET), is a soft tissue tumor that appears either as a single lesion or as multiple pleural nodules in children and young adults. It is classified as a member of the Ewing’s sarcoma family because the chromosomal aberration t(11;22)(q24;q12) is peculiar to both tumors. However, PNET originates from soft tissue, whereas Ewing’s sarcoma is a tumor of bone. Extrapolated data from a single-center report of treatment of PNET144 or the combined results of both tumors145 suggest consideration of neoadjuvant chemotherapy, surgical resection, and adjuvant chemoradiotherapy.
Desmoplastic Small Round Cell Tumor
The desmoplastic small round cell tumor is a rare aggressive malignancy of adolescents and young men; fewer than 10 thoracic cases have been described.95,146,147 These tumors may remain localized, but more commonly they involve the pleura diffusely. They have a unique expression of epithelial, muscle, and neural markers, with a characteristic fusion protein as a result of gene fusion transcript between the Ewing’s sarcoma gene on chromosome 22 and the Wilms’ tumor gene on chromosome 11, t(11;22)(p13;q22).123,138 The prognosis is poor, as it is in patients with abdominal presentations of this tumor.
Malignant Pleural Tumors
Metastatic Malignant Tumors
Metastatic tumors to the pleura are common (>150,000 new cases per year in the United States); the tumor of origin in 75% of cases is lung, breast, or lymphoma, with minor contributions from ovary and stomach. In 5% to 10% of cases, the primary is unknown.148,149 Patients with metastatic pleural tumors often present with symptoms from a pleural effusion because of lymphatic obstruction of pleural fluid or because of increased capillary permeability in response to tumor invasion, either by direct local tumor extension or by tumors (ovary, breast) with a predilection for serosal invasion by a hematogenous route.150 Examples of secondary pleural effusions (paramalignant effusions) caused by tumor-associated effusions without direct neoplastic involvement of serosal surfaces are chylothoraces, proximal bronchus obstruction with postobstructive atelectasis, and effusions resulting from cancer cachexia.149 Metastatic tumors to serosal surfaces resulting in effusions develop site-based cellular differences between effusion tumor cells and cells from the primary or other metastatic sites. Such differences include upregulation of matrix metalloprotein (MMP)-2, and other adhesion molecules (cadherins, integrins), reduced reliance on ERK-driven pathways or chemokines for proliferation, and apoptosis resistance despite increased death receptor expression and in accordance with increased inhibitors of apoptosis proteins.150
Determination of malignancy by cytologic examination of pleural effusions ranges from 62% to 90% of patients with malignant pleural effusions; increased test sensitivity can be achieved by repeated thoracocenteses, electro-chemiluminescence immunoassay, genetic analysis by microsatellite analysis, DNA methylation, determination of aneuploidy, and immunocytochemistry by a variety of techniques.151 Gene expression tests such as EGFR mutation and receptor analysis can be useful both for tumor differentiation and for response to treatment.152 Malignant pleural effusions can be managed by a variety of techniques with a goal of creating a pleural symphysis. Such techniques range from a variety of catheter drainage procedures with the option of several different sclerosing agents, to pleuroperitoneal shunting or a surgical pleurectomy and decortication.148,149,151,153,154
Restraint with respect to aggressive surgical strategies is appropriate, because, apart from breast cancer, the median survival from malignant pleural effusions is less than 6 months.155 Massive effusions fare worse than lesser effusions.156
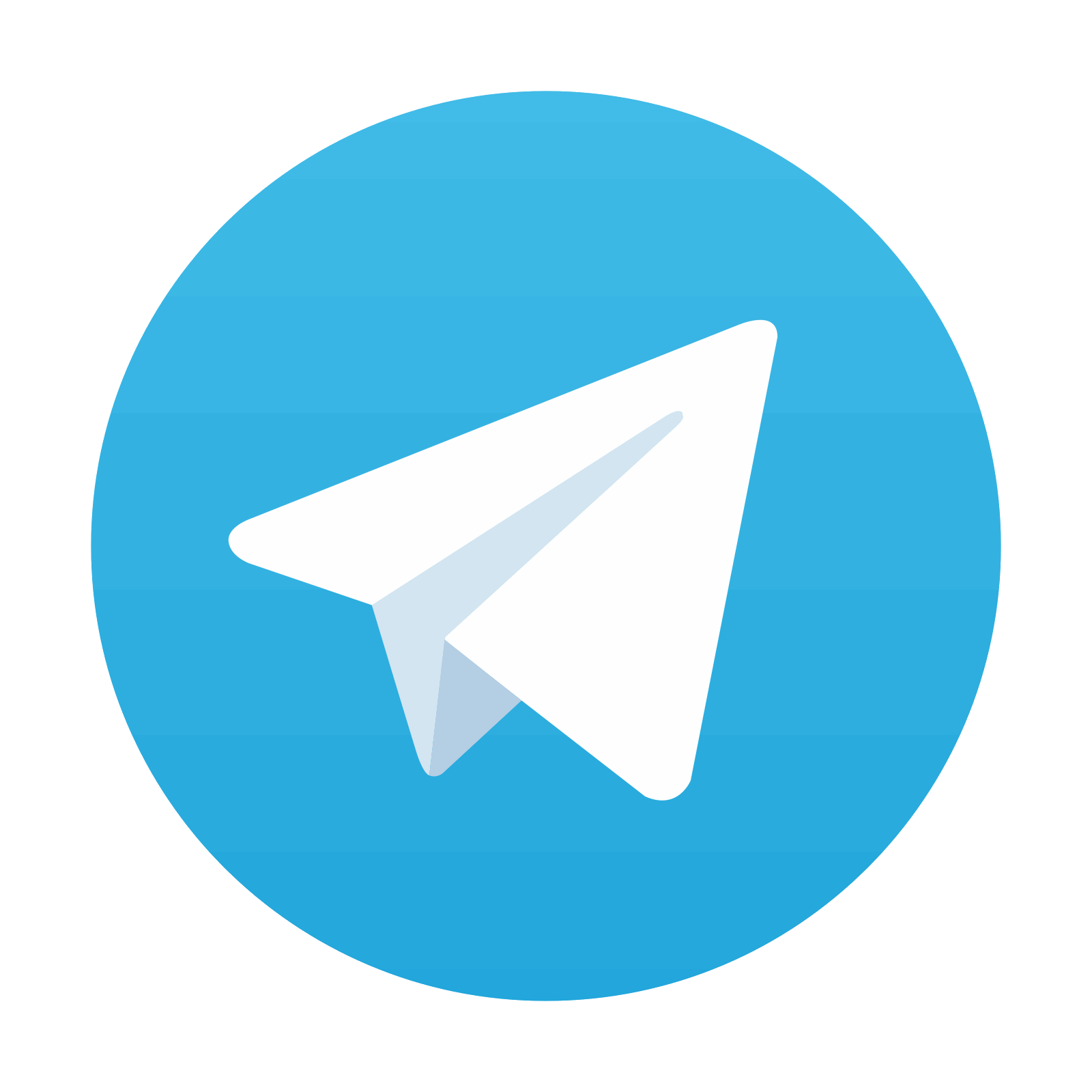
Stay updated, free articles. Join our Telegram channel

Full access? Get Clinical Tree
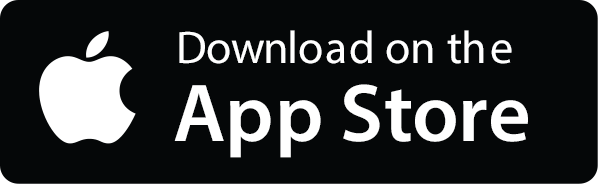
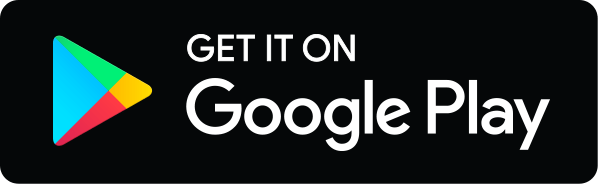