Physiology of Pleural Fluid Production
Richard W. Light
The author22 has estimated that more than 1 million cases of pleural effusion occur annually in the United States. The possibility of a pleural effusion should be considered whenever a patient with an abnormal chest radiograph is evaluated. Increased densities on chest radiography are frequently attributed to parenchymal infiltrates when they actually represent pleural fluid. Free pleural fluid is best demonstrated with ultrasonography, computed tomography (CT), or lateral decubitus radiography. If, on one of these studies, the distance between the inside of the chest wall and the outside of the lung is greater than 10 mm and the etiology of the effusion is unclear, a diagnostic thoracentesis is indicated. If this distance is less than 10 mm, the pleural effusion is probably not clinically significant and a thoracentesis is not indicated. The presence of loculated pleural fluid is best demonstrated with ultrasonography.
Formation and Resorption of Pleural Fluid
Pleural fluid has several possible origins. It can originate in the capillaries in the parietal or visceral pleura. It can come from the interstitial spaces of the lung, or it can come from the peritoneal cavity through small holes in the diaphragm. Wiener-Kronish and coworkers34 have reported that the rate of entry of fluid into the pleural space in normal individuals is approximately 0.01 mL/kg per hour. It can also come from disruption of the blood vessels in the chest, producing a hemithorax or from disruption of the thoracic duct, producing a chylothorax.
Capillary Origin
The movement of fluid across the pleural membranes is believed to be governed by Starling’s law of transcapillary exchange. When this law is applied to the pleura:

where Qf is the liquid movement; Lp is the filtration coefficient per unit area or the hydraulic water conductivity of the membrane; A is the surface area of the membrane; P and π are the hydrostatic and oncotic pressures, respectively, of the capillary (cap) and pleural (pl) space; and σd is the solute reflection coefficient for protein, a measure of the membrane’s ability to restrict the passage of large molecules. Kinasewitz and colleagues16 have estimated that σd is approximately 0.80 in humans.
Estimates of the magnitude of the pressures affecting fluid movement in and out of the pleural space are shown in Figure 59-1. When the parietal pleura is considered, a gradient for fluid filtration is normally present. The hydrostatic pressure in the parietal pleura is approximately 30 cm H2O, whereas the pleural pressure is approximately -5 cm H2O. The net hydrostatic pressure gradient is therefore 30 – (-5) = 35 cm H2O and favors the movement of fluid from the capillaries in the parietal pleura to the pleural space. Opposing this hydrostatic pressure gradient is the oncotic pressure gradient. The oncotic pressure in the plasma is approximately 34 cm H2O. Because the oncotic pressure of the small amount of pleural fluid is approximately 5 cm H2O, the net oncotic gradient is 34 – 5 = 29 cm H2O. Thus, the net gradient is 35 – 29 = 6 cm H2O, favoring the movement of fluid from the capillaries in the parietal pleura to the pleural space. In normal humans, the parietal capillaries are thought to be the origin for most pleural fluid, which is estimated to be 0.01 mL/kg per hour.22
Albertine and coworkers3 have shown that the blood supply to the visceral pleura in humans is from the bronchial artery rather than the pulmonary artery. The net gradient for fluid movement across the visceral pleura in humans is probably close to zero. The pressure in the visceral pleural capillaries is approximately 6 cm H2O less than that in the parietal pleural capillaries because the former drain into the pulmonary veins. Because this is the only pressure that differs from those affecting fluid movement across the parietal pleura and the net gradient for the parietal pleura is 6 cm H2O, it follows that the net gradient for fluid movement across the visceral pleura is approximately zero. It is also quite likely that the filtration coefficient (Lp) for the visceral pleura is substantially less than that for the parietal pleura, because Albertine and coworkers2 have shown that the capillaries in the visceral pleura are much farther from the pleural space than are those in the parietal pleura.
Interstitial Origin
Convincing evidence has been presented that the origin of a substantial percentage of pleural fluid is the interstitial spaces
of the lung. Broaddus and coworkers8 have demonstrated that when sheep, a species with a pleura anatomically similar to that of humans, were volume overloaded by administration of 20% of their body weight as Ringer’s lactate, transudative liquid flowed across the visceral pleura of isolated, in situ lungs. The pleural fluid contained the same protein concentration as did the lung lymph and the interstitial edema liquid in the lung. The volume of pleural fluid constituted approximately 25% of all edema formed in the lung. In other experiments, Allen and colleagues4 have shown that with high-pressure pulmonary edema in sheep, pleural fluid accumulates only after pulmonary edema develops. In the clinical situation, Wiener-Kronish and coworkers35 have shown that in patients with congestive heart failure, the presence of pleural effusions on ultrasonography correlates more closely with the pulmonary venous pressure than with the systemic venous pressure and that the likelihood of pleural effusions increases as the severity of the pulmonary edema on chest radiography increases.
of the lung. Broaddus and coworkers8 have demonstrated that when sheep, a species with a pleura anatomically similar to that of humans, were volume overloaded by administration of 20% of their body weight as Ringer’s lactate, transudative liquid flowed across the visceral pleura of isolated, in situ lungs. The pleural fluid contained the same protein concentration as did the lung lymph and the interstitial edema liquid in the lung. The volume of pleural fluid constituted approximately 25% of all edema formed in the lung. In other experiments, Allen and colleagues4 have shown that with high-pressure pulmonary edema in sheep, pleural fluid accumulates only after pulmonary edema develops. In the clinical situation, Wiener-Kronish and coworkers35 have shown that in patients with congestive heart failure, the presence of pleural effusions on ultrasonography correlates more closely with the pulmonary venous pressure than with the systemic venous pressure and that the likelihood of pleural effusions increases as the severity of the pulmonary edema on chest radiography increases.
Exudates found in association with increased permeability pulmonary edema probably also originate from the lung interstitium. When Wiener-Kronish and associates36 induced increased permeability edema in sheep by the infusion of oleic acid, pleural fluid accumulated only after pulmonary edema developed. In this report, no detectable injury to the visceral pleura was seen by morphologic studies. They found that approximately 20% of the excess lung liquid that formed after oleic acid–induced lung injury was cleared from the lung through the pleural space. It has also been shown that the high-protein pleural effusion that develops in rats after the administration of xylazine has its origin in the interstitial spaces of the lung,5 as did the exudative pleural effusions induced by hypoxia in rats.6
Peritoneal Origin
Fluid that is free in the peritoneal cavity can move directly into the pleural space if the diaphragm has holes in it. This mechanism is responsible for the pleural effusions that occur in conjunction with cirrhosis and ascites, pancreatic ascites, Meigs’ syndrome, and peritoneal dialysis.
Lymphatic Clearance
Figure 59-1 might lead one to conclude that pleural fluid should continuously accumulate because Starling’s equation favors fluid formation through the parietal pleura and no gradient exists for fluid absorption through the visceral pleura. Fluid clearance via the pleural lymphatics is thought to explain the lack of fluid accumulation in the normal individual. Stewart31 demonstrated that proteins, cells, and all other particulate matter are removed from the pleural space by lymphatics in the parietal pleura. Wang33 demonstrated that, at least in rabbits, the pleural space is in communication with the lymphatic vessels by means of stomas located within the parietal pleura. No such stomas are present in the visceral pleura. Although these stomas have been more difficult to demonstrate in humans, Li21 has demonstrated the presence of stomas in the diaphragmatic parietal pleura but not in the thoracic parietal pleura or the visceral pleura. The mean diameter of the stomas is 6.2 μm, and there are great numbers of microvilli on the mesothelial cells surrounding their openings.21
Broaddus and coworkers7 produced artificial hydrothoraces in awake sheep by injecting an autologous protein solution at a volume of 10 mL/kg with a protein level of 1.0 g/dL. They found that the hydrothorax was removed almost completely by the lymphatics in a linear fashion at a rate of 0.28 mL/kg per hour. This linearity suggests that the lymphatics operate at maximum capacity once the pleural liquid exceeds a certain threshold volume. Note that the capacity for lymphatic clearance is 28 times the normal rate of pleural fluid formation.
In the aforementioned experiments,7 the fluid that was introduced into the pleural space had an oncotic pressure of approximately 5 cm H2O. From Figure 59-1, one might speculate that if fluids with oncotic pressures other than 5 cm H2O were introduced, the equilibrium would be altered such that fluid would enter the pleural space from visceral pleura in animals with high pleural fluid oncotic pressures and would leave the pleural space through the visceral pleura in animals with low pleural fluid oncotic pressures. This does not appear to be the case. Aiba and coworkers1 produced artificial pleural effusions in dogs with protein levels ranging from 0.1 to 9.0 g/dL. Even when the induced pleural effusion had an oncotic pressure of 0.1 g/dL, no increase in the concentration of protein occurred with time, indicating that the low oncotic pressure did not induce a rapid efflux of fluid out of the pleural space. When the protein concentration of the induced effusions was greater than 4 g/dL, the concentration of protein in the pleural fluid did decrease with time, indicating a net transfer of protein-free fluid into the pleural space. However, the net amount of fluid entering the pleural space, even with a protein level of 9.0 g/dL, was only 0.22 mL/kg per hour. This degree of fluid flux is similar to the lymphatic clearance of 0.22 mL/kg per hour reported in the same studies. These observations explain why protein levels and hematocrits remain relatively stable in individuals with hemothoraces.
The amount of fluid that can be cleared through these lymphatics is substantial. Stewart31 found that the mean lymphatic flow from one pleural space in seven patients was 0.40 mL/kg per hour, whereas Leckie and Tothill19 found that the mean lymphatic flow was 0.22 mL/kg per hour in seven patients with congestive heart failure. In both these studies, marked variability was noted from one patient to another. If these results from
patients with congestive heart failure can be extrapolated to the normal person, a 60-kg individual should have the capacity to absorb approximately 20 mL/hr or 500 mL/day from each pleural space through the lymphatics.
patients with congestive heart failure can be extrapolated to the normal person, a 60-kg individual should have the capacity to absorb approximately 20 mL/hr or 500 mL/day from each pleural space through the lymphatics.
In summary, if the experimental results in sheep can be extrapolated to humans, it appears that a small amount (0.01 mL/kg per hour) of fluid constantly enters the pleural space from the capillaries in the parietal pleura. Almost all of this fluid is removed by the lymphatics in the parietal pleura, which can remove approximately 0.20 mL/kg per hour. Little net fluid movement occurs across the visceral pleura. A pleural effusion develops when the amount of fluid that enters the pleural space exceeds the amount that can be removed via the lymphatics. Accordingly, pleural effusions can develop and are caused by increased pleural fluid formation, decreased lymphatic clearance from the pleural space, or a combination of these two factors. The three primary origins of pleural fluid are the pulmonary interstitial spaces, pleural capillaries, and peritoneal cavity.
Differential Diagnosis
Pleural effusions can occur as complications of many different diseases and therapeutic procedures (Table 59-1). The initial step in evaluation of the patient is the differentiation between exudative and transudative effusions.
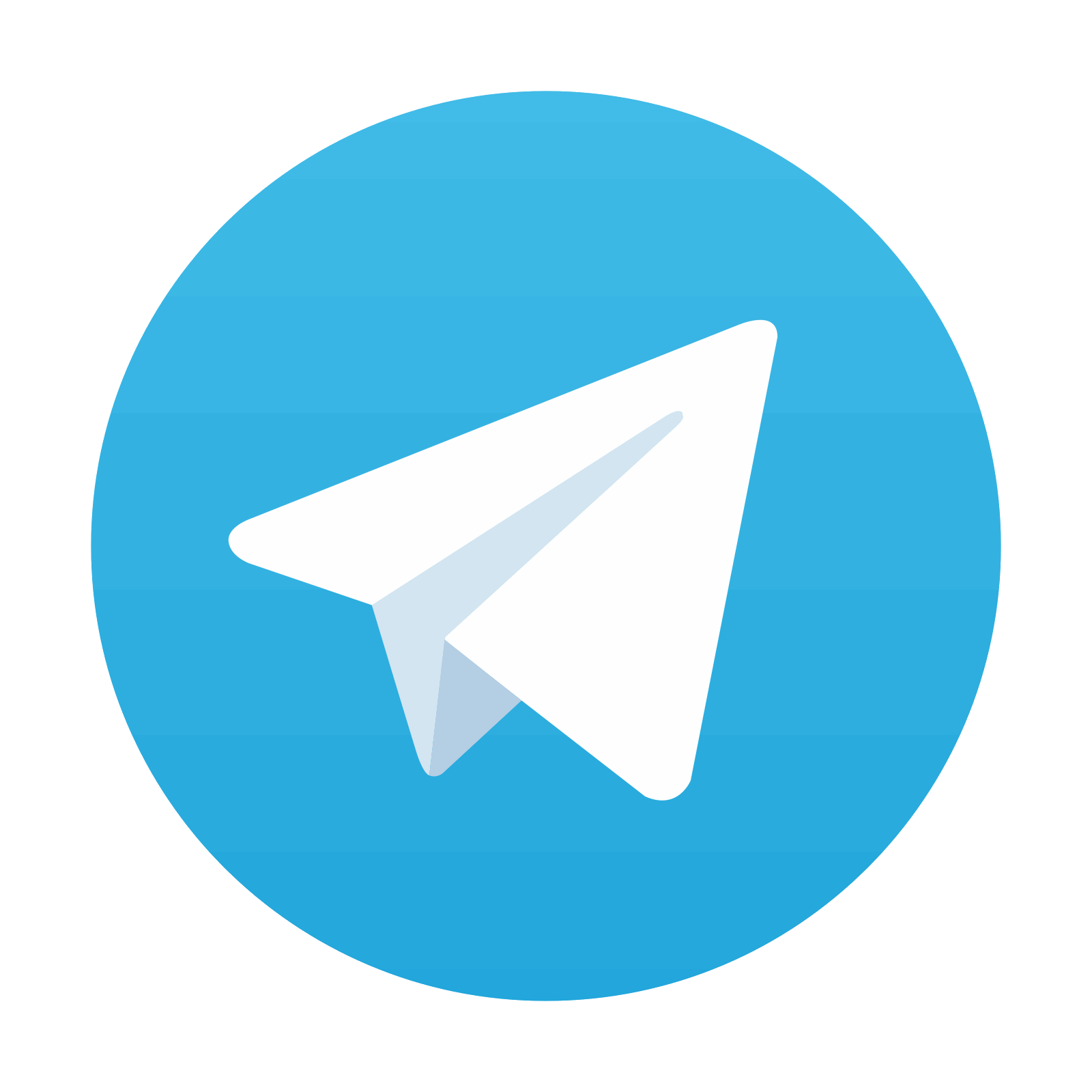
Stay updated, free articles. Join our Telegram channel

Full access? Get Clinical Tree
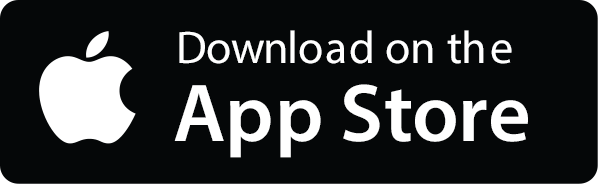
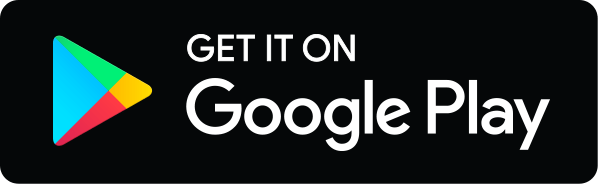