Percutaneous Therapeutic Technologies for Medically Inoperable Lung Cancer
Shawn Haji-Momenian
Ricardo S. Santos
Hiran C. Fernando
Damian E. Dupuy
Lung cancer is the number one cause of cancer death in the United States, accounting annually for approximately 160,000 deaths per year.1 If diagnosed at an early stage, surgical resection of non-small cell lung cancer (NSCLC) offers the best opportunity for local control and survival. However, it is estimated that more than 15% of all patients and 30% of those aged 75 years or older diagnosed with stage I or II NSCLC will be considered medically inoperable.2 The majority of these patients are not surgical candidates because of their poor cardiopulmonary function.
For many years since the first pulmonary resections described by Churchill et al.3 in the early 20th century, pneumonectomy and, subsequently, lobectomy were considered the standard operations for lung cancer. Sublobar resection for stage I NSCLC then emerged as an attractive option, but was subsequently demonstrated to be associated with a threefold increase in local recurrence in the randomized trial conducted by the Lung Cancer Study Group4 during the late 1980s and early 1990s (see Chapters 32 and 33).
Since the publication of that study, most surgeons consider sublobar resections (such as a wedge or segmentectomy) a compromise operation that should be reserved for patients who are felt to be at increased risk for lobectomy, but can still tolerate a smaller pulmonary resection.5,6 With the increasing identification of smaller cancers, particularly with implementation of computed tomography (CT) screening programs, this approach is being challenged, particularly for small stage I cancers. Further discussion of sublobar resection for smalldiameter cancers is found in Chapters 32 and 33.
One approach that has been described to minimize local recurrence when sublobar resection is performed is the use of adjuvant intraoperative brachytherapy.7 Initial results have been encouraging, with a multicenter trial of this technique currently underway. For patients that are considered too high risk for any resection, the standard therapy has been external beam radiation. An alternative to radiotherapy (XRT) is percutaneous image-guided tumor ablation, which has been used for various solid tumors, including liver, kidney, breast, bone, adrenal, and is now proving to be an important tool in the treatment of primary and secondary lung neoplasms. Radiofrequency ablation (RFA) is the current ablative method of choice, although other techniques including microwave ablation (MWA) and cryoablation are also available. In this chapter, we review these alternative therapies that can be used for the high-risk patient with NSCLC.
SUBLOBAR RESECTION AND BRACHYTHERAPY
Higher local recurrence remains the primary problem of sublobar resection for NSCLC. In an effort to improve local control, postoperative external beam XRT following sublobar resection can be used. Miller and Hatcher8 used this approach on a small group of patients who were treated with sublobar resection followed by “postage stamp” external beam radiation. The authors reported a significant decrease in local recurrence compared with an earlier group of patients they had managed with sublobar resection alone.
Unfortunately, this approach is not easy to apply to most of the high-risk patients with NSCLC where sublobar resection is selected. Many of these elderly patients are unwilling to travel for several radiation treatments. Additionally, radiation treatment planning is challenging, because it can be difficult to target small areas around the staple line, particularly if this is in a region of lung that moves with respiration. Radiation pneumonitis is also a potentially life-threatening problem, particularly for patients with impaired pulmonary function, who are the typical candidates referred for these compromise therapies.
An alternative option is the intraoperative placement of 125Iodine (125I) brachytherapy seeds along the staple line after sublobar resection.7,9 This brachytherapy technique utilizes a commercially available vicryl suture that contains 10125I pellets incorporated at 1-cm intervals along the length of the suture (Oncura, Arlington Heights, IL). In the first report describing this technique, these vicryl sutures were placed on a
vicryl mesh. Usually 40 to 60125I pellets with four to five lines of suture material are used with each brachytherapy implant. The total delivered radiation dose to the local tissues (staple line) is usually around 10,000 cGY at a 1-cm distance from the staple line. In essence, this technique effectively extended the margin of resection by another centimeter. The advantage of using intraoperative brachytherapy compared with external beam radiation therapy is that this is a one-time treatment, with 100% patient compliance. There is no burden placed on the patient to return for several radiation therapies after recovering from their operation. Additionally, the radiation is delivered directly at the staple line with minimal injury to surrounding lung parenchyma.
vicryl mesh. Usually 40 to 60125I pellets with four to five lines of suture material are used with each brachytherapy implant. The total delivered radiation dose to the local tissues (staple line) is usually around 10,000 cGY at a 1-cm distance from the staple line. In essence, this technique effectively extended the margin of resection by another centimeter. The advantage of using intraoperative brachytherapy compared with external beam radiation therapy is that this is a one-time treatment, with 100% patient compliance. There is no burden placed on the patient to return for several radiation therapies after recovering from their operation. Additionally, the radiation is delivered directly at the staple line with minimal injury to surrounding lung parenchyma.
An alternative technique to that described previously is to place the125I sutures directly adjacent to the staple line without the use of mesh. This technique of “paired”125I suture lines was described by Lee et al.10 The authors reported the use of brachytherapy “paired-suture line” technique in 33 high-risk patients, primarily after wedge resection. The local recurrence rate was 6.1%, which was similar to the 6.4% reported after lobectomy in the Lung Cancer Study Group study.
A larger study from Pittsburgh utilizing the mesh technique compared the results of sublobar resection with adjuvant 125I brachytherapy in 101 patients with a historical control group of 102 patients who underwent sublobar resection alone; both groups had poor cardiopulmonary function.9 A significant reduction in local recurrence was noticed with the use of brachytherapy (18% vs. 2%). There was no evidence of radiation fibrosis or implant migration among patients receiving125I brachytherapy (Fig. 37.1). Another multicenter study of stage IA patients undergoing lobar and sublobar resection was also reported.11 Included in the study cohort were 124 patients who underwent sublobar resection. Brachytherapy was used in 60 of 124 patients. A decrease in local recurrence from 17.2% to 3.3% was demonstrated in the patients treated with adjuvant brachytherapy. More recently, Birdas et al.12 analyzed the local recurrence, disease-free survival, and overall survival for patients with stage IB cancer who underwent lobectomy (n = 126) versus sublobar resection with brachytherapy (n = 41). Local recurrence was similar between the groups (3% to 5%), with no statistical difference in survival or disease-free interval.
One concern with the technique of brachytherapy is the radiation risk posed to the staff caring for the patient, as well as to the patient’s family. Smith et al.13 have addressed this risk in a prospective study of 22 patients who underwent125I vicryl mesh implantations. Diodes to measure radiation exposure were placed on the back of each hand of the primary radiation oncologist and primary surgeon during the creation and implantation of the mesh. A control reading was obtained by placing diodes on the posterior shoulder of the patient. The authors concluded that there is very little radiation exposure: 1 and 2 mrem/h for the radiation oncologist and surgeon, respectively. Median dose to the control diode on the patient was 5.4 mrem/h. Although the ALARA (as low as reasonably achievable) principle should still be followed, this is a safe method of lung cancer treatment for healthcare professionals. As a precaution beyond state and national radiation guidelines, general recommendations postoperatively for the patients include avoiding close contact with small children or pregnant women for the first 3 months following surgery.
The results of these single-center studies of sublobar resection and brachytherapy have been encouraging. A phase III study of this technique in stage IA NSCLC patients who are at high risk for lobectomy is currently underway by the American College of Surgeons Oncology Group (Z4032). This study will better define the role of adjuvant brachytherapy when sublobar resection is selected for NSCLC.
PERCUTANEOUS IMAGE-GUIDED ABLATION FOR NSCLC
Mechanism Percutaneous image-guided ablation is performed using various thermal energy sources, including radiofrequency (RF), microwave (MW), high-intensity focused ultrasound, laser, and cryoablation to kill tumor cells. These techniques are being applied with increasing frequency for several cancers. The most commonly used ablative modality for NSCLC has been RFA, although some centers are using MWA and cryoablation.
Heat-based ablative methods are effective means of depositing energy into a discrete focus via percutaneously placed electrodes. The volume of heat ablation is dependent on the temperature distribution within the tissue, which often has heterogeneous composition and perfusion. The heat distribution in a target lesion was described by Pennes’ “bioheat”
equation14 in 1948. This equation can be simplified to a first approximation as “necrosis = energy deposited × local tissue interactions — heat loss.”15
equation14 in 1948. This equation can be simplified to a first approximation as “necrosis = energy deposited × local tissue interactions — heat loss.”15
Cellular homeostasis can be achieved with mild temperature elevation of approximately 40°C. Hyperthermic states (42°C to 45°C) cause cellular susceptibility to chemotherapy and radiation therapy.16,17 Cellular death does not occur at these elevated temperatures, however, and continued cellular proliferation has been observed even after prolonged exposure to these temperatures. Long-term exposure to temperatures above 46°C can cause irreversible cellular damage. Increasing the temperature by a few degrees to 50°C to 52°C markedly shortens the time necessary to induce cytotoxicity (down to 4 to 6 minutes).
Between 60°C and 100°C, there is near instantaneous induction of protein coagulation that results in irreversible damage to critical cytosolic and mitochondrial proteins and nucleic acid-histone complexes. Cells experiencing this degree of thermal damage immediately die and undergo coagulative necrosis over the course of several days. Coagulative necrosis denotes irreversible thermal damage and cellular death regardless of the ultimate microscopic findings, which may not meet histologic criteria for coagulation necrosis. Temperatures greater than 105°C to 115°C result in tissue boiling, vaporization, and carbonization.18 These processes limit optimal ablation as a result of the insulating effects of the produced gas, which decreases the amount of energy deposited, and reduces thermal conduction into the lesion. The goal of ablative therapies is to achieve stable temperatures ranging between 60°C and 100°C throughout the entire volume of the lesion.
Technique
Radiofrequency Ablation RFA is currently the most robust technique for the treatment of solid malignancies. RFA has become the ablative method of choice because of its relatively low cost, its capability of creating large regions of coagulative necrosis in a controlled fashion, and its relatively low toxicity. RFA applies to all electromagnetic energy sources with frequencies less than 30 MHz, although most clinically available devices function in the 375- to 500-kHz range. The technique for thermal ablation utilizing RFA was first described in animal lung tumor models in 1995,19 and reported in human lungs in 2000.20
Percutaneous RFA deposits energy into the local tissue environment by converting RF waves into heat via ionic vibrations, elevating cellular temperatures, and causing necrosis. RF electrodes are available in various lengths and have an insulated shaft and an uninsulated conductive “active tip” that emits the RF current (Fig. 37.2). When possible, the electrode is positioned along the long axis of the lesion, and advanced into deepest margin of the lesion for the first treatment. The more proximal margins of the tumor can then be treated by retracting the electrode and administering an additional treatment. Larger tumors can be treated by repositioning the electrode into a new portion of the tumor 1.5 to 2 cm away from the initial longitudinal axis of the previous treatment.
The RF electrode is coupled to the RF generator and grounded by means of a grounding pad applied to the opposite side of the chest wall or the thigh. The generator produces a voltage between the two electrodes, establishing lines of electrical field that oscillate with the alternating current. This oscillating electrical field causes ionic vibrations in proportion to the field intensity. Tissue heating occurs by frictional or resistive energy loss caused by ionic motion from the current.21 Tissue heating more than temperatures of 60°C for varying amounts of time leads to coagulation necrosis and, ultimately, cell death.22
The volume of ablation is based on the energy balance between heat conduction of the local RF energy and the heat convection from the circulating blood and extracellular fluid. In the lung, RF energy is efficiently deposited because the surrounding air acts as an insulator (concentrating the energy within the lesion), and the high pulmonary vascular flow acts as a “heat sink” that dissipates heat from normal parenchyma. Although this will have a protective effect on the surrounding normal parenchyma and pulmonary vasculature, this peripheral heat sink will also limit the therapeutic ablation margins surrounding the lesion, which is important to prevent local recurrence.
Currently in the United States, there are three commercially available RFA systems. Two of the systems (Radiotherapeutics, Boston Scientific, Watertown, MA and RITA Medical Systems Inc., Mountain View, CA) utilize a deployable array RF electrode that consists of 4 to 16 small wires (tines) deployed through a 14- to 17-gauge needle. Because the tines curve backward toward the handle, the Boston Scientific device (Leveen electrode) is initially deployed at the deep aspect of the tumor. In contrast, the RITA electrode tines course forward and lateral so the probe is deployed on the near surface of the tumor. The Leveen electrode measures only impedance, and
treatment time depends on repeated rises in impedance during active heating to achieve adequate thermocoagulation. With the RITA system electrodes, temperature readings are obtained throughout the ablation cycle through multiple peripheral thermocouples. The third RF system (Radionics CC-1, Valleylab, Boulder, CO) utilizes a single or triple “cluster” (three single electrodes spaced 5 mm apart) perfused electrode; the tip is positioned at the deepest aspect of the tumor. The single or cluster RF electrode contains a thermocouple embedded in its tip, used to measure intratumoral temperature. A switching controller can be used with the Valleylab system, allowing for the placement of up to three separate single electrodes spaced 2 to 2.5 cm apart, increasing the duty cycle of the generator and allowing for the creation of a greater volume of tissue thermocoagulation compared with three separate ablations with a single electrode.
treatment time depends on repeated rises in impedance during active heating to achieve adequate thermocoagulation. With the RITA system electrodes, temperature readings are obtained throughout the ablation cycle through multiple peripheral thermocouples. The third RF system (Radionics CC-1, Valleylab, Boulder, CO) utilizes a single or triple “cluster” (three single electrodes spaced 5 mm apart) perfused electrode; the tip is positioned at the deepest aspect of the tumor. The single or cluster RF electrode contains a thermocouple embedded in its tip, used to measure intratumoral temperature. A switching controller can be used with the Valleylab system, allowing for the placement of up to three separate single electrodes spaced 2 to 2.5 cm apart, increasing the duty cycle of the generator and allowing for the creation of a greater volume of tissue thermocoagulation compared with three separate ablations with a single electrode.
The effective local control and safety profile of percutaneous RFA has been firmly established in the treatment of numerous solid malignancies, including those in liver, bone, lung, breast, kidney, and adrenals. As of this writing, there have been no head-to-head comparisons of safety and efficacy between these devices in the lung.
Microwave Ablation Like RFA, MWA utilizes electromagnetic waves to produce tissue heating effects. Unlike RFA, however, the microwave energy spectrum lies in a much higher frequency range, extending from 300 MHz to 300 GHz; microwave probes available for clinical use generally operate in the 900- to 2450-MHz range.23 Microwave tissue heating occurs as a result of the induction of kinetic energy in surrounding water molecules. Because of their electron configuration, water molecules are highly polar and function as small electrical dipoles with the negative charges preferentially localized around the oxygen nucleus. The rapidly alternating electric field of the microwave probe causes water molecules to rapidly spin in an attempt to align with electromagnetic charges of opposite polarity. These spinning water molecules interact with neighboring tissues, transferring a portion of their kinetic energy. Because temperature is merely a proxy measurement of molecular kinetic energy, this energy transfer results in local tissue hyperthermia. Currently, in the United States, there is one commercially available microwave system (VivawWave, Valleylab, Boulder, CO) that utilizes a 915-MHz generator and straight antennae with active tips of 3.7 cm (Fig. 37.2). Cooling of the antennae shaft with infused room temperature saline reduces conductive heating of the nonactive portion of the applicator, thus preventing damage to the skin and tissues proximal to the active tip.
Cryoablation Cryoablation, with the local application of liquid nitrogen directly or within sealed metal cryoprobes, has been used to treat tumors in the operating room setting for more than 3 decades. With the development of the argonbased cryoablation systems, which are now widely available, cryotherapy applicator diameters have decreased significantly, making the percutaneous utilization of this technique to other sites of disease more feasible than ever before.24
Cryoablation involves the insertion of one or more hollow needles (cryoprobes) directly into a tumor, under image guidance. The probes are filled with pressurized argon gas and cooled to temperatures as low as — 140°C via the Joule-Thomson effect (in which the expansion of compressed gas through a small gap causes a temperature drop). The extreme cold at the tip of the probe creates an expanding ice ball that freezes the surrounding tissue as it progresses. At temperatures of below — 40°C, cryogenic destruction of living tissue occurs via several mechanisms, including protein denaturation, osmotic shifts in intracellular and extracellular water, membrane destabilization, cell rupture, and tissue ischemia. Currently, there are two commercially available percutaneous argonbased cryoablation devices available (CryoHit, Galil Medical, Plymouth Meeting, PA and Cryocare, Endocare Inc., Irvine, CA; Fig. 37.2). These systems allow the placement of between 1 and 15 individual 1.5- to 2.4-mm diameter cryoprobes. Typically, a freeze-thaw-freeze cycle in a single probe position is used to achieve local tumor necrosis. Ice ball visualization under CT or MR imaging allows for direct comparison of kill zone with respect to tumor margins, and allows for accounting of a cytotoxic ice margin of 3 to 5 mm within the most peripheral aspect of the ice ball.
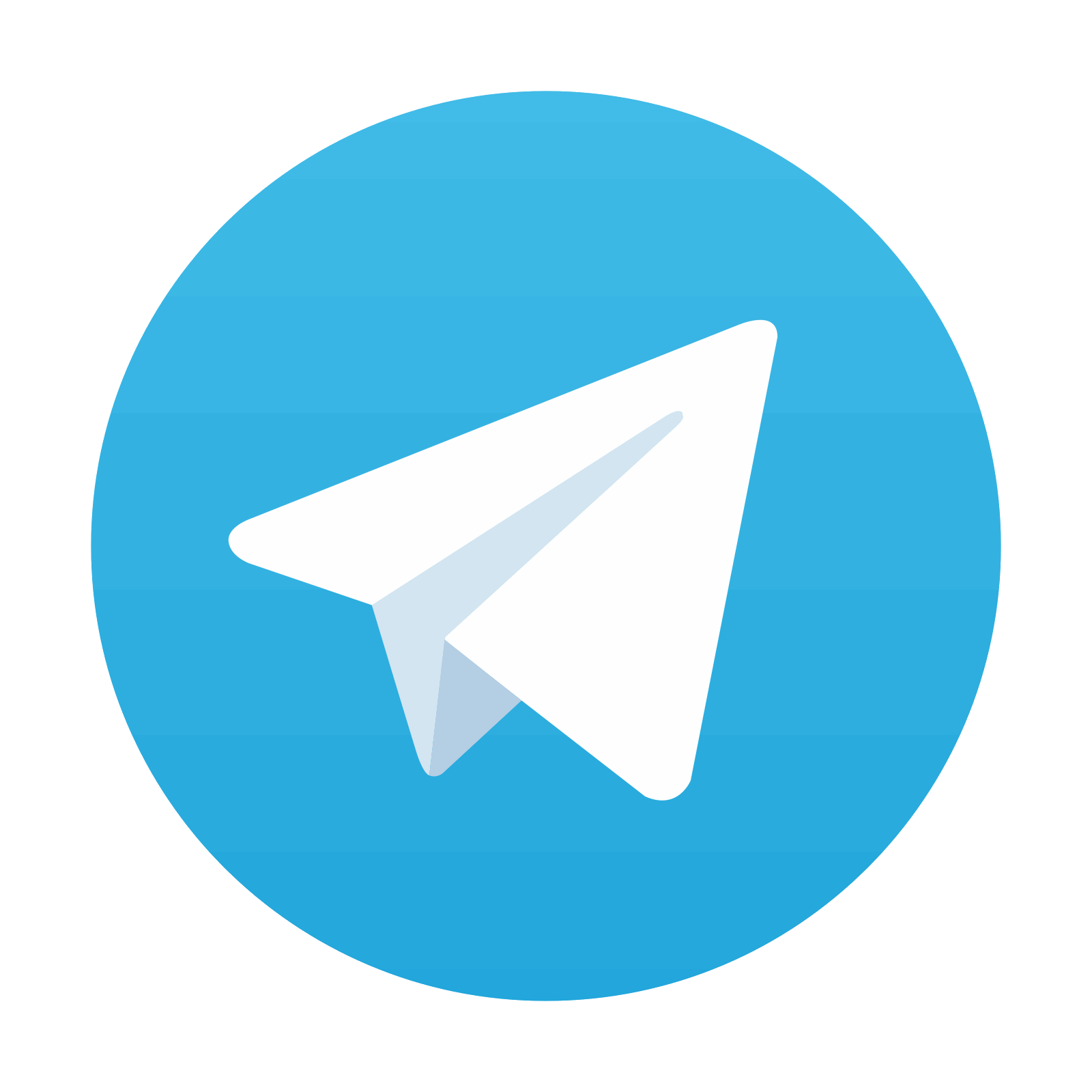
Stay updated, free articles. Join our Telegram channel

Full access? Get Clinical Tree
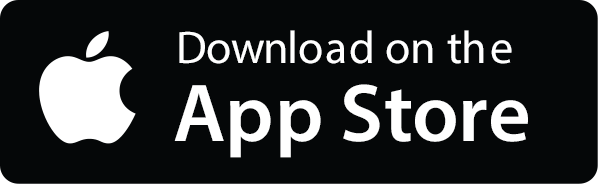
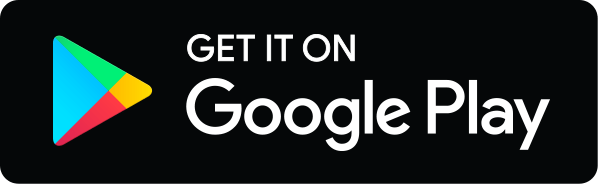