Pulmonary hypertension exists as a pathologic finding in newborn infants in several clinical settings. In the early newborn period, the syndrome of persistent pulmonary hypertension of the newborn (PPHN) can occur, consisting of failure of the fetal circulation to adapt to postnatal newborn physiology, mainly because of a persistent elevation in pulmonary vascular resistance. PPHN can occur as a primary pathology or secondary to pulmonary diseases such as meconium aspiration syndrome, pneumonia, pulmonary hypoplasia, or pulmonary/intrathoracic anomalies. Pulmonary hypertension can also occur after the early newborn period as a consequence of chronic pulmonary diseases such as bronchopulmonary dysplasia, or in association with congenital heart disease. At a physiologic level, pulmonary hypertension can be caused by increased pulmonary vascular resistance, by increased pulmonary blood flow, or by obstruction to pulmonary venous drainage. While the measurements of pulmonary artery pressure are similar no matter what the cause, some of the associated echocardiographic findings and the clinical scenarios will differ. The primary problem might not be easily discernible by echocardiography alone. However, assessment of the hemodynamics can often assist the clinician in choosing appropriate therapies.
Understanding the physiology of normal transition and of pulmonary hypertension is essential for interpreting the echocardiographic findings. Fetal circulation is characterized by high pulmonary vascular resistance and low pulmonary blood flow. Pulmonary pressures in the fetus are higher than those of the post-transitional newborn circulation. The normal transitional circulation involves a decreasing pulmonary vascular resistance and pulmonary artery pressure over the first 3 days of life. In a study of normal-term infants catheterized in the first 3 days of life, peak systolic pulmonary pressures were approximately equal to systemic pressures in the first 10 hours of life. Pulmonary pressures were lower in infants with increasing age over the first 3 days of life.1 PPHN was previously referred to as persistent fetal circulation (PFC) because the circulatory pattern does not transition from the fetal to newborn pattern along the normal timeline, and the baby becomes hypoxemic.
It is important to understand the expected findings in normal transition in order to interpret the echocardiogram in the clinical setting of PPHN. The physiology of PPHN includes elevated pulmonary pressures and decreased pulmonary blood flow due to high pulmonary vascular resistance. A higher force from the right ventricle is required to overcome the resistance of the pulmonary vessels. With decreased pulmonary blood flow, there will be decreased pulmonary venous return to the left atrium and therefore decreased left ventricular preload. Because of the increased pressure in the right ventricle, the interventricular septum can be pushed toward the left, potentially impairing function of the left ventricle. The systemic blood flow can be decreased as a result of the combination of these factors. The echocardiogram can be used to estimate the pulmonary artery pressure, provide information about the relative pulmonary to systemic pressure gradients, and evaluate the overall hemodynamic consequences. The echocardiogram is also critical in differentiating the diagnosis of pulmonary hypertension from other causes of cyanosis.
In this chapter, the specific echocardiographic findings in pulmonary hypertension compared to the normal newborn is reviewed. Though each measure is discussed individually, the clinician must look at all of the information from the echocardiogram (including anatomy and function) and interpret the imaging data in conjunction with the clinical information, in order to make the best management decisions.
Pulmonary artery pressure can be estimated by echocardiography in several ways. The most direct and accurate measure of systolic pulmonary artery pressure uses the tricuspid regurgitation (TR) jet. Tricuspid regurgitation occurs because the tricuspid valve is not able to contain all of the blood in the right ventricle when under pressure during systole. The speed with which blood escapes the right ventricle through the tricuspid valve can be measured by Doppler echocardiography. The modified Bernoulli equation is used to estimate the pressure difference between two chambers from the velocity of blood flow between the two chambers:
where P = pressure, v = velocity (measured by Doppler).
Since the modified Bernoulli equation estimates the pressure difference between the right ventricle (RV) and right atrium (RA), an estimate of right atrial pressure must be added to this value in order to determine an estimate of the pulmonary artery pressure.2,3 Since RA pressure is not actually measured, a factor of 5-10 mmHg is often used as an estimate. It is important to understand that the estimation of pulmonary artery pressure from the tricuspid regurgitation jet assumes that the pressures in the pulmonary artery (PA) and right ventricle are equal. This is only true when there is no obstruction between the RV and PA. Therefore, to interpret the significance of the TR jet, one must know that the anatomy at the pulmonary valve and right ventricular outflow tract is normal. The tricuspid regurgitation jet is not always present or measurable when the pulmonary pressure is elevated. In one reported series of infants with PPHN, the TR jet was measurable in 70 to 80% of infants studied.4,5 When present, it provides one of the most accurate and repeatable noninvasive measures of pulmonary artery pressure.6,7
Tricuspid regurgitation can be imaged from several views. It is often easily identified and best measured from either the parasternal long-axis right ventricular inflow view (Figure and Video 12-1) or the apical four-chamber view (Figure and Video 12-2). The tricuspid regurgitation jet is identified with color flow Doppler, and the velocity is then measured by positioning the continuous-wave Doppler sample in line with the jet. Continuous-wave Doppler is usually necessary because the TR jet velocity will be too high to be measured with pulsed wave Doppler. Since the TR jet can be multidirectional, the Doppler sampling beam might need to be maneuvered into an optimal location. For an accurate measurement of velocity, the spectral Doppler signal should be complete throughout the systolic phase of the cardiac cycle and should reach a clear peak. An example of a complete Doppler signal is shown in the last cycle of the tracing in Figure 12-3. In contrast, when the complete Doppler signal is not obtained, the velocity will be falsely low. An example of an incomplete Doppler signal is shown in Figure 12-4.
Tricuspid regurgitation has been evaluated in healthy term and preterm infants in the first days of life.8 In a group of healthy transitioning infants, all preterm infants had some identifiable TR in the first three days of life, half of which were measurable. The TR was no longer seen in these healthy infants after 72 hours of life. About 70% of term infants had some identifiable TR during this period, but only 20 to 30% were measurable. In a comparison between healthy infants and infants with respiratory distress syndrome (RDS), those with RDS were more likely to have TR present at 72 hours of life and had higher estimates of pulmonary artery pressure between 12 and 48 hours of life.9
The pulmonary artery pressure can also be estimated from the Doppler examination of the patent ductus arteriosus (PDA). If the PDA flow is bidirectional, the relative difference between the systemic and pulmonary pressures can be estimated by the percentage of time that blood is flowing right to left compared with the entire cardiac cycle length. If the right-to-left component of a bidirectional shunt is greater than 60% of systole or greater than 30% of the total cycle, the peak pulmonary artery pressure is likely to be suprasystemic.10 Figure 12-5 shows a pulsed wave Doppler tracing of a bidirectional PDA with the right-to-left component that is approximately 30% of the cycle. Figure and Video 12-6 shows the color Doppler image of this PDA. Note that it is difficult to see the blue (right-to-left) component when the video is playing at full speed. Slowing the video down and reviewing it frame by frame allows for identification of blue followed by red flow patterns. The small left-to-right component of the shunt can also be visualized by spectral Doppler.
An estimate of the pulmonary artery pressure can also be determined by measuring the peak velocity of the PDA flow. Once the peak velocity of the PDA flow is measured, the pressure difference between the aorta and pulmonary artery can then be calculated from the modified Bernoulli equation. With pure left-to-right PDA flow, the systemic systolic pressure minus the pressure difference between the aorta and the pulmonary artery (as calculated by Doppler velocity) will be an estimate of the systolic pulmonary artery pressure. Figure 12-7 shows a pulsed wave Doppler image of a pure left-to-right PDA. The peak velocity of the left-to-right component is measured at 2.11 m/s, and the pressure difference between the aorta and the pulmonary artery is calculated as 17.75 mmHg (calculated by machine software using the modified Bernoulli equation). The infant’s systemic systolic blood pressure was 68 mmHg. Therefore, the peak pulmonary pressure can be estimated to be 50.25 mmHg. Conversely, with pure right-to-left flow, the systolic systemic blood pressure plus the pressure difference between the aorta and the pulmonary artery will be an estimate of the systolic pulmonary artery pressure. In clinical practice, knowledge about the relative pressure differences between the systemic and pulmonary circulations adds to the overall assessment that a clinician makes when evaluating an infant.
The PDA is best imaged from the suprasternal ductal cut (Chapter 3). Once a good 2D image is obtained (Figure and Video 12-8), color Doppler is applied and a visual assessment of the direction of flow through the PDA can be made. It is generally best to take a clip of the color Doppler imaging and review the clip in a frame-by-frame approach to identify the color at each point in the cardiac cycle. A color signal cycling between blue and red suggests bidirectional shunting, whereas a color signal that incorporates multiple colors in the same stream suggests high-velocity, turbulent flow with color aliasing. Figure and Video 12-9 shows a PDA that is mostly right to left, indicated by the mostly blue color. Intermittently there is a small amount of red seen, indicating the left-to-right portion of the flow. Compare this with Figure 12-6, which shows a bidirectional PDA with more left-to-right flow and Figure and Video 12-10, which shows a completely left-to-right PDA that has many different colors due to constriction resulting in high velocity with color aliasing. With live imaging, once a good color image visualizing the entire length of the PDA has been obtained, pulsed and continuous-wave Doppler evaluation can be performed in line with the PDA to obtain the velocity and pattern of the flow.
In term infants, immediately after birth the direction of PDA shunting is often bidirectional. As discussed previously, values of greater than 30% right-to-left flow throughout the cardiac cycle correlate with significantly elevated pulmonary artery pressure. By 12 hours of life the PDA is completely left-to-right in most healthy-term newborn infants.11 Assessment of the PDA shunt direction and character is helpful to the clinician caring for infants with PPHN, but once the PDA is closed, pulmonary artery pressure can no longer be estimated using these methods.
The clinician should also evaluate the patent foramen ovale (PFO) flow in the assessment of pulmonary hypertension. The best images of the atrial septum can be obtained from the subcostal coronal posterior view with the probe angled slightly posteriorly and superiorly (Chapter 3). Figure and Video 12-11 shows an example of the atrial septum imaged from this window. In this example, the septum is bulging toward the right. Once a good 2D image is obtained, color Doppler should be used to identify flow across the septum. Figure and Video 12-12 shows the color Doppler imaging of this atrial septum. Note that the flow is left-to-right and higher velocity. However, when imaging the atrial septum, the clinician should keep the Doppler scale low enough to identify low-velocity flow. An atrial shunt from the same baby with the scale set lower is shown in Figure and Video 12-13. A pulsed wave Doppler signal at the foramen should also be obtained to best identify the direction of flow. An example of the pulsed wave Doppler signal from the same study is shown in Figure 12-14. The flow through the PFO is frequently bidirectional though predominantly left-to-right in the first 24 hours of life in most term and preterm newborn infants.12,13 However, term infants with pulmonary hypertension have a longer right-to-left component of the shunt throughout the cardiac cycle. Additionally, the bidirectional shunting at the PFO can last for several days in infants with PPHN, even after the PDA closes.12 Changes in direction of flow of both the PDA and PFO can be a useful guide in monitoring a baby’s progress throughout the course of PPHN.
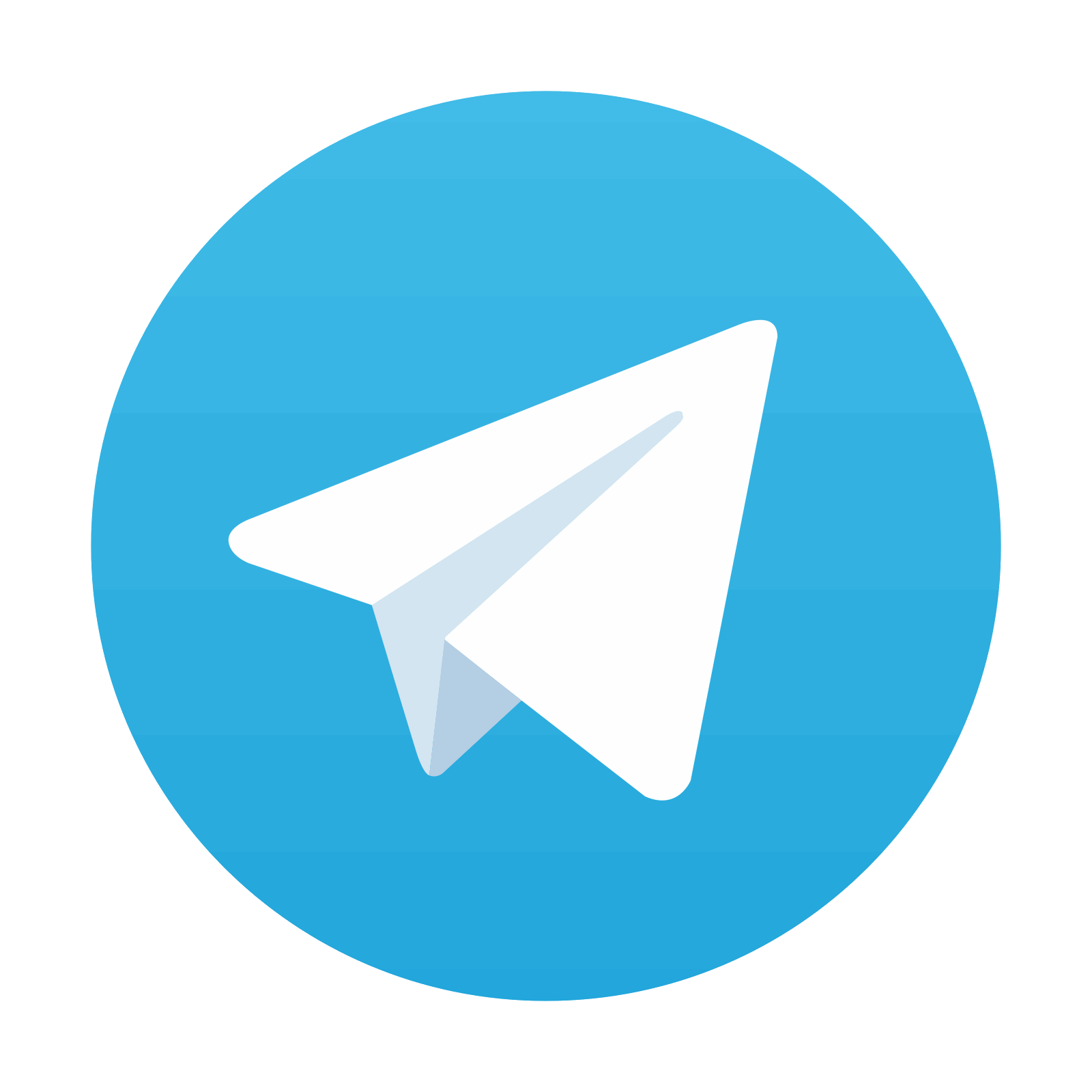
Stay updated, free articles. Join our Telegram channel

Full access? Get Clinical Tree
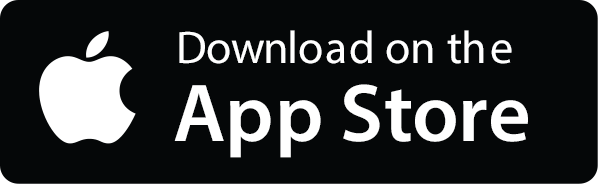
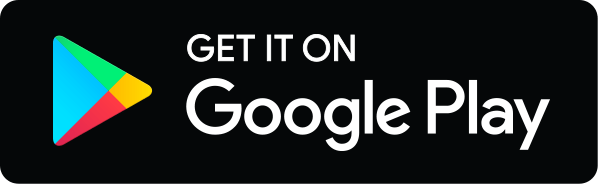