Chapter 17 Pathophysiology of Peripheral Artery Disease, Intermittent Claudication, and Critical Limb Ischemia
Peripheral artery disease (PAD) is a manifestation of systemic atherosclerosis that commonly coexists with coronary and carotid artery disease. This places the patient with PAD at high risk of cardiovascular events, including myocardial infarction (MI), ischemic stroke, and death.1,2 The pathobiology of atherosclerosis and atherothrombosis has been described previously (see Chapter 8).3,4 The focus of this chapter is on the pathophysiology of atherosclerotic disease in the arteries of the lower extremity that leads to the symptomatic manifestations of PAD, including claudication and critical limb ischemia (CLI). Understanding the pathophysiological mechanisms that underlie the development and progression of limb atherosclerosis and ischemic symptoms is critical in the overall management of the patient with PAD and for the development of potential new therapies. Table 17-1 summarizes the major pathophysiological mechanisms contributing to intermittent claudication and CLI that are reviewed in this chapter.
Table 17-1 Abnormalities Observed in Peripheral Artery Disease
Changes in Pad | Possible Consequences |
---|---|
Hemodynamic | |
Arterial stenosis/occlusion | Pressure drop across stenosis |
Inability to increase flow relative to demand | |
Collateral formation | Partial compensation for arterial stenosis |
Increased blood viscosity | Reduced flow |
Endothelial dysfunction | Altered arteriolar regulation of flow |
Muscle capillary supply | Increased oxygen diffusion |
Oxidant Stress | |
Free radical generation | Endothelial and muscle injury |
White cell activation | Contributes to oxidant injury |
Mitochondrial DNA deletions | Indication of mitochondrial injury |
Structural | |
Distal axonal denervation | Muscle weakness |
Reinnervation | Partial compensation |
Type II fiber loss | Decreased muscle mass/strength |
Metabolic | |
Increased oxidative enzymes | Compensation for reduced oxygen delivery |
Increased short-chain acylcarnitine | Reflects altered oxidative metabolism |
Decreased electron transport | Accumulation related to performance |
Chain activity | Reduced ATP production |
ATP, adenosine triphosphate; DNA, deoxyribonucleic acid; PAD peripheral artery disease.
Clinical Manifestations of Peripheral Artery Disease
The pathophysiology of PAD begins with progressive atherosclerosis, resulting in stenosis and occlusion of the major arteries supplying the lower extremities. Compared with the often acute nature of coronary and carotid atherothrombosis, clinical manifestations of PAD tend to be more chronic and progressive, with primarily functional consequences. Diagnosis of PAD can readily be established through noninvasive hemodynamic assessments. As noted in Chapter 16, the ankle-brachial index (ABI) is the ratio of the systolic blood pressure in the ankle to that in the arm. An ABI of 0.9 take all ABI values to second decimal place, i.e. 0.90 not 0.9 or less establishes a diagnosis of PAD with high sensitivity and specificity when compared with imaging modalities.5
Exercise Limitation and Systemic Risk
Patients with PAD suffer from exercise limitation secondary to impaired hemodynamics. The classic symptom of intermittent claudication is an exercise-induced discomfort in the calf associated with reversible muscle ischemia and relieved by rest. The term claudication is derived from the Latin word claudicato, meaning “to limp,” which is typical of the gait pattern of the patient who experiences claudication when walking. Claudication is characterized by a cramping and aching in the affected muscle. Discomfort develops only during exercise, steadily increases during walking activity to a point where the patient has to stop, and then is quickly relieved by rest without change of position. This sequence of exercise-induced progression and complete relief with rest are important clinical differentiators of claudication from other lower-extremity musculoskeletal conditions. Patients with claudication have severe limitations in exercise performance and walking ability. Compared to healthy individuals of the same age, patients with claudication have a 50% to 60% reduction in peak treadmill performance, reflecting a disability similar to that of patients with severe congestive heart failure (CHF).6 This exercise impairment is associated with a marked decrease in ambulatory activity and the physical dimension of several quality-of-life instruments.7
Although classic claudication symptoms occur in less than a third of patients with PAD, all patients with PAD have reductions in ambulatory activity and daily functional capacity.8 Even “asymptomatic” patients with PAD have a marked reduction in quality of life.9 Thus, major goals of treatment in ambulatory PAD patients are to prevent progression of systemic atherosclerosis and relieve the symptoms of claudication and enhance quality of life.
In addition to physical disability, PAD is a marker of systemic atherosclerotic disease and associated risk. Peripheral artery disease is associated with a three- to sixfold increased risk of coronary artery disease (CAD) and events, cerebral artery disease and stroke, and cardiovascular death.1,10 Thus, consensus guidelines recommend that patients with PAD be considered to have established atherosclerotic disease, and secondary prevention standards are applicable.11,12
Critical Limb Ischemia
Critical limb ischemia is the most severe of the limb manifestations of PAD. Critical limb ischemia is defined by chronic ischemic pain at rest and/or presence of ischemic skin lesions (gangrene or ulcerations). Although its epidemiology is not well known, a recent population study of 8000 individuals between 60 and 90 years of age found the prevalence of CLI to be 1.2%, and more women than men were affected.13 Prognosis for patients with CLI is poor.14,15 In clinical trials enrolling patients with CLI and an ischemic foot ulcer, the annualized risk of death or major amputation ranges from 33% to 50%.15 Independent predictors of worse outcomes include diabetes, end-stage renal disease, and cardiac dysfunction.16,17 Patients with CLI have more severe hemodynamic compromise than patients with claudication, owing to multiple and more distal levels of arterial occlusions. Tibial vessels are most often affected in CLI, usually in combination with disease in the popliteal and superficial femoral arteries (and other more proximal vessels), which leads to a severe compromise of blood flow and oxygen delivery to distal tissues.18 In CLI, ankle pressures typically are less than 50 mmHg. As CLI progresses, skin breakdown to ulceration and gangrene is inevitable. Resting energy expenditure is reduced in CLI compared to intermittent claudication, probably reflecting the fact that these patients become more inactive and sedentary.19 Revascularization to directly address the hemodynamic compromise in CLI remains the primary therapeutic approach.
Hemodynamics in Peripheral Artery Disease
Skeletal Muscle Oxygen Consumption
Muscle oxygen consumption both at rest and during exercise involves oxygen delivery (pulmonary oxygen uptake, oxygen content of hemoglobin (Hb), and regional blood flow) and oxygen extraction by skeletal muscle mitochondria. In healthy persons, maximal muscle oxygen consumption is determined primarily by maximal oxygen delivery, rather than mitochondrial metabolic rate.20 Muscle mitochondrial oxidative capacity remains tightly coupled with maximal exercise capacity and increases with exercise training.21 At the onset of submaximal exercise, skeletal muscle rapidly extracts oxygen from Hb, producing deoxyhemoglobin.22 The kinetics of the changes in tissue oxygen uptake are coupled to systemic oxygen consumption to maintain a balance between oxygen delivery and oxygen utilization.
Determinants of Limb Blood Flow in Healthy Individuals
In healthy persons, exercise is a major stimulus for vasodilation, causing a decrease in peripheral resistance, which when combined with an increase in systemic pressure results in a large increase in arterial flow to skeletal muscle. Normal arteries have the capacity to support large volumetric increases in blood flow without a significant drop in pressure across the large and medium conduit vessels (Fig. 17-1).
Hemodynamic Abnormalities in Peripheral Artery Disease
The arterial occlusive disease process results in fixed-resistance elements in the circulation, and thus initiates multiple pathophysiological processes that manifest clinically as claudication, ischemic rest pain, or ulceration (see Fig. 17-1). Major factors that determine the pressure drop across an arterial stenosis include blood flow velocity and the resistance caused by the stenosis, which in turn is defined by the length and internal radius of the stenosis and blood viscosity. These parameters have been classically described by the Poiseuille equation, which defines the relationships between resistance, pressure, and flow:
where L is length of stenosis, r is internal radius of the artery, and η is viscosity.
This equation makes clear that the radius or cross-sectional area of the stenosis is the primary factor in determining the drop in pressure and flow across a stenosis; a 50% reduction in cross-sectional diameter of the vessel results in a 16-fold increase in resistance. This relationship indicates that as a stenosis worsens, perfusion pressure (and thus the potential pressure that can be dissipated across the stenosis) and the maximal achieved blood flow will decrease dramatically. The dissipation of energy that occurs as blood flow traverses a stenosis is determined in part by the morphology of the stenosis and blood viscosity.23 Pressure drop across the stenosis manifests as reduced limb systolic pressure and decreased ABI as discussed earlier. In patients with PAD, arterial occlusions limit increase in blood flow to exercising muscle. Resting blood flow is usually preserved because of the pressure of arterial collaterals in most patients. In patients with CLI, however, even resting flow may be reduced below normal levels.
Based on the Poiseuille equation, the length of an individual stenosis has only mild impact on blood flow and the pressure gradient. Nevertheless, the hemodynamic effect of two equivalent lesions in series is double that of a single lesion.24 Thus, individual noncritical stenoses may become hemodynamically significant when combined in series in the same limb.25 In CLI, patients typically have disease in the inflow vessels (aorta, iliac arteries) and outflow vessels (superficial femoral, popliteal and tibial arteries).18 These lesions in series create more hemodynamic compromise than is typically seen in claudication.
Critical Artery Stenosis
The hemodynamic significance of an arterial stenosis is not only a function of the percent stenosis, but also linear flow velocity across the lesion, as reflected in the Poiseuille equation.26,27 The term critical artery stenosis is defined as the degree of stenosis that causes a decrease in distal blood flow. The concept integrates the relationship of a stenotic narrowing in an artery with arterial flow velocity and the resultant volumetric flow distal to the stenosis. Importantly, a critical artery stenosis may differ between resting and exercising states because flow velocity in these two conditions is different. Because the pressure gradient across any given stenosis is proportional to the flow velocity, states of higher flow velocity, as occurs with exercise, may result in a decrease in distal perfusion pressure, whereas states of lower velocity, as occurs at rest, may not. For example, resting blood flow velocity in the femoral artery may be only 10 to 20 cm/s, corresponding to a downstream calf blood flow of 1 to 2 mL/100 mL of tissue/min.28 When a large-vessel stenosis of 50% is imposed on the system at this resting flow velocity, loss of kinetic energy across the stenosis may cause no or only a minimal decrease in distal perfusion pressure. Distal flow will be maintained, since the mild reduction in perfusion pressure will be compensated by a reduction in downstream peripheral resistance. Once the stenosis becomes greater than 90%, there is a greater pressure gradient and fall in distal perfusion pressure, and changes in peripheral resistance can no longer compensate. Thus distal flow decreases. In this example, the critical arterial stenosis needed to reduce distal blood flow at rest is 90%.
During walking exercise, blood flow velocity increases—for example, to 150 cm/s. An exercise-induced increase in flow velocity across a 50% stenosis could significantly increase the pressure gradient and reduce distal perfusion pressure. The associated fall in peripheral resistance would be insufficient to compensate for the fall in pressure, and distal blood flow would decrease. Thus the critical arterial stenosis needed to reduce distal blood flow during exercise may be only 50%.28 The concept of critical artery stenosis has clinical significance. In a patient with a single iliac artery stenosis of 50%, the calf blood flow, pedal pulse examination, and ABI may be normal at rest. However, when flow velocity increases with exercise, the same iliac artery lesion becomes hemodynamically significant, resulting in a loss of pedal pulses due to the decrease in ankle pressure distal to the stenosis.
As in patients with CAD, the concept of fractional flow reserve describes the ratio of the blood flow through a diseased coronary artery to the maximal hyperemic flow through that artery in the absence of disease.29,30 This approach is applicable to PAD and provides a functional interpretation of any degree of percent stenosis and the associated critical arterial stenosis. For example, a functional flow reserve of 0.80 indicates a 20% reduction in maximal hyperemic blood flow due to a stenotic lesion. However, minimal luminal area (MLA, or degree of stenosis) correlates poorly with functional flow reserve until the artery disease results in a large reduction in MLA. Thus anatomy per se may not provide sufficient evidence of the functional significance of a particular degree of arterial stenosis.
Blood Flow Response to Exercise in Intermittent Claudication
Most patients with PAD have no limb symptoms at rest (with the exception of those with CLI). This is because resting blood flow is sufficient to meet the relatively low metabolic needs of the tissue, and therefore there is no mismatch between supply and demand to maintain leg oxygen consumption.31,32 At the onset of leg exercise, patients with PAD have an initial rise in leg blood flow and leg oxygen consumption that is delayed.33 With a graded increase in exercise intensity, there is an initial linear increase in flow. However, as exercise intensity increases in PAD, blood flow reaches a plateau because of the limitation imposed by arterial obstructions. This plateau reflects dissipation of energy across the stenotic lesions, removing any additional driving force for increase in flow. Severity of arterial disease (defined by the ABI) correlates inversely with the maximal increase in flow.34 With cessation of exercise, the hyperemic phase (increased flow over resting levels) is prolonged in patients with PAD relative to healthy controls. Despite the plateau in oxygen delivery during exercise, further increases in oxidative work output are supported by increases in muscle oxygen extraction.35 Nonoxidative adenosine triphosphate (ATP) production also contributes to muscle energy metabolism.36 Importantly, muscle ischemia is not simply due to lack of increase in blood flow. The resultant mismatch between the demands for bioenergetics and the flow supply also contribute (see Fig. 17-1).
Other Contributors to Altered Blood Flow in Peripheral Artery Disease
Although arterial flow limitations are of critical importance in the pathophysiology of claudication, the hemodynamic status of the limb correlates poorly with exercise performance. Most studies have shown that resting ankle blood pressure (or ABI) and exercise blood flow do not predict treadmill walking time,37 whereas some studies have shown a weak positive correlation.38,39 This lack of consistent relationship between ABI and claudication-limited exercise capacity is surprising, especially given the relationship between ABI and exercise-induced peak blood flow. Thus, factors distal to the arterial obstruction likely contribute to functional limitations in PAD.
Endothelial Regulation of Flow
Blood flow and its distribution within skeletal muscle beds are determined by endothelial and microcirculatory factors (see Fig. 17-1). Endothelium-derived nitric oxide (NO) is central to the physiological regulation of arteriolar tone. Nitric oxide and prostaglandins (PGs) are major autocrine and paracrine mediators of local vascular resistance during exercise in normal individuals.40–42 Patients with atherosclerosis have a systemic abnormality in endothelial function that is associated with impaired vasodilation and enhanced platelet aggregation.43 A primary mediator of endothelial dysfunction is felt to be oxidant stress from the generation of superoxide anion.44 Consistent with the above, abnormalities in endothelium-dependent vasodilation have been observed in PAD.45 Amputation of an ischemic limb in CLI is associated with improvement of some markers of endothelial function, suggesting local generation of oxidative stress from an ischemic segment of limb.46 Thus, altered oxygen delivery to exercising skeletal muscle in PAD is related not only to the large-vessel occlusive process but also to endothelial dysfunction and impaired vasodilation.
Hemorheology in Peripheral Artery Disease
Peripheral artery disease is associated with altered hemorheology (flow properties of blood and its cellular components) that result in increased viscosity and altered flow as described by the Poiseuille equation (see earlier discussion). Patients with PAD have increased blood concentrations of fibrinogen, von Willebrand factor (vWF), and plasminogen activator inhibitor (PAI), as well as increased fibrin turnover.47 These changes also may affect blood flow characteristics in the microcirculation, but none of these factors has been directly correlated with claudication-limited exercise performance. Previous reports have noted that PAD patients have higher blood viscosity than age-matched controls; according to the Poiseuille law, this could be a contributing factor for exercise-induced ischemia.48 Red cell flexibility is reduced in patients with intermittent claudication, and thus the passage of erythrocytes through nutritive capillaries might be compromised by microcirculatory vessel plugging.49
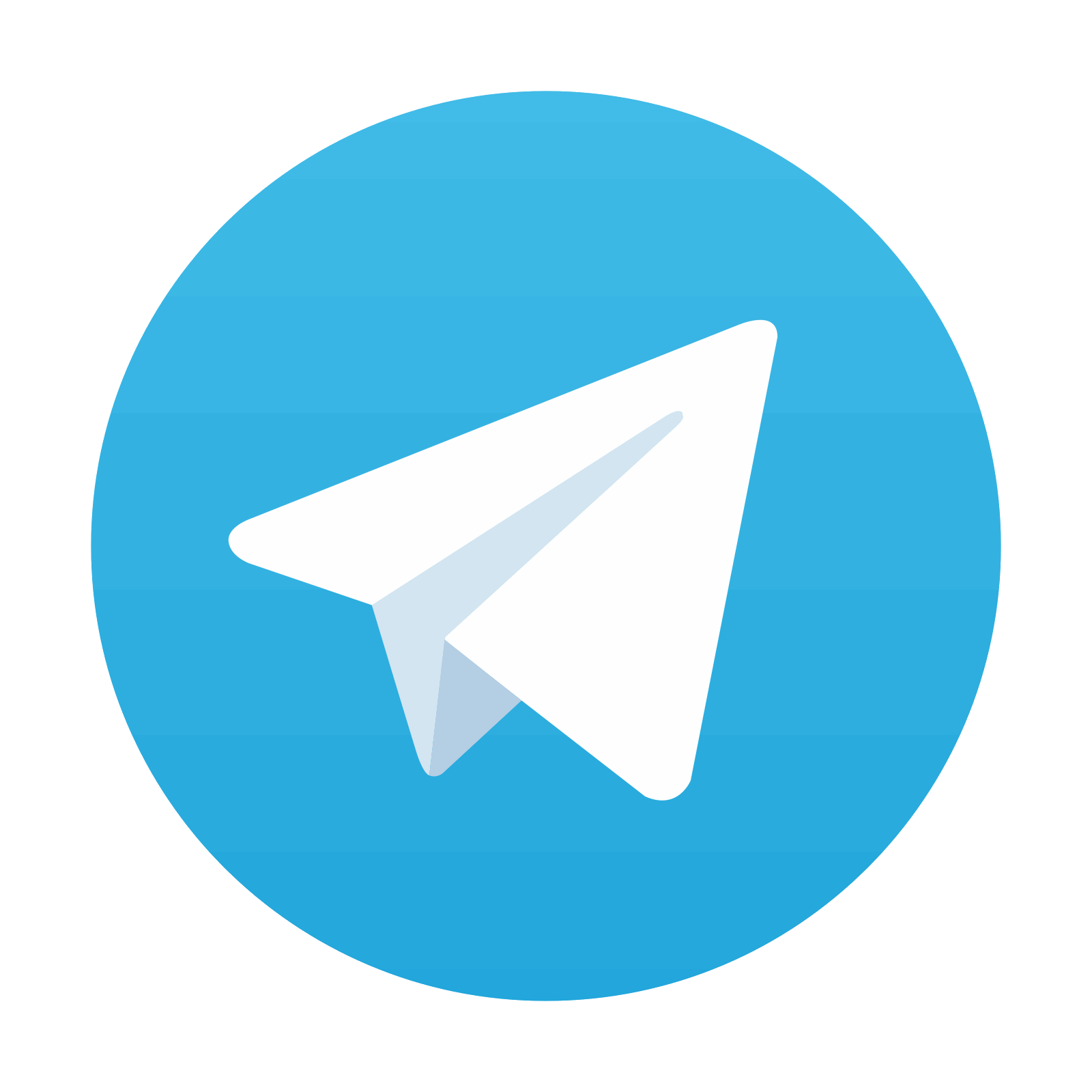
Stay updated, free articles. Join our Telegram channel

Full access? Get Clinical Tree
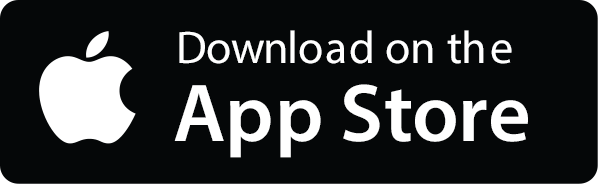
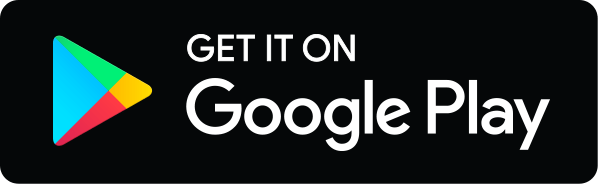