In this chapter, the impact of a patent ductus arteriosus (PDA) will be discussed in the clinical setting of a neonate with an otherwise structurally normal heart (± a patent foramen ovale). Specific emphasis will be placed on preterm infants with relatively low pulmonary vascular resistance and ductal shunting that is predominantly or completely left to right.
Until recently, because the harmful effects of a PDA were thought to be self-evident, the prevailing opinion was that PDA closure was indicated in all preterm infants. The predominant question was the best method to close a PDA in such patients, and this was the subject of numerous randomized clinical trials.1,2 However, over the last decade, the rationale for the treatment of a PDA, in general, and ligation, in particular, has been increasingly challenged. The current trend is to recommend a less aggressive approach to the pharmacologic and surgical treatment of a PDA. This in turn has made the recognition of a hemodynamically significant patent ductus arteriosus (hsPDA) even more important. In this chapter, the hemodynamic effects of a PDA and its ligation will be reviewed, followed by a detailed description of the echocardiographic indices that can be helpful in identifying an hsPDA. Finally, the use of PDA flow to estimate relative pulmonary vascular resistance in the setting of pulmonary hypertension will be briefly discussed.
In the presence of a PDA with left-to-right shunting, pulmonary blood flow increases, leading to an increase in pulmonary venous return to the left atrium (LA). This increase in LA volume can augment the left ventricular (LV) preload, provided no significant shunt at the patent foramen ovale (PFO) is present (see below). The increase in LV preload will increase the stroke volume according to the Starling curve.3–6 However, a PFO can significantly alter the effects of a PDA on LV stroke volume by decompressing the LA. Indeed, in the presence of a significant PFO (diameter >4 mm), right ventricular output (RVO) can even be greater than left ventricular output (LVO) despite the presence of a significant left-to-right PDA shunt.7 Despite the inherent vulnerability of the immature myocardium and the potential for decreased myocardial perfusion due to low diastolic pressure, myocardial contractility appears not to be affected by an hsPDA. With short exposure (days) to an hsPDA, both load-dependent (LV shortening fraction, or SF) and load-independent (stress-velocity index) measures of systolic function are found to be normal.8
In the presence of a PDA, the heart rate does not change; therefore, the increase in LVO is solely the result of an increase in stroke volume. As systolic blood pressure is primarily affected by changes in stroke volume and diastolic blood pressure is mainly reflective of changes in systemic vascular resistance (SVR), the PDA-associated increase in stroke volume and decrease in SVR causes systolic blood pressure to be maintained or minimally decreased compared to a significant drop in diastolic blood pressure. This results in a wide pulse pressure, the hallmark of an hsPDA. However, in the first few days of postnatal life, both systolic and diastolic blood pressure can be affected to the same degree, thereby maintaining an unchanged pulse pressure.9,10 This may be the result of inadequate compensatory increase in stroke volume and/or low amount of left-to-right PDA shunting during the early postnatal period. In animals, a left-to-right PDA shunt decreases diastolic and mean blood pressure, while only a moderate to large left-to-right PDA shunt reduces systolic blood pressure.4
The increase in LVO may compensate for the shunt, but as discussed below, this compensation may not be adequate and distal organ blood flow distribution is often significantly altered. Animal studies suggest that systemic blood flow is redistributed even in the presence of a small PDA.4 The effect of blood flow diversion from the “steal phenomenon” of an hsPDA on organ blood flow varies. In general, blood flow to the brain is preserved better than any other organ. Skin, bone, and skeletal muscle are among the first organs to be adversely affected by an hsPDA. Next, the blood flow to the gastrointestinal system and kidneys is compromised. Importantly, organ blood flow can be compromised without any signs of left ventricular failure.11,12
Pulmonary blood flow is derived from RVO and supplemented by the left-to-right PDA shunt (both during systole and diastole). In the presence of an hsPDA, the systemic flow (effective left cardiac output) can be compromised, leading to a decrease in venous return to the right side of heart and consequently a decrease in RVO. Despite this decrease in RVO, the net pulmonary blood flow increases in the presence of a left-to-right PDA shunt. Furthermore, with a significant PFO or atrial septal defect, the RVO is maintained despite a reduction in systemic venous return. In fact, with a significant PFO, the larger the PDA, the greater will be the RVO.
The PDA effect on cerebral blood flow (CBF) varies from one patient to another, depending upon the adequacy of a compensatory increase in left ventricular output and decrease in cerebral vascular resistance. In most patients, the compensatory increase in stroke volume results in an increase in CBF during systole,12,13 and the steal phenomenon leads to a decrease, absent, or reversal of flow during diastole.14,15 The net effect is an abnormal flow pattern but maintenance of a normal amount of CBF. Interestingly, in a subset of infants, there appears to be an effective compensatory vasodilation in the brain, as evidenced by a normal diastolic flow pattern in the large cerebral arteries.
The compensatory increase in stroke volume appears to be the key in maintaining CBF.16 This compensatory mechanism may not be fully in place in the first few hours after birth. In term neonates, during the first 20 minutes after birth, there is an strong inverse relationship between middle cerebral artery (MCA) mean velocity (a surrogate for CBF) and the net left-to-right shunt through the PDA.17 Similarly, in preterm infants, a significant PDA is an independent predictor of low superior vena cava flow, a surrogate for CBF, only during the first 12 hours after birth.18 In other words, the adverse effect of a PDA on CBF is most prominent during the early postnatal period before the compensatory increase in LVO is yet fully operational.
Blood flow to the abdominal organs is decreased in the presence of an hsPDA. Even a small ductal shunt has been shown to cause significant reductions in blood flow to the abdominal organs in preterm lambs.4 Assessment of superior mesenteric and celiac arteries by Doppler shows a decrease in flow as well as an abnormal pattern: a decreased, absent, or reversed flow during diastole.15,19,20 Importantly, this reduction in abdominal organ blood flow occurs despite a compensatory increase in LVO.12 Therefore, while a compensatory increase in LVO might maintain adequate CBF (albeit with an abnormal pattern), it does not result in normal blood flow to the organs supplied by the abdominal aorta. Interestingly, in premature primates, not only is the baseline intestinal blood flow decreased, but also the normal postprandial increase in intestinal flow is blunted.21
Similar to SMA flow, renal blood flow is affected by an hsPDA. A reduced overall flow with absent or reversed diastolic flow mark the effect of an hsPDA on renal artery flow.
Up to 45% of the preterm infants undergoing surgical ligation of their PDA develop a period of cardiopulmonary deterioration after the procedure.22 This deterioration—marked by hypotension, increased vasopressor and/or inotrope treatment, and increased respiratory support—usually presents a few hours after the procedure, with the patient’s condition improving by 24 to 48 hours.22–28 While the exact cause of this deterioration is not clear, cardiac dysfunction and adrenal insufficiency play a role, as will be discussed below.22,24,27,29
With ligation of a PDA, the LV preload decreases, resulting in a reduction in LVO. The heart rate does not change after ligation. Therefore, the decrease in LVO is solely due to a decrease in stroke volume. In the presence of a PDA, the vascular resistance against which the LV pumps includes both the systemic and pulmonary vascular beds. Following ligation of the PDA, the contribution of the low-resistance pulmonary vascular bed is eliminated; therefore, the calculated vascular resistance increases. However, despite the increase in vascular resistance, the LV afterload, as assessed by the wall stress, does not change.24,30 The lack of increase in afterload is due to the fact that afterload depends on blood pressure, ventricular diameter, and ventricular wall thickness (see Chapter 8). Therefore, the increase in blood pressure due to elevated vascular resistance is counterbalanced by the reduction in LV diameter and increase in ventricular wall thickness as a result of decreased LV preload.24 Regarding myocardial contractility, overall there are no significant changes in either load-dependent (SF, corrected velocity of circumferential fiber shortening) or load-independent (stress-velocity index) measures of contractility.24,30 Among those presenting with hemodynamic instability after ligation, some have poor systolic function (low SF and cardiac output), while others have primarily vasodilation most probably secondary to relative adrenal insufficiency.22,29 A very small subset of patients presents with a dramatic reduction of the interventricular septal wall motion after ligation (Figures and Videos 11-1 and 11-2).1 More commonly, a subtle deterioration in global myocardial function is noted by measurement of the myocardial performance index (MPI) immediately after ligation, with partial recovery by 24 hours.24 This deterioration in myocardial performance might be reflective of “resetting” the set point of the Starling curve of the myocardium after a prolonged period of stretch so that the decrease in end-diastolic fiber length following ligation will result in a decrease in contractile force. Therefore, the post-ligation decrease in LVO results not only from a decrease in preload but also as a consequence of transient myocardial dysfunction. In other words, after ligation, the LVO decreases in excess of what is expected by removal of the shunt alone. It is interesting that the pattern of changes in MPI mimics the changes in LVO (Figure 11-3). Furthermore, it was the MPI—rather than the preload, contractility, or afterload—that was most predictive of the LVO after ligation.24 It is possible that MPI is more sensitive than conventional indices of cardiac function. Similarly, decreased LV performance, as defined by either a LVO of <170 mL/kg/min, fractional shortening of <25%, or systolic blood pressure less than the 3rd percentile, was found to be prevalent in patients with hemodynamic instability at 8 hours after PDA ligation.27
FIGURE and VIDEO 11-1.
Parasternal short-axis view of the left ventricle at the level at the tips of mitral valve in an extremely preterm infant with a large PDA. The left ventricle is dilated as the result of the high preload secondary to the left-to-right PDA shunting. Note the normal motion of intraventricular septum during systole and diastole in M-mode. Abbreviations: RV, right ventricle; LV, left ventricle.

FIGURE and VIDEO 11-2.
Parasternal short-axis view of the left ventricle at the level of the tips of mitral valve in the extremely preterm infant (same patient as in Figure and Video 11-1) after ligation of the large PDA. The left ventricular cavity is small and the M-mode tracing clearly demonstrates the minimal motion of the intraventricular septum during the cardiac cycle. Abbreviations: RV, right ventricle; LV, left ventricle.

FIGURE 11-3.
Changes in left ventricular output (LVO) and myocardial performance index (MPI) after PDA ligation are shown. Note that changes in MPI, a measure of global function, follow a similar pattern to the changes in LVO, supporting the notion that myocardial dysfunction may have played a role in the drop in cardiac output after ligation. (Data from Noori S, Friedlich P, Seri I, et al. Changes in myocardial function and hemodynamics after ligation of the ductus arteriosus in preterm infants. J Pediatr. 2007 Jun;150(6):597-602.)

Given the complexity of the effect of ligation on cardiac function and the significant incidence of cardiovascular compromise, assessment of cardiovascular function by echocardiography can be helpful in guiding the treatment strategy in these patients.
The effects of ligation on CBF depend upon the pre-ligation CBF pattern and post-ligation cardiovascular function. As discussed earlier, the typical cerebral artery Doppler pattern in which the LV has compensated for the left-to-right PDA shunt shows high systolic flow and a reduced, absent, or reversal flow in diastole. With the decrease in stroke volume following ligation, and the accompanying increase in SVR, generally the systolic flow decreases while the diastolic flow increases. Depending on the relative changes in flow during the systole and diastole, mean or total blood flow can increase, decrease, or stay the same.
In most cases, prior to ligation, the compensatory increase in stroke volume has resulted in normal CBF; following ligation, the relative changes in systolic and diastolic flow cancel each other out, with the net result being little or no change in mean blood flow velocity. Conversely, in a small subset where pre-ligation compensatory increase in CBF during systole was not present prior to ligation, the improvement in diastolic flow would then result in an increase in CBF after ligation. Furthermore, in a subset of preterm infants, the CBF decreases post-ligation either due to an excess of pre-ligation systolic flow or because of post-ligation myocardial dysfunction and/or hypotension. Therefore, CBF status after ligation depends on the degree of pre-ligation compensatory increase in CBF and whether or not post-ligation cardiovascular compromise develops. This might explain some of the inconsistency in the literature on changes in CBF after ligation.31–37
With the removal of a left-to-right PDA shunt, RVO increases but the total pulmonary blood flow decreases. The flow to all other organs, except for the brain, improves after ligation, provided no significant post-ligation cardiovascular compromise develops. The improvement in flow is primarily the result of an increase in diastolic blood flow.33
The definition of hsPDA remains controversial. A hsPDA is a PDA that alters organ blood flow and could potentially contribute to pathogenesis of common disease of prematurity such as bronchopulmonary dysplasia and necrotizing enterocolitis. As discussed above, a PDA can have a variable effect on different organs. The symptomatology and adverse hemodynamic effect depends upon the size of the ductus arteriosus, the relative pulmonary to systemic resistance, and compensatory mechanisms within the heart and the other organ(s). While a PDA might be “significant” for the lung (ie, cause pulmonary edema), it might not be “significant” for the brain or kidney. On the other hand, a PDA might have minimal effects on the lung, yet have a profound effect on the gut or renal blood flow. Therefore, whenever one asks the question as to whether a PDA is hemodynamically significant, one should always clarify the target organ in question. Moreover, while the potential hemodynamic effect of a PDA on organ blood flow can be evaluated, it can be difficult to assess the adequacy of the organ’s compensatory mechanisms.
Among the indicators of an hsPDA discussed below, ductal diameter has been consistently shown to be the most important parameter. However, measurement of ductal diameter is often inadequate as the sole indicator of hemodynamic significance of a PDA. Other indices of hsPDA, when used in conjunction with ductal diameter, can help predict the degree of shunt.38 To determine the best management strategy for an hsPDA, one needs to use multiple echocardiographic indicators39,40 and consider these in the context of the clinical setting, gestational and chronological age, vulnerability of the target organ(s), and the risks and benefits of a treatment regimen.
The patient should be placed supine. If the patient’s condition allows, the placement of a roll underneath the shoulders can facilitate neck extension and provide a better access to the suprasternal window. Otherwise, turning the head to the side can be helpful.
For better resolution, a high-frequency (10–12 MHz) probe is preferable.
The ductus arteriosus can best be assessed from suprasternal or high parasternal short-axis views.
In the suprasternal view, after visualization of the aortic arch, place the color box over the distal part of the arch and rotate the transducer slightly counterclockwise and tilt anteriorly. This maneuver should enable visualization of the ductus arteriosus, descending aorta and the main pulmonary artery (Figure and Video 11-4). With a slight adjustment in position and orientation of the transducer, the ductus arteriosus can be seen in its entirety (Figure and Video 11-5). Further minor adjustments will yield the “three fingers”: the PDA (uppermost), and the left (middle) and right (bottom) pulmonary arteries (Figure and Video 11-6). Alternatively, the PDA can be visualized from the high left parasternal parasagittal view (“ductal view”) or the high parasternal short-axis view. Depending on the direction of the shunt, the color flow can be blue (right-to-left; Figure and Video 11-7), red (left-to-right; Figure and Video 11-8), or blue and red (bidirectional; Figure and Video 11-9). Adjust (increase) the color scale to minimize oversaturation and reduce aliasing. However, when the flow velocity is low, too high of a scale will lead to poor visualization of ductal flow (Figure and Video 11-10). Next, using pulsed wave (PW) Doppler, obtain a spectral Doppler flow envelope by placing the Doppler gate at the narrowest portion of the PDA. Since PW Doppler allows for estimation of flow velocity at a specific location (range resolution), it is the preferred mode of Doppler interrogation. However, when aliasing occurs because the flow velocity is too high (eg, with ductal constriction), continuous-wave (CW) Doppler should be used to quantify accurately the velocity across the PDA.
FIGURE and VIDEO 11-4.
To visualize the PDA from the suprasternal view, first identify the aortic arch. Then with a slight counterclockwise rotation and anterior angulation, the operator will view the ductus arteriosus, as shown in this video clip. Further anterior angulation and minor adjustment of the transducer will yield the pulmonary artery and the ductus in its entirety (Figure and Video 11-5).

FIGURE and VIDEO 11-8.
A large PDA with a left-to-right shunt (red color) in an extremely preterm infant at 16 hours after birth.
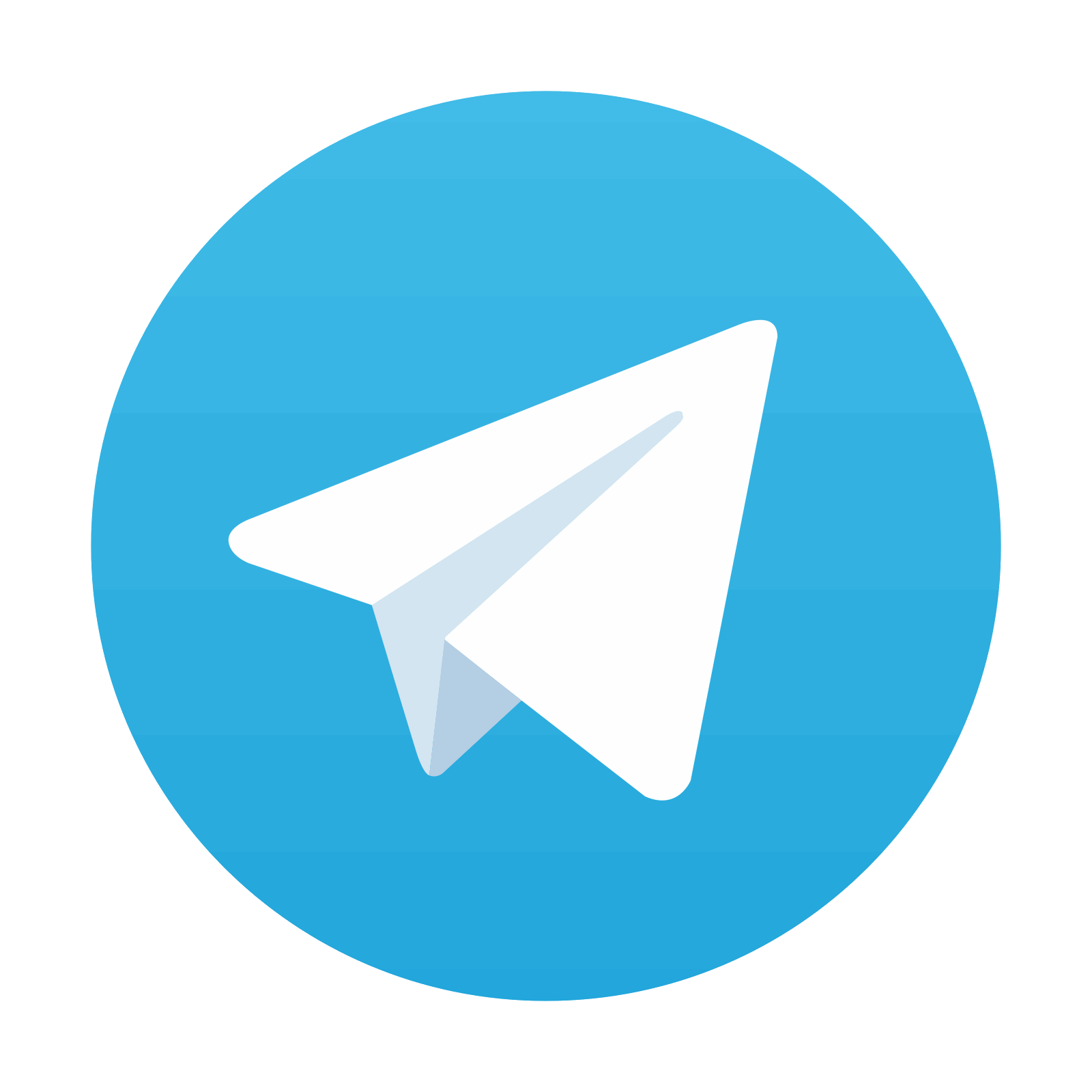
Stay updated, free articles. Join our Telegram channel

Full access? Get Clinical Tree
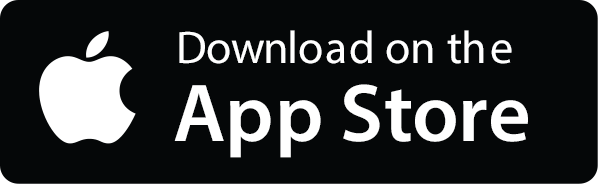
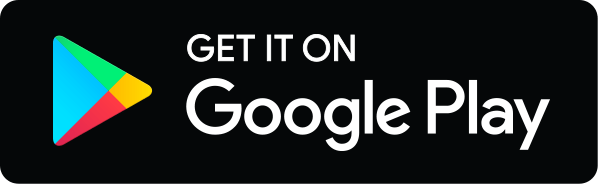
