Laura Mauri, Deepak L. Bhatt
Percutaneous Coronary Intervention
The use of percutaneous coronary intervention (PCI) to treat ischemic coronary artery disease (CAD) has expanded dramatically over the past three decades. In the absence of left main or complex multivessel CAD, PCI is the preferred method of revascularization in the United States for most patients with ischemic CAD. The estimated 600,000 PCI procedures performed annually in the United States exceed the number of coronary artery bypass graft (CABG) procedures.1 Over the past several years, however, the growth of PCI has slowed because of the effectiveness of risk factor modification, prevention of restenosis with drug-eluting stents (DESs), and a better understanding of the patients who will benefit from revascularization.2,3 The number of PCIs is expected to grow modestly (1% to 5%) over the next decade as a result of the aging U.S. population and an increased frequency of obesity and diabetes. Other key enablers of the expanded use of PCI in patients with complex CAD include improvements in equipment design (e.g., catheters with lower profile and enhanced deliverability), the development of adjunctive pharmacologic strategies (e.g., adenosine diphosphate [ADP] receptor antagonists and direct thrombin inhibitors) to improve safety, and better hemodynamic support devices in “ultrahigh”-risk patients. “Hybrid” procedures for the treatment of CAD and valvular heart disease have also been performed with the collaboration of interventional cardiologists and cardiac surgeons.4,5
This chapter reviews the indications and clinical considerations for the selection of patients for PCI; discusses the current array of coronary devices, antithrombotic therapy, vascular access approaches, and vascular closure devices used for PCI; details the short- and long-term outcomes of PCI; and summarizes the requirements for operator and institutional proficiency.
Coronary balloon angioplasty, or percutaneous transluminal coronary angioplasty (PTCA), was first performed by Andreas Gruentzig in 1977 with a fixed-wire balloon catheter. The procedure was initially limited to the fewer than 10% of patients with symptomatic CAD who had a single, focal, noncalcified lesion in a proximal coronary vessel. As equipment design and operator experience evolved over the next decade, the use of PCI expanded to encompass an increasing spectrum of coronary anatomy—including multivessel CAD, total occlusions, diseased saphenous vein grafts (SVGs), and acute ST-segment elevation myocardial infarction (STEMI) (see Chapter 52), among other complexities. Two limitations prevented the widespread use of balloon angioplasty for CAD: abrupt closure of the treated vessel occurred in 5% to 8% of cases and required emergency CABG in 3% to 5%, and restenosis resulted in recurrence of symptoms in 30% of patients within the following year.
New coronary devices were developed in the late 1980s to overcome the limitations associated with balloon angioplasty. Coronary stents act as a scaffold on the inner arterial wall to prevent early and late vascular remodeling. Rotational atherectomy ablates calcific atherosclerotic plaque and was developed as stand-alone therapy for nondilatable coronary stenoses or for use in combination with coronary stents following the ablation of calcific plaque. By the early 2000s, several devices had been developed to protect the distal circulation from atherothrombotic embolization (i.e., embolic protection devices). Aspiration and thrombectomy catheters were developed to remove medium and large thrombi from within the coronary artery, thereby preventing distal embolization. The term percutaneous coronary intervention now encompasses the broad array of balloons, stents, and adjunctive devices required to perform safe and effective percutaneous revascularization in complex coronary artery lesions.
Indications for Percutaneous Coronary Intervention
Clinical Presentations
The major value of percutaneous or surgical coronary revascularization is relief of the symptoms and signs of ischemic CAD (see Chapters 52 and 54 and PCI Guidelines at the end of this chapter). PCI reduces the risk for mortality and subsequent myocardial infarction (MI) when compared with medical therapy in patients with acute coronary syndromes. Optimal medical therapy appears to be as effective as PCI in reducing death and MI in patients with stable angina, although relief of symptoms2 and improvement of ischemia6 are better with PCI. Greater than 5% improvement in the ischemic burden is achieved more often with PCI, and the magnitude of the residual ischemia correlates with less frequent death and MI.6 Further studies comparing the use of coronary arteriography and PCI in patients with moderate degrees of myocardial ischemia are planned (e.g., ISCHEMIA [International Study of Comparative Health Effectiveness with Medical and Invasive Approaches]),7 and recent randomized trials requiring physiologic evidence of ischemia (as measured by fractional flow reserve [FFR]) have identified a benefit of PCI over medical therapy in preventing urgent revascularization.8 Irrespective of the indication for revascularization, PCI should be coupled with optimal medical therapy after the procedure, such as control of hypertension and diabetes, exercise, and smoking cessation (see Chapter 42 and PCI Guidelines in this chapter). Lipid management, particularly statin use, is also an important component of optimal medical therapy.
When compared with PCI alone, CABG is associated with a late mortality benefit in certain high-risk medical and anatomic subsets, such as patients with left main disease, three-vessel CAD, and extensive markers of higher anatomic risk for PCI (as determined by a SYNTAX [Synergy Between PCI with TAXUS and Cardiac Surgery] score, for example)9 or patients with diabetes and significant multivessel disease.10 These benefits are manifested beyond 1 year after treatment and for up to 5 years of follow-up, but the early periprocedural risks, particularly for stroke, are higher with CABG, and it has a longer in-hospital recovery period. The risks and benefits associated with coronary revascularization therefore need careful review with the patient and family, and the relative options of PCI, CABG, or optimal medical therapy should be discussed before performing these procedures. Patients with multivessel disease benefit from joint consultation with a cardiac surgeon, an interventional cardiologist, and the referring cardiologist, and consideration of patient preferences in weighing diverse factors is valuable. A task force of the American College of Cardiology (ACC) and American Heart Association (AHA) has published guidelines for the performance of PCI and CABG procedures,11–13 and a multispecialty writing committee has developed appropriate use criteria for revascularization in several clinical and lesion-specific subsets (see PCI Guidelines).14,15
Asymptomatic or Minimally Symptomatic Patients
Asymptomatic patients or those who have only mild symptoms are generally best treated with medical therapy unless one or more high-grade lesions subtend a moderate to large area of viable myocardium, the patient prefers to maintain a very active lifestyle or has a high-risk occupation, and the procedure can be performed with a high chance of success and low likelihood of complications (see PCI Guidelines at the end of this chapter).15 Patients who are minimally symptomatic or asymptomatic should not undergo coronary revascularization if only a small area of myocardium is at risk, if no objective evidence of ischemia can be detected, or if the likelihood of success is low or the chance of complications is high.14
Patients with Moderate to Severe Angina (See Chapter 54)
Patients with Canadian Cardiovascular Society (CCS) class III angina, particularly those who are refractory to medical therapy, can benefit from coronary revascularization, provided that the lesion subtends a moderate to large area of viable myocardium as determined by noninvasive testing.12 Patients with recurrent symptoms while receiving medical therapy are candidates for revascularization even if they have a higher risk for an adverse outcome with revascularization. Patients with class III symptoms should not undergo revascularization without noninvasive evidence of myocardial ischemia or a trial of medical therapy, particularly if only a small region of myocardium is at risk, the likelihood of success is low, or the chance of complications is high.14
Patients with Unstable Angina, Non–ST-Segment Elevation Myocardial Infarction, and ST-Segment Elevation Myocardial Infarction (see Chapters 52 and 53)
Cardiac catheterization and coronary revascularization in moderate- to high-risk patients with unstable angina or non–ST-segment elevation MI (NSTEMI) may improve mortality and reduce the rate of reinfarction.16 In a meta-analysis of seven trials with 8375 patients monitored for up to 2 years, the all-cause mortality rate was 4.9% in the early invasive group as compared with 6.5% in the conservative group (risk ratio [RR], 0.75; P = 0.001). The 2-year incidence of nonfatal MI was 7.6% in the invasive group and 9.1% in the conservative group (RR, 0.83; P = 0.012). At a mean of 13 months of follow-up, rehospitalization for unstable angina was reduced as well (RR, 0.69; P < 0.0001). Current guidelines suggest that an early invasive strategy should be pursued in patients with recurrent ischemia despite therapy, elevated troponin levels, new ST-segment depression, new or worsening symptoms of heart failure, depressed left ventricular (LV) function, hemodynamic instability, sustained ventricular tachycardia, or a recent PCI or CABG procedure (see PCI Guidelines).16
Several clinical recommendations pertaining to patients with STEMI, including primary PCI, rescue PCI, facilitated PCI, and PCI following successful thrombolysis, have been published (see PCI Guidelines).17 Timely PCI in patients with STEMI improves survival over that achieved with medical therapy, provided that it is performed by a physician who routinely performs PCI, and that the hospital has sufficient PCI volume to support its proficiency. Patients with cardiogenic shock or severe heart failure also benefit from primary PCI, irrespective of their age at initial evaluation.
Left Main and Three-Vessel Coronary Artery Disease
The SYNTAX trial randomly assigned 1800 patients with multivessel or left main disease (1709 had multivessel disease) to PCI with a DES versus CABG.3 Complete revascularization was the goal for both study groups, and the average number of treated vessels and stents in patients undergoing PCI for left main or multivessel disease was 3.6 lesions and 4.6 stents. One-year outcomes were not different between the PCI and CABG groups in terms of all-cause mortality or MI. However, rates of major adverse cardiovascular or cerebrovascular events were significantly higher with PCI, mainly attributable to the significantly higher rates of target lesion revascularization in the PCI group. Three-year outcomes were similar to these findings, with no significant difference in all-cause mortality, but a persistently elevated rate of target lesion revascularization was associated with PCI.9 These findings were dependent on the SYNTAX score—an angiographic grading system to quantify the complexity of PCI (see Chapter 20). With low scores (≤22), there was no significant difference in the primary outcome between patients treated with PCI and those who underwent CABG, but with high scores (>33), mortality and major adverse cardiovascular or cerebrovascular event rates were lower with CABG procedures.
Patients with Diabetes Mellitus
The largest trial to date devoted to studying outcomes after revascularization in patients with diabetes mellitus and multivessel disease is FREEDOM (Future Revascularization Evaluation in Patients with Diabetes Mellitus: Optimal Management of Multivessel Disease), a randomized controlled trial that compared the outcomes of PCI and CABG in patients with diabetes and multivessel disease.10 In this trial, diabetic patients requiring revascularization with angiographically proven multivessel disease and lesions amenable to either PCI or CABG were randomly assigned to complete coronary revascularization with one of these techniques. The primary outcome (all-cause mortality, nonfatal MI, or nonfatal stroke) at 5 years was better in patients treated with PCI than in those who underwent CABG (26.6% versus 18.7%, P = 0.005). Considering individual components of the primary outcome, there was a significantly increased long-term risk for all-cause mortality and nonfatal MI with PCI as opposed to CABG. CABG, however, was associated with an increased risk for nonfatal stroke, and the severity of strokes in the CABG group was twice as likely to severely disable a patient as were strokes occurring in the PCI group.
The results of the FREEDOM trial have largely validated smaller studies, subgroup analyses, and meta-analyses attempting to compare methods of revascularization in diabetic patients with multivessel disease. The CARDia (Coronary Artery Revascularization in Diabetes) randomized trial compared PCI with CABG; although smaller in size, it was dedicated to patients with diabetes, and a significant fraction suffered from multivessel disease.18 The trial lacked sufficient power to compare mortality. Like the FREEDOM trial, however, it revealed an increased risk for stroke in the CABG group. Similarly, even though most patients were treated with DESs in this trial, the need for repeated revascularization procedures was significantly increased with PCI.
Patients Without Options for Revascularization
Patients who suffer from substantial angina but are poor candidates for conventional revascularization have limited therapeutic options. These patients generally either have occlusion of a single proximal vessel that subtends a large amount of myocardium or have undergone one or more previous CABG operations with stenoses or occlusions of the SVGs, which are poorly suited for conventional repeated revascularization. “Limited-option” patients account for approximately 4% to 12% of those undergoing coronary angiography; a larger group (20% to 30%) of patients has incomplete revascularization because the coronary anatomy is unsuitable for surgical or percutaneous techniques. Better techniques and equipment for crossing chronic total occlusions (see later) have helped some of these patients. Antianginal medications such as ranolazine (see Chapter 54) may also be particularly useful in this subset.
Patient-Specific Considerations for Percutaneous Coronary Intervention
Assessment of the potential risks and benefits of PCI must address five fundamental patient-specific risk factors: extent of jeopardized myocardium, baseline lesion morphology, underlying cardiac function (including LV function, rhythm stability, and coexisting valvular heart disease), presence of renal dysfunction, and preexisting medical comorbid conditions that may place the patient at higher risk for PCI. Each of these factors contributes independently to the risks and benefits attributable to PCI. Proper planning for a PCI procedure requires careful attention to each of these factors.
Extent of Jeopardized Myocardium
The proportion of viable myocardium subtended by the treated coronary artery is the principal consideration in assessing the acute risk associated with the PCI procedure. PCI interrupts coronary blood flow for a period of seconds to minutes, and the ability of patients to hemodynamically tolerate a sustained coronary occlusion depends on both the extent of “downstream” viable myocardium and the presence and grade of collaterals to the ischemic region. Although the risk for abrupt closure has been reduced substantially with the availability of coronary stents, when other procedural complications develop—such as a large side branch occlusion, distal embolization, perforation, or no-reflow—rapid clinical deterioration may occur that is proportionate to the extent of jeopardized myocardium. In the unlikely event that out-of-hospital stent thrombosis develops, the clinical sequelae of the episode are related to the extent of myocardium subtended by the occluded stent. Predictors of cardiovascular collapse with a failed PCI include the magnitude of myocardium at risk, the severity of the baseline stenosis, multivessel CAD, and the presence of diffuse disease.
Complete Versus Ischemia-Targeted Percutaneous Coronary Intervention for Multivessel Disease
The treatment approaches for PCI and CABG compared in randomized trials have essentially all focused on the strategy of complete revascularization. Data supporting complete revascularization of all angiographic lesions during treatment of multivessel disease with PCI or CABG have been observational (nonrandomized) and thus limited by selection bias in that patients in whom full revascularization is feasible are also those who are at lower risk for procedural and future adverse events. The concept of targeted treatment of vessels with only physiologically significant—not just angiographically significant—stenosis may be one that allows improved outcomes in patients with multivessel disease being treated with either PCI or CABG.
The FFR technique (see Chapters 49 and 54) involves placement of a pressure wire across a potentially significant lesion, and under conditions of maximal coronary blood flow, the ratio of pressure distal to versus proximal to a lesion or series of sequential lesions is measured within a given artery (Fig. 55-1). In contrast to traditional angiography, which can provide only an anatomic evaluation, FFR provides a functional assessment of the presence of a reduction in flow that correlates well with ischemia as detected by nuclear scintigraphy. The FAME (Fractional Flow Reserve versus Angiography for Multivessel Evaluation) trial compared angiography with FFR guidance for selection of lesions during DES PCI in more than 1000 patients.19 Only lesions with an FFR of 0.8 or less were considered to warrant PCI in the FFR arm. FFR guidance resulted in fewer overall stented lesions, and a 2-year analysis revealed significantly reduced mortality or MI with the use of FFR versus pure angiographic guidance, thus corroborating not only the simple procedural benefit of FFR but also the morbidity and mortality advantages of stenting across physiologically relevant lesions.
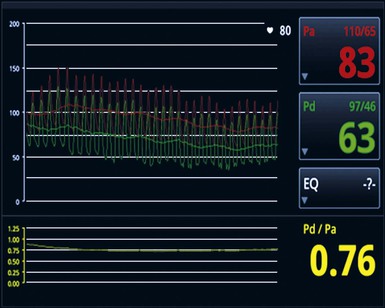
In patients with STEMI, revascularization of only the culprit infarct-related artery is generally recommended14 unless there is ongoing cardiogenic shock because of jeopardized myocardium in other regions. Appropriately powered trials to determine whether nonculprit severe lesions should be treated even in the absence of shock are planned.
Baseline Lesion Morphology
Several angiographic findings increase the technical complexity of PCI and elevate the risk for acute and long-term complications. The initial ACC/AHA lesion classification system has been refined with use of the Society for Cardiovascular Angiography and Interventions (SCAI) risk system, which further characterizes risk by the presence or absence of total occlusion. Although coronary stents have reduced the need for emergency CABG from 3% to 8% with balloon angioplasty to less than 1% with the availability of coronary stents, they have not eliminated the risk for periprocedural MI, stent thrombosis, or distal embolization and “no reflow.” Vessel patency and lesion complexity remain important predictors of outcome in patients undergoing coronary stent placement. Reviews of registry data have confirmed the impact of high-risk lesion features on procedural success rates and the risk for short- and long-term complications. Most recently, the SYNTAX angiographic scoring system—when combined with clinical factors—has become a method of deciding between complex PCI and CABG.20,21 An online calculator is available (www.syntaxscore.com).
Chronic Total Occlusions
Chronic coronary occlusions occur in many patients with severe (>70% stenosis) CAD and are the most important factor leading to referral for CABG procedures rather than PCI. The inability of guidewires to recanalize total coronary occlusions is related to several factors, including the duration of the occlusion, the presence of bridging collaterals, occlusion length greater than 15 mm, and the absence of a “beak” to assist in advancement of the guidewire. Although approaches such as retrograde crossing via collaterals and newer guidance technologies have been used to recanalize refractory occlusions, better guidewires and wire techniques have accounted for much of the improvement in successfully crossing occlusions over recent years.22 Once the chronic total occlusion has been crossed, DESs may be used to reduce late clinical recurrence.
Saphenous Vein Grafts
SVG interventions account for approximately 8% of PCI procedures and pose an increased risk for postprocedural MI as a result of the atheroembolization that occurs during PCI. When no-reflow occurs, administration of arterial vasodilators (e.g., nitroprusside, verapamil, or adenosine) into the SVG may improve flow into the distal native circulation, but the risk for death or MI is still substantially increased. More extensive SVG degeneration and bulkier lesions are associated with higher complication rates than are SVGs that have less extensive disease.23 In the setting of “high-risk” SVG anatomy, alternative approaches using the native coronary artery should be pursued whenever possible. Lower rates of restenosis in SVG lesions occur after coronary stent placement than after balloon angioplasty. Although DESs provide lower restenosis rates in SVGs that are 4.0 mm or less, they are currently not available for SVGs larger than 4.5 mm in diameter, and bare metal stents (BMSs) are reasonable in this setting. Embolic protection devices are strongly recommended in patients treated for SVG stenoses to lessen the risk for distal embolization of atherothrombotic debris.
Bifurcation Lesions
Optimal management of lesions involving both branches of a coronary bifurcation remains controversial. “Snowplowing” of plaque into the adjacent parent vessel or side branch is a major limitation of conventional balloon angioplasty. Atheroablative procedures such as rotational atherectomy have not really reduced this risk. Risk stratification for bifurcation PCI includes assessment of the extent of atherosclerotic disease in both vessels, estimation of relative vessel size and distribution in the parent vessel and side branch, and determination of the orientation of the vessels to one another. Side branch compromise may also occur in up to 30% of bifurcation lesions without apparent branch vessel disease.
Stent placement in one vessel rather than in both the parent vessel and side branch is generally preferred. In a meta-analysis of six randomized trials that included 1642 patients with coronary bifurcation lesions who were randomly selected to undergo PCI involving either double or single stenting, the risk for MI increased with double stenting (RR, 1.78; P = 0.001).24
When extensive disease occurs in both vessels, various strategies have been used, including simultaneous “kissing” stents (Fig. 55-2) and “crush,” culotte, T stenting, and TAP (“T and small protrusion”) techniques. Irrespective of the bifurcation stenting strategy used, a final kissing balloon inflation in the parent vessel and side branch should generally be performed. DESs appear to have lower restenosis rates than BMSs do, but when recurrence develops in patients treated with a DES, it generally occurs at the origin of the side branch. New dedicated bifurcation stents and side branch access main vessel stents are in development. Determination of the FFR of an angiographically narrowed side branch can be useful if it demonstrates no significant impairment of flow and therefore no need to place a stent.
Lesion Calcification
The presence of extensive coronary calcification poses unique challenges for PCI because calcium in the vessel wall leads to irregular and inflexible lumens, thus making delivery of guidewires, balloons, and stents much more challenging. Extensive coronary calcification also renders the vessel wall rigid, which necessitates higher balloon inflation pressure to achieve complete stent expansion and, on occasion, leads to “undilatable” lesions that resist any balloon expansion pressure that can be achieved. Rotational atherectomy effectively ablates the vessel wall calcification and facilitates stent delivery and complete stent expansion (Fig. 55-3).
Thrombus
Conventional angiography has poor sensitivity for the detection of coronary thrombus, but the presence of a large, angiographically apparent coronary thrombus heightens the risk for procedural complications. Large coronary thrombi may fragment and embolize during PCI or may extrude through gaps between stent struts placed in the vessel, thereby risking lumen compromise or thrombus propagation and acute thrombosis of the treated vessel. In addition, large coronary thrombi can embolize to other coronary branches or vessels or dislodge and compromise the cerebral or other vascular beds. In the setting of STEMI, manual catheter aspiration of thrombus appears to reduce the risk for future ischemic events, including stent thrombosis and, potentially, mortality.
Left Main Coronary Artery Disease
The presence of left main CAD has been an accepted indication for CABG because of the potential for hemodynamic collapse in the setting of acute complications, stent thrombosis, or restenosis involving the body of the left main coronary artery or its extension into the left anterior descending or left circumflex coronary artery. Registry and randomized studies have suggested that rates of death or MI are similar in patients undergoing CABG or PCI,3,25,26 although the need for repeated revascularization is higher in patients treated with PCI with additional vessel disease.3,27–34 The use of PCI for left main CAD has been elevated to a class IIb indication (see PCI Guidelines at the end of this chapter) pending additional ongoing randomized study, such as the EXCEL (Evaluation of Xience Prime versus Coronary Artery Bypass Surgery for Effectiveness of Left Main Revascularization) trial, in which patients with left main CAD lesions were randomly assigned to everolimus-eluting stents or CABG.
Underlying Cardiac Function
LV function is an important predictor of outcome during PCI. For each 10% decrement in resting LV ejection fraction, the risk for in-hospital mortality following PCI increases approximately twofold. Associated valvular disease or ventricular arrhythmia further increases the risk associated with PCI in the setting of LV dysfunction. Intra-aortic balloon pump support may be useful when LV function is severely compromised (i.e., ejection fraction <35%) or when the PCI target lesion supplies a substantial portion of viable myocardium. Routine use of intra-aortic balloon pumps has limited benefit in patients with STEMI,35 although they are recommended for patients in cardiogenic shock. Other percutaneous cardiopulmonary support devices that do not effectively reduce LV pressure have been replaced by percutaneous LV assist devices that are positioned in the left atrium (e.g., TandemHeart, CardiacAssist, Inc., Pittsburgh, Pa)36,37 or directly in the left ventricle (Impella, Abiomed, Inc., Danvers, Mass)38–40 (Fig. 55-4). These devices may permit very high-risk PCI with less chance of hemodynamic collapse during the procedure, although current data do not show them to be superior to intra-aortic balloon pumps.41 Peripheral extracorporeal membrane oxygenation (ECMO) support through large-bore arterial and venous access may be useful in cases of cardiovascular collapse.
Renal Insufficiency
The morbidity and mortality associated with PCI are directly related to the extent of baseline renal disease (see also Chapter 88). Patients with evidence of mild renal dysfunction have a 20% higher risk for death at 1 year following PCI than do those with preserved renal function. Renal dysfunction following the administration of contrast material during angiography may be related to contrast-induced nephropathy (see Chapter 19), to cholesterol embolization syndrome (see Chapters 58 and 88), or to both. The risk for nephropathy is dependent on the dose of the contrast agents used, hydration status at the time of the procedure, preexisting renal function of the patient, age, hemodynamic stability, anemia, and diabetes. The risk for cholesterol embolization syndrome is related to manipulation of the catheter in an ascending or descending atherosclerotic aorta from which cholesterol crystals are released. Although the risk associated with hemodialysis is less than 3% in cases of uncomplicated contrast-induced nephropathy, in-hospital mortality in the setting of hemodialysis exceeds 30%. Mild renal dysfunction following PCI is associated with an up to fourfold increased risk for death at 1 year following PCI when compared with patients with preserved renal function, although this association is probably not causal.
Associated Medical Comorbid Conditions
A bleeding diathesis or need for chronic warfarin therapy may preclude patients from tolerating long-term combination aspirin and clopidogrel therapy after placement of a DES, thereby placing them at higher risk for stent thrombosis. The need for discontinuation of dual antiplatelet therapy before impending non–cardiac-related surgery soon after stent implantation may also predispose to stent thrombosis. In each of these circumstances, BMS placement may be the preferred approach, particularly if the surgery can be deferred for approximately 6 weeks after placement of the stent (Fig. 55-5).42,43
Vascular Access
The most frequently used vascular access sites for PCI include the common femoral artery, the brachial artery, and increasingly, the radial artery (see Chapters 19 and 20). The femoral approach (either right or left sided) is the most commonly used vascular access site in the United States and provides the advantages of large vessel size (typically 6 to 8 mm in diameter) and the ability to accommodate larger (>6 French [F]) sheath sizes, including intra-aortic balloon pumps. In addition, because of the typically straight path from the femoral artery to the ascending aorta, the femoral approach provides excellent guide catheter support and manipulability and access to the venous system through the adjacent femoral vein. The presence of severe peripheral arterial disease or peripheral vascular bypass grafts and the requirement for immobilization following the procedure limit use of the femoral approach in some patients.
The brachial arterial approach was historically used as the principal alternative to femoral access, but because the brachial artery provides the only circulation to the forearm and hand (i.e., it is a functional end-artery), any compromise of the brachial artery can lead to severe ischemic complications in the hand.
The radial artery approach (see Chapters 19 and 20) has gained in popularity as an alternative to femoral access in patients with significant peripheral vascular disease, particularly in obese patients, in whom direct compression of the radial artery reduces bleeding complications.44,45 The radial approach provides direct access to the ascending aorta and has the unique advantage of allowing immediate mobilization following PCI. An Allen test is useful to assess flow to the hand before radial artery cannulation. Tortuosity of the brachiocephalic trunk may limit use of the approach in 2% to 3% of patients. The small size of the radial artery limits the size of guiding catheters that can be used during PCI (typically 5F or 6F for women and 7F for men). Transradial access is associated with a generally lower rate (2%) of vascular complications.46 A meta-analysis suggested that radial access reduced major bleeding in comparison to femoral access.47 The RIVAL (Radial vs Femoral Access for Coronary Intervention) trial randomly assigned patients to either femoral or radial access; no significant difference was found in the primary endpoint of major ischemic events or bleeding48 (Table 55-1), but the rate of vascular complications was significantly reduced with the radial approach.
TABLE 55-1
Outcomes in Radial Versus Femoral Access in the RIVAL Trial
FEMORAL (n = 3514) | RADIAL (n = 3507) | P VALUE | |
Composite of death, MI, stroke, or non-CABG–related major bleeding at 30 days* | 4.0% | 3.7% | 0.50 |
Death at 30 days | 1.5% | 1.3% | 0.47 |
MI at 30 days | 1.9% | 1.7% | 0.65 |
Stroke at 30 days | 0.4% | 0.6% | 0.30 |
PCI success | 95.2% | 95.4% | 0.83 |
Access site crossover | 2.0% | 7.6% | <0.0001 |
Major vascular complications | 3.7% | 1.4% | <0.0001 |
Access site major bleeding | 0.3% | 0.2% | Not provided |
Symptomatic radial occlusion | NA | 0.2% | NA |
Procedure time (min) | 35 | 34 | 0.62 |
Fluoroscopy time (min) | 8.0 | 9.3 | <0.0001 |
Contrast volume (mL) | 180 | 181 | 0.87 |
Patient prefers radial access for next procedure | 50.7% | 90.2% | <0.0001 |
* Primary endpoint.
NA = not applicable.
Modified from Jolly SS, Yusuf S, Cairns J, et al: Radial versus femoral access for coronary angiography and intervention in patients with acute coronary syndromes (RIVAL): A randomised, parallel group, multicentre trial. Lancet 377:1409, 2011.
Vascular Access Complications
Vascular access site complications occur after 3% to 7% of femoral PCIs and lead to significantly increased length of hospital stay, total cost, and morbidity and mortality. Complications range from relatively minor access site hematomas, to life-threatening retroperitoneal bleeding requiring emergency blood transfusion, to damage to the vasculature necessitating prompt surgical intervention. Factors predisposing patients to increased risk for serious vascular complications following PCI include older age, female sex, larger vascular sheath size, low body mass index, renal insufficiency, and degree of anticoagulation during the procedure. The location of the entry point for transfemoral access predicts the risk and type of vascular complication (see Chapter 19). If the access site is above the level of the inguinal ligament, the risk for retroperitoneal hemorrhage increases substantially. If the access site is distal to the femoral bifurcation, pseudoaneurysms (0.4%) and arteriovenous fistulas (0.2%) may occur. Major vascular complications of the femoral approach include limb-threatening ischemia (0.1%) and retroperitoneal hemorrhage (0.4%), which are associated with a 2- to 10-fold increased risk for death in the first 30 days following the PCI procedure.
Vascular Closure Devices
Vascular access closure devices were introduced in the mid-1990s as a new way of managing access sites following femoral access procedures. Vascular closure devices reduce the time to ambulation, increase patient comfort following PCI, and facilitate efficient case flow in the catheterization laboratory.49
Currently approved vascular closure devices fall into three categories: (1) sealant devices include collagen-based and thrombin-based systems that leave no mechanical anchor inside or outside the vessel; (2) mechanical closure devices include suture-mediated and nitinol clip–based systems and provide immediate secure closure to the vessel; and (3) hybrid closure devices, such as the dissolvable AngioSeal device (St. Jude Medical, Minneapolis), use a combination of collagen sealant and internal mechanical closure to induce rapid hemostasis.50 Although each device has proved to be relatively safe and effective, a lack of comparative data prohibits evaluation of the relative risks and benefits associated with each device. Meta-analyses have concluded that vascular closure devices do not lower the risk for vascular complications when compared with manual hemostasis, but infections may occur more often with suture-based closure devices, and occlusions are found more often with hybrid devices. Registry analyses have suggested that closure devices reduce bleeding complications in selected patients,51 but randomized clinical trials are necessary to validate this finding.
Coronary Devices
Over the past three decades, steady improvements in the equipment used for coronary revascularization (e.g., reductions in device profile and improvements in catheter flexibility) have been supplemented by the introduction of periodic “transformational technology,” such as coronary stents and, more recently, DESs, which have extended the scope and breadth of clinical practice. The type of lesions amenable to PCI has become progressively more complex over this period, and the outcomes associated with the use of these devices have progressively improved. A brief overview of currently available coronary devices follows.
Balloon Angioplasty
Balloon angioplasty expands the coronary lumen by stretching and tearing the atherosclerotic plaque and vessel wall and, to a lesser extent, by redistributing atherosclerotic plaque along its longitudinal axis. Elastic recoil of the stretched vessel wall generally leaves a 30% to 35% residual diameter stenosis, and the vessel expansion can result in propagation of coronary dissections and lead to abrupt vessel closure in 5% to 8% of patients. Although stand-alone balloon angioplasty is rarely used other than for very small (<2.25 mm) vessels, balloon angioplasty remains integral to PCI for predilating lesions before stent placement, deploying coronary stents, and further expanding stents after deployment.
Most enhancements in balloon technology are related to the development of low-profile (deflated diameter = 0.7 mm) balloons that can be tracked more readily through tortuous anatomy and noncompliant balloons that can be inflated to pressures in excess of 20 atm without overexpansion or rupture. A modification of balloon angioplasty includes a focused-force dilation in which a scoring blade or guidewire external to the balloon concentrates the dilating force and resists balloon slippage during inflation. The Cutting Balloon (Boston Scientific Corp., Natick, Mass) and the AngioScore catheter (AngioScore, Inc., Fremont, Calif) are focused-force balloon angioplasty systems that are currently used in a small minority (<5%) of PCIs. They are sometimes useful in restenotic stent lesions to prevent slippage of the balloon during inflation.
Coronary Atherectomy
Atherectomy refers to removal (rather than simple displacement) of the obstructing atherosclerotic plaque. By removing plaque or improving lesion wall compliance in calcified or fibrotic lesions, atherectomy can provide a larger final minimal lumen diameter than can be achieved by balloon angioplasty alone. Atherectomy was performed in 30% of interventional procedures between 1992 and 1994, but its use fell dramatically with the availability of coronary stents. Fewer than 5% of current procedures involve the use of atherectomy, most often rotational atherectomy in combination with coronary stents.
The Rotablator Rotational Atherectomy System (Boston Scientific) is the most commonly used atherectomy device and removes atheromatous plaque by abrasion of the inelastic calcified plaque with microscopic (20 to 50 µm) diamond chips embedded on the surface of a rapidly rotating (160,000 rpm) olive-shaped atherectomy burr. Such abrasion generates 2- to 5-µm microparticles that pass through the coronary microcirculation for removal by the reticuloendothelial system. The burrs travel over a specialized 0.009-inch guidewire and are available in diameters ranging from 1.25 to 2.50 mm. In the setting of severe calcification, smaller (1.25 mm) burrs can be used initially, followed by larger burrs in 0.25- to 0.50-mm increments up to 70% of the reference vessel diameter. Aggressive rotational atherectomy techniques do not provide a restenosis advantage over more conservative methods and tend to increase acute procedural complications such as distal embolization or coronary perforation. Rotational atherectomy does not appear to reduce restenosis in noncalcified vessels any more than balloon angioplasty does. Rotational atherectomy is currently reserved for ostial and heavily calcified lesions that cannot be dilated with balloon angioplasty or those that prevent the delivery of coronary stents. Rotational atherectomy is generally limited to abrasion of superficial calcification with a single 1.5- or 1.75-mm burr to improve lesion compliance (plaque modification) before the lesion is treated definitively by balloon dilation and stent placement. Rotational atherectomy is presently used in fewer than 5% of PCI procedures (Fig. 55-6).
Thrombectomy and Aspiration Devices
The AngioJet rheolytic thrombectomy catheter (Possis Medical, Inc., Minneapolis, Minn) was introduced as a dedicated device for thrombus removal through dissolution and aspiration of the thrombus. High-speed saline jets within the tip of the catheter create intense local suction via the Venturi effect; surrounding blood, thrombus, and saline are pulled into the lumen of the catheter opening and the debris is propelled proximally through the catheter lumen. Rheolytic thrombectomy was superior to a prolonged intraluminal urokinase infusion in patients with a large thrombus, but its routine use in patients with STEMI was not associated with improvement in infarct size on single photon emission computed tomography (SPECT) imaging and may have caused more complications. Rheolytic thrombectomy may still be useful in clinical practice when a large angiographic thrombus is located in a native vessel or SVG.
Newer, lower-profile aspiration catheters that use 6F guiding catheters have been developed as alternatives to rheolytic thrombectomy in patients with thrombus-containing lesions. Although simpler to use, these techniques may be slightly less effective (particularly with partially organized thrombus) than rheolytic thrombectomy, but the risk for distal particulate embolization and device-related trauma in smaller vessels may be lower with these aspiration catheters. In a multicenter study of 1071 patients with STEMI who were randomly assigned to a thrombus aspiration group or a conventional PCI group, a myocardial blush grade of 0 or 1 occurred in 17.1% of the patients in the thrombus aspiration group and in 26.3% of those in the conventional PCI group (P < 0.001).52 At 30 days the rate of death in patients with a myocardial blush grade of 0 or 1, 2, and 3 was 5.2%, 2.9%, and 1.0%, respectively (P = 0.003), and the rate of adverse events was 14.1%, 8.8%, and 4.2%, respectively (P < 0.001).53 Meta-analysis of the data suggests that simple manual thrombus aspiration before PCI reduces mortality in patients undergoing primary PCI (Fig. 55-7).53
Embolic Protection Devices
The advent of embolic protection systems has reduced the risk for postprocedural adverse events following SVG PCI. Although embolization of atherosclerotic debris was not considered a major complication during the early years of native coronary balloon angioplasty, it is now recognized as a potential cause of distal myocardial necrosis after PCI, particularly in friable SVG lesions. Distal embolization causes postprocedural elevation of cardiac enzymes in almost 20% of cases after SVG PCI, and this enzyme elevation is associated with substantial morbidity and mortality. Numerous additional occlusive and filter-based distal protection systems, as well as proximal occlusion devices, have undergone evaluation for use in SVG interventions,23,54 but currently the filter devices are most commonly available. Despite their potential benefit in preventing thromboembolization in patients with STEMI, none of the embolic protection devices have reduced MI size with primary intervention, for which thrombus aspiration remains the mainstay of therapy.
Distal Embolic Filters
Distal filters are advanced across the target lesion in their smaller collapsed state, and withdrawal of the retaining sheath allows the filters to open and expand against the vessel wall. The filters then remain in place to catch any liberated embolic material larger than the pore size (usually 120 to 150 µm) of the filter during intervention. At the end of the intervention the filters are collapsed by using a sheath, and the captured embolic material is removed from the body. This type of device has the advantage of maintaining anterograde flow during the procedure and allowing intermittent injection of contrast material to visualize the underlying anatomy, but it has the potential disadvantage of allowing debris with a diameter smaller than the pore size of the filter to pass (Fig. 55-8).
Coronary Stents
Coronary stents have emerged as the predominant form of PCI and are currently used in more than 90% of PCI procedures worldwide. Coronary stents act as scaffolds for arterial dissection flaps, thereby lowering the incidence of vessel closure and need for emergency CABG; they also lessen the frequency of restenosis because of their effect in preventing arterial recoil, which is the primary mechanism of restenosis with balloon angioplasty. Despite the late clinical improvement in comparison to balloon angioplasty, restenosis after coronary stent placement occurs in some patients as a result of excessive intimal hyperplasia within the stent. A number of second-generation balloon-expandable stents were introduced between 1997 and 2003; they vary in metallic composition (i.e., cobalt chromium or layered metals versus solid 316 L stainless steel), strut design, stent length, delivery and deployment system, and arterial surface coverage, among other factors. These modifications enhanced flexibility and ease of delivery of the stent while also improving vessel scaffolding and side branch access.
The early use of coronary stents was limited by high (3% to 5%) subacute thrombosis rates despite aggressive antithrombotic therapy with aspirin, dipyridamole, periprocedural low-molecular-weight dextran, and an uninterrupted transition from intravenous heparin to oral warfarin. Subacute thrombosis produced profound clinical consequences that resulted in an untoward outcome (e.g., death, MI, or emergency revascularization) in virtually every such patient. Lower frequencies of subacute stent thrombosis (roughly 0.5% to 1.0%) have resulted from the use of high-pressure stent deployment and with a drug regimen that includes aspirin and an ADP receptor antagonist (clopidogrel, prasugrel, or ticagrelor) (see Chapter 82) started just before or after stent placement.
Even though coronary BMSs reduce the incidence of angiographic and clinical restenosis when compared with balloon angioplasty, angiographic restenosis (follow-up diameter stenosis >50%) still occurs in 20% to 30% of patients, and clinical restenosis (recurrent angina caused by restenosis in the treated segment) develops in 10% to 15% of patients in the first year after treatment. Restenosis with BMSs occurs more often in patients with small vessels, long lesions, and diabetes mellitus, among other factors. Adjunctive pharmacologic therapy has not prevented restenosis after stent placement.
Several mechanical treatments of in-stent restenosis have been attempted, including balloon redilation, removal of in-stent hyperplasia by means of atherectomy, and repeated BMS placement. Brachytherapy using beta or gamma sources modestly improved this outcome for in-stent restenosis, but brachytherapy has several limitations, including the requirement for a radiation therapist, a tendency for late “catch-up” restenosis, and inhibition of endothelialization, which markedly increases the risk for thrombosis if another stent is implanted in the same vessel segment. Brachytherapy was found to be inferior to DES placement for treating restenosis in two randomized studies.55,56
BMSs are currently used in 10% to 30% of patients undergoing PCI, most often because of an inability to take long-term dual antiplatelet therapy; in larger (>4.0 mm) vessels, in which the risk for restenosis is lower; and in acute MI, for which the issues related to patient compliance are more difficult to ascertain.
Drug-Eluting Stents
DESs were developed in the early 2000s to provide sustained local delivery of an antiproliferative agent at the site of vessel wall injury. The three components of current DESs are a balloon-expandable stent, a durable or resorbable polymer coating that provides sustained drug delivery, and the pharmacologic agent used to limit intimal hyperplasia.
DESs have proven efficacy in patients with focal, de novo, and “workhorse” lesions that include reference vessel diameters between 2.5 and 3.5 mm and lesion lengths between 15 and 30 mm. Additional randomized trials and registries have also demonstrated the benefit of DESs in patients with long (>30 mm in length) and small (<2.5 mm) vessels, chronic total occlusions, SVG and internal mammary artery disease, in-stent restenosis, and STEMI.57 With the expanded follow-up of patients receiving DESs, it appears that DES placement may require extended (up to 1 year) therapy with a combination of aspirin and clopidogrel to prevent stent thrombosis.58 Moreover, even after 1 year there is a low (0.2% to 0.6%) annual rate of very late stent thrombosis, which warrants a careful discussion of the risks, benefits, and alternative therapies in candidates for PCI. Ongoing trials will determine whether longer (or shorter) durations of therapy are sufficient.59–61 The risk for late and very late stent thrombosis appears to be related to endothelial dysfunction and an abnormal healing response to the vessel wall attributable to the durable stent polymer.62 Although some trials63 and meta-analyses64 of more recently approved DESs with new polymers show a reduction in stent thrombosis when compared with earlier DESs, other large randomized trials have not.65
Sirolimus-Eluting Stents
The CYPHER stent (Cordis, Warren, NJ) contains sirolimus, a naturally occurring immunosuppressive agent that causes cytostatic inhibition of cell proliferation. Sirolimus is released from a biostable polymer over a 30-day period. The pivotal SIRIUS (Sirolimus-Eluting Stent in De-Novo Native Coronary Lesions) trial included 1058 patients with workhorse lesions who were randomly assigned to treatment with a sirolimus-eluting stent or a BMS. The primary clinical endpoint of 8-month target vessel failure, which included target vessel revascularization, death, or MI, was reduced from 21% in patients treated with BMSs to 8.6% in patients treated with sirolimus-eluting stents (P < 0.001). The rate of target vessel revascularization was reduced from 16.6% in BMSs to 4.1% in sirolimus-eluting stents at 1 year (P < 0.001) and was sustained at 5 years.66,67 The cumulative incidence of MI or revascularization attributed to remote segments of the target vessel did not differ between the two groups. Even though manufacture of the CYPHER stent ceased in 2011, other sirolimus-eluting stents continue to be manufactured and sold outside the United States.
Paclitaxel-Eluting Stents
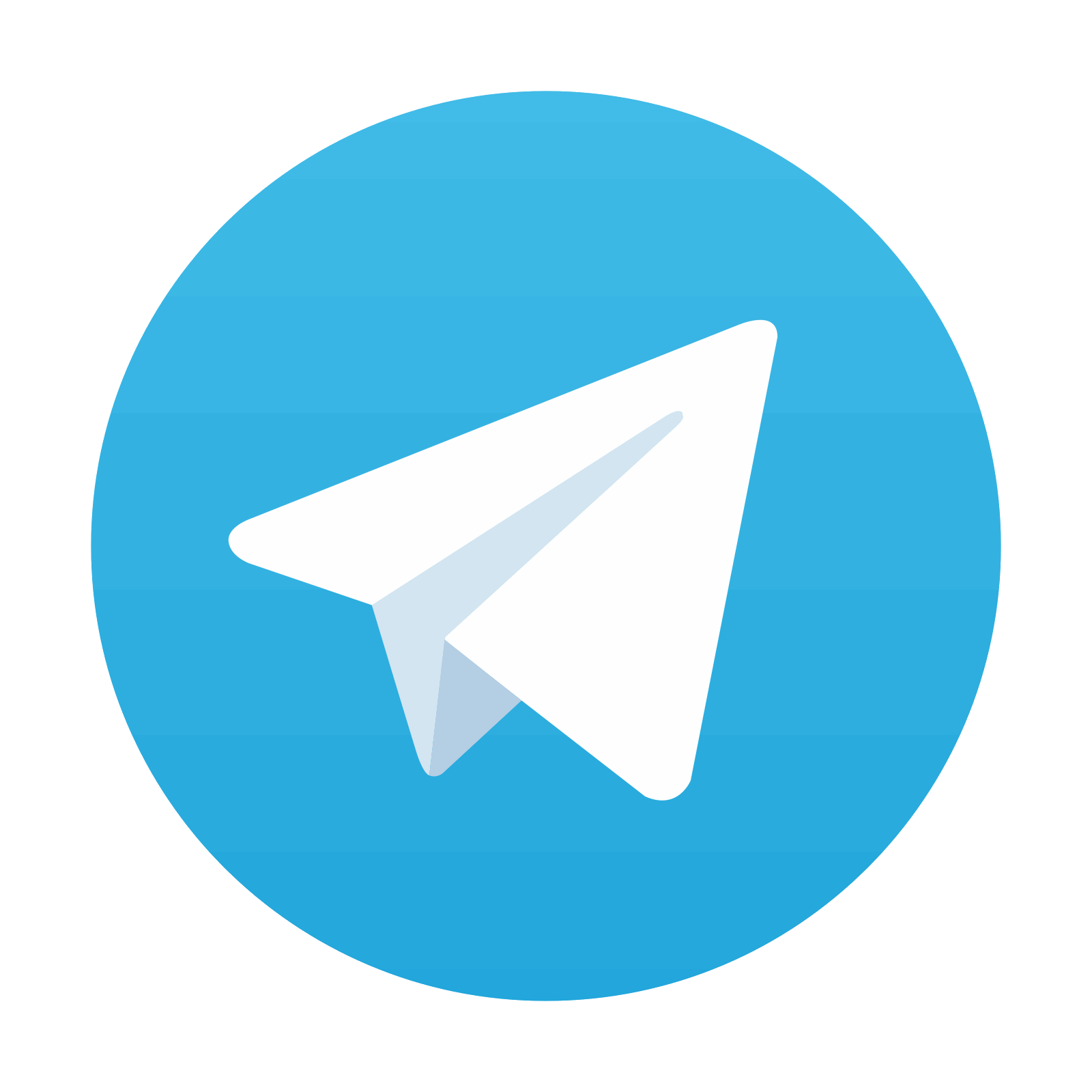
Stay updated, free articles. Join our Telegram channel

Full access? Get Clinical Tree
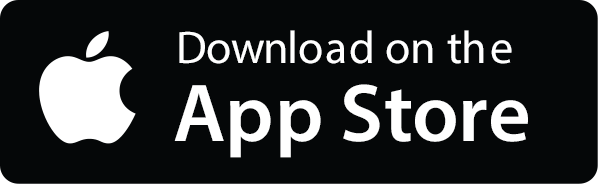
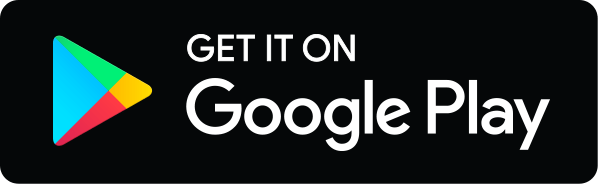
