Fig. 21.1
A normal right arterial tree (except for occlusion of the right common iliac artery) beginning with the common iliac artery down to the pedal branches. The left side shows occlusion of the left common iliac artery, stenosis of the left external iliac artery, and occlusion of the left superficial femoral artery, popliteal artery, and diseased tibioperoneal trunk
Collateral Circulation of the Lower Extremity
In the event of chronic obstruction of major arterial vessels, collateral pathways exist that allow preservation of sufficient distal blood flow to maintain viability of the tissues distally. The degree of adequacy of these pathways determines what degree of functional disability results.
With obstruction at the level of the distal aorta and common iliac arteries, a variety of pathways for collateral circulation exist (Fig. 21.2). Communications may exist between the lumbar and circumflex iliac or hypogastric arteries. Other communications may exist between the gluteal branches of the hypogastric arteries and recurrent branches of the common femoral or profunda femoris arteries. Visceral–parietal communications may also exist at this level between the inferior mesenteric and internal iliac vessels via hemorrhoidal branches at the level of the rectum.




Fig. 21.2
(a) Collateral circulatio n of the left lower extremity secondary to occlusion of the major arterial segments as noted in Fig. 21.1. (b) Arteriogram showing complete occlusion of the left common iliac artery (arrow). Note the collateral circulation around the obstruction. This also shows extensive disease of both right and left external iliac arteries
More distally, with obstruction of the common femoral artery, collateral circulation around the hip is provided via communication of the inferior epigastric and deep circumflex branches of the external iliac arteries with the internal pudendal and obturator branches of the internal iliac arteries (Fig. 21.2b).
With chronic obstruction of the superficial femoral artery, collateral circulation to the popliteal artery is provided by communications with the profunda femoris artery via the geniculate arteries, as well as descending branches of the lateral femoral circumflex arteries. With popliteal occlusion, it is these geniculate arteries that are responsible for the filling of the more distal tibial vessels as well (Fig. 21.2). More distally, branches of the peroneal, anterior tibial, and posterior tibial arteries all provide collateral supply to the plantar arch vessels [1, 2].
Normal Structure of the Arterial Wall
The intima, the innermost layer, consists of a layer of endothelium that lines the luminal surface and overlies one or more layers of smooth muscle. These are then covered by a layer of connective tissue known as the internal elastic lamina.
Just beyond the internal elastic lamina begins the media, which is bounded by the internal and external elastic lamina. The media is composed of smooth muscle cells arranged in layers and lying in a matrix of proteoglycan substance. Collagen and elastin fibers are also present within this layer.
The adventitia is the outermost layer of the arterial wall and the layer responsible for the majority of the vessel’s strength. It is composed of connective tissue, fibroblasts, capillaries, neural fibers, and occasional leukocytes. In large vessels a microvasculature known as the vasa vasorum is present within the adventitial layer, serving to nourish the adventitia and outermost layers of the media [3].
Incidence/Prevalence of Peripheral Arterial Disease
The overall prevalence of peripheral arterial disease (PAD) based on objective data has been evaluated in several epidemiological studies [4–6] and has been demonstrated to be a global problem with the number of people with PAD increased by 23.5% from the year 2000 to 2010 [6]. The prevalence of PAD increases with age in the range of 3–10%, in the general population which increases to 10–20% in people over the age of 70 years [4–6]. Peripheral arterial disease affects up to one in six individuals older than 80 years old [6]. The prevalence of asymptomatic PAD of the lower extremity can only be estimated using noninvasive vascular testing in the general population with the ankle-brachial index (ABI) being the most widely used testing. A resting ABI of ≤0.9 is generally caused by hemodynamically significant arterial stenosis and most often is used as a hemodynamic definition of PAD. This definition in symptomatic individuals (ABI ≤0.9) is 95% sensitive in detecting PAD confirmed by angiography and 100% specific in identifying healthy individuals [4]. The PARTNERS (PAD Awareness, Risk, and Treatment: New Resources for Survival) study, which screened 6979 patients for PAD who were aged 70 years or older or aged 50 through 69 years with history of cigarette smoking or diabetes using an ABI of ≤0.9 or a prior history of lower extremity revascularization, reported the presence of PAD in 29% of the total population [7]. Classic vascular claudication was present in 5.5% of the newly diagnosed patients with PAD versus 12.6% of patients with a prior diagnosis of PAD [7].
The prevalence of symptomatic PAD, i.e., intermittent claudication, would appear to increase from about 3% in patients aged 40 to 6% in patients aged 60 years. Several large population studies have analyzed the prevalence of intermittent claudication as noted in Fig. 21.3. As noted in this figure, the prevalence in patients between 30 and 34 years is less than 1%, which increases to approximately 7% for patients between 70 and 74 years. It has also been reported that in relatively younger age groups, claudication is more common in men, but at an older age, there is little difference between men and women. It has also been reported that black ethnicity increases the risk of PAD by over twofold, which cannot be explained by a higher level of other risk factors for PAD [4].


Fig. 21.3
Weighted mean prevalence of intermittent claudication (symptomatic PAD) in large population-based studies. From Norgren L, Hiatt WR, Dormandy JA, et al. Inter-Society Consensus for the Management of Peripheral Arterial Disease (TASC II). Journal of Vascular Surgery, 2007;45:S5A–S67A. Reprinted with permission from Elsevier
Risk Factors
Through epidemiologic data, a number of risk factors for the development of atherosclerosis have been reasonably well established. These factors include hypertension, hypercholesterolemia, cigarette smoking, obesity, diabetes mellitus, stress, sedentary lifestyle, and family history.
Age
As noted in Fig. 21.3, there is a striking increase in both the incidence and prevalence of PAD with increasing age [4].
Merino et al. analyzed the incidence and risk factors of PAD in a prospective cohort of 700 adult elderly men followed for 5 years and concluded that 12% of adult men aged between 55 and 74 years developed PAD. Besides subjects with a history of cardiovascular disease, men older than aged 70 years and heavy smokers constituted a high-risk group for PAD and, therefore, the object of directed efforts of primary prevention [8].
Gender
The prevalence of PAD is slightly higher in men than in women, particularly in the younger age group. The ratio of men to women in patients with intermittent claudication ranges between 1:1 and 2:1. This ratio increases in some studies to at least 3:1 in individuals with a severe stage of PAD [4].
Smoking
Intermittent claudication is three times more common among smokers than non-smokers. It has also been suggested that the association between PAD and smoking is even stronger than between coronary artery disease and smoking. A diagnosis of PAD is made approximately a decade earlier in smokers than in non-smokers, and the severity of PAD tends to increase with the number of cigarettes smoked. Smoking cessation is also associated with a decline in the incidence of intermittent claudication, as reported by the Edinburgh Artery Study [9], which concluded that the relative risk of intermittent claudication was 3.7 in smokers, compared with 3.0 in previous smokers (who quit smoking for less than 5 years).
Diabetes Mellitus
Intermittent claudication is twice as common among diabetic patients as among nondiabetics. It has also been estimated that for every 1% increase in hemoglobin A1c, there is a corresponding 26% increased risk of PAD [10]. Insulin resistance plays a major role in clustering of cardiometabolic risk factors, which include hypertension, dyslipidemia, hyperglycemia, and obesity. Insulin resistance is also a risk factor for PAD in patients without diabetes, raising the risk to 40–50% [11]. PAD in patients with diabetes mellitus is also more aggressive, compared to nondiabetics with early large vessel involvement combined with distal small vessel disease and neuropathy. Major amputation is also 5–10 times higher in diabetics than in nondiabetics. Based on these findings, a consensus statement from the American Diabetes Association recommended PAD screening with an ABI every 5 years in diabetic patients [12]. There is strong evidence that better control of blood glucose delays the onset of microvascular diabetic retinopathy and nephropathy; however, the effect of such control in progression of macrovascular disease remains controversial [13].
Dyslipidemia
A fasting cholesterol level greater than 270 mg/dl was associated with a doubling of the incidence of intermittent claudication, based on the Framingham study. However, the ratio of total to high-density lipoprotein cholesterol was the best predictor of occurrence of PAD. Although some studies have shown that total cholesterol is a powerful independent risk factor for PAD, others have failed to confirm this association [4]. An association between hypertriglyceridemia and PAD has also been noted and has been shown to be associated with progression/complications of PAD. Lipoprotein (a) has also been shown to be a significant independent risk factor for PAD and has been demonstrated to be higher in patients with critical limb ischemia (CLI) than patients with intermittent claudication (IC) [14].
Hypertension
Hypertension is associated with all forms of cardiovascular disorders, e.g., PAD. However, it should be noted that hypertension carries less relative risk of developing PAD than diabetes or smoking (Fig. 21.4). The role of hypertension in the development of peripheral arterial disease has been controversial, with the Framingham and the Finnish studies arriving at opposite conclusions. Hypertension may have both a cause and effect relation to peripheral arterial disease. Aggressive blood pressure control in newly diagnosed hypertensive patients occasionally decreases perfusion sufficiently to unmask and recognize hemodynamically significant stenotic lesions.


Fig. 21.4
Approximate range of odds ratios for risk factors for symptomatic peripheral arterial disease. From Norgren L, Hiatt WR, Dormandy JA, et al. Inter-Society Consensus for the Management of Peripheral Arterial Disease (TASC II). Journal of Vascular Surgery, 2007;45:S5A–S67A. Reprinted with permission from Elsevier
Hyperhomocysteinemia
The prevalence of hyperhomocysteinemia is higher in patients with PAD compared to the general population [15]. Hyperhomocysteinemia is detected in about 30% of young patients with PAD, and it is believed to be an independent risk factor for atherosclerosis. It has also been felt to be a stronger risk factor for PAD than for CAD [4]. Unfortunately, the effect of folate supplementation on patients with PAD does not have robust evidence of clinically beneficial effects [15].
Inflammatory Markers/C-Reactive Protein
Recent studies have shown that C-reactive protein (CRP ) is higher in asymptomatic subjects who developed PAD in the subsequent 5 years, compared to an age-matched control group who remained asymptomatic. The risk of developing PAD in the highest quartile of baseline CRP was more than twice that in the lower quartile [16].
Chronic Renal Insufficiency
Hypercoagulable States
Hyperviscosity and raised hematocrit have been noticed in patients with PAD, which may be a consequence of smoking. Increased plasma fibrinogen levels have also been associated with PAD in some studies [4].
Race
PAD , as defined as an ABI of ≤0.9, was more common in non-Hispanic blacks (7.9%) than in whites (4.4%), based on the National Health and Nutrition Examination Survey in the United States [5]. This was also confirmed by the GENOA (Genetic Epidemiology Network of Arteriopathy) study [19].
Finally, a family history has not been found to be a significant independent risk factor in the development of peripheral arterial disease, in contrast to patients with coronary artery disease. Figure 21.4 demonstrates the approximate range of odds ratio for risk factors for symptomatic PAD.
Alzamora et al. reported on the PAD study (PERART/ARTPER) and the prevalence and risk factors in the general population. They performed a cross-sectional, multicenter, population-based study in 3786 individuals aged over 49 years, randomly selected in 28 primary care centers in Barcelona, Spain. Patients were diagnosed as having PAD if their ABI was <0.9. The prevalence of PAD was 7.6% (10.2% for males and 5.3% for females). A multivariate analysis showed the following risk factors: male sex (odds ratio [OR] 1.62), age (OR 2.00 per 10 years), inability to perform physical activity (OR 1.77 for mild limitation to OR 7.08 for breathless performing any activity), smoking (OR 2.19 for former smokers and OR 3.83 for current smokers), hypertension (OR 1.85), diabetes (OR 2.01), previous cardiovascular disease (OR 2.19), hypercholesterolemia (OR 1.55), and hypertriglyceridemia (OR 1.55). Body mass index ≥25 kg/m2 (OR 0.57) and walking more than 7 h/week (OR 0.67) were found as protector factors. They concluded that the prevalence of PAD is low, higher in males, and increases with age in both sexes. In addition to previously described risk factors, they found a protector effect in physical exercise and also with being overweight [20].
Association of Peripheral Arterial Disease, Coronary Artery Disease, and Cerebrovascular Disease
Pooling evidence from available studies, the Transatlantic Intersociety Consensus (TASC) concluded that approximately 60% of patients with peripheral arterial disease have significant coronary artery disease , cerebrovascular disease , or both, whereas about 40% of those with coronary artery or cerebrovascular disease also have peripheral arterial disease [21]. Murabito et al. [22] reported that the diagnosis of concomitant coronary artery disease can be established with a clinical history, physical examination, and EKG in 40–60% of all patients with intermittent claudication. The 4-year risk of myocardial infarction for a patient with intermittent claudication is 6.6% and ranges from 9.4–10.9% for a patient with critical limb ischemia [23]. Also concerning is that the 4-year risk of ischemic stroke in a patient with PAD is 5.4% for a patient with intermittent claudication and ranges from 7.4%–9.1% for a patient with critical limb ischemia [23].
Atherosclerosis
Pathophysiology
Intermittent claudication as a symptom of peripheral arterial disease can be caused by flow-limiting stenosis, which is almost always secondary to atherosclerosis. Whether or not a stenotic lesion is flow limiting depends on both flow velocities and the degree of stenosis [24]. Flow velocity at rest has been estimated to be as low as 20 cm/s in the femoral artery. A diameter reduction of >90% would be required for a stenotic lesion at these rates to be considered hemodynamically significant. However, the metabolic requirements in the distal tissue of an exercising or active individual are higher, and the femoral artery velocities may increase up to 150 cm/s, and at this velocity level, a stenosis of 50% can cause significant pressure and flow gradient leading to inadequate oxygen delivery. In general, patients with mild intermittent claudication typically have a single segment disease, which is often associated with well-developed collateral circulation, in contrast to patients with severe claudication or critical limb ischemia, which is associated with multilevel disease.
The hemodynamic abnormalities of peripheral arterial occlusive disease reflected in ankle-brachial index (ABI) measurements or direct measurement of calf blood flow do not necessarily correlate with walking performance or severity of the claudication symptoms [25]. Biochemical changes and microcirculatory changes induced by the cycle of ischemia and reperfusion have been suggested as evidence of this observation. This may lead to skeletal muscle injury due to distal axonal degeneration, which, in turn, may cause muscle atrophy, further compromising exercise tolerance. This injury may be mediated at the cellular level through increased oxidative stress, generation of oxygen-free radicals, and lipid peroxidation that occurs during reperfusion of ischemic tissue. Several studies have demonstrated the accumulation of several metabolic intermediates, such as acylcarnitines, impaired synthesis of phosphocreatinine, and supranormal levels of adenosine diphosphates [26]. Patients with advanced chronic peripheral arterial disease have an abundance of these antimetabolic compounds, which signify well-established metabolic myopathy. Increased acylcarnitine accumulation has been noted to correlate well with decreased treadmill exercise performance [27].
The basic underlying disease process affecting the arterial wall, and the one responsible for the clinical manifestations of lower extremity peripheral arterial disease, is atherosclerosis. Simply put, atherosclerosis is a disease of arterial vessels that causes luminal narrowing, thrombosis, and occlusion resulting in ischemia of the end organ involved. The process of atherosclerosis is extremely complex and is the subject of continuous investigation within the medical and surgical community. In addition to lower extremity vascular disease, atherosclerosis is known to produce many other clinical events of importance including, but not limited to, myocardial infarction, stroke, mesenteric vascular insufficiency, and aortic aneurysm for mation [28].
Atherosclerosis is primarily a disease of the intima characterized by the proliferation of smooth muscle cells and the accumulation of lipid material. The earliest lesion appears to be that of the fatty streak. In this lesion, lipid accumulates within the vessel wall, either extracellularly or intracellularly, within macrophages known as foam cells. This lesion often forms quite early in the course of the disease and may even be found in the arterial system of young children [29].
The fibrous plaque, the next phase of atherogenesis, is characterized histologically by a thick fibrous luminal cap composed of smooth muscle cells and connective tissue. This plaque typically overlies a core composed of necrotic debris and lipid material (the atheroma). Continued proliferation of smooth muscle cells and accumulation of lipid material result in the luminal narrowing characteristic of the disease [30].
In some cases, although it is unclear why, the plaque may develop features of luminal ulceration and wall calcification or hemorrhage. These changes result in what is known as the complicated lesion of atherosclerosis. The nature of this lesion is far more unstable and is the source for the arterioarterial thromboembolic events as well as acute arterial thrombosis that can be observed in these patients [31].
Theories of Atherosclerosis
The extreme complexity of the atherosclerotic process has resulted in the formulation of several theories to explain its pathogenesis. These theories are based on attempts to account for one or more aspects of the disease and are therefore not mutually exclusive. In general, three theories have emerged as the most reasonable: the response to injury hypothesis, the lipid hypothesis, and the monoclonal (or mutagenic cellular transformation) hypothesis.
The Response to Injury Hypothesis
The response to injury hypothesis is based on the marked similarity of atherosclerotic lesions to those occurring after experimental injury. The hypothesis states that some form of arterial injury (via the aforementioned risk factors) results in focal disruption of the endothelium, thus allowing interaction between the blood elements and the arterial wall. This then allows interaction of leukocytes and platelets with the disrupted surface. Platelet degranulation results, as does migration of macrophages into the injured intimal layer. One substance released from the platelet—platelet-derived growth factor (PDGF)—is thought to induce the smooth muscle proliferation characteristic of the process [32, 33]. This hypothesis may explain the marked tendency for atherosclerosis to develop in regions of increased turbulence and endothelial shear stress such as that observed at bifurcations, branchings, and curvatures in arteries. Both low endothelial and high endothelial shear stress are felt to be involved in the atherosclerotic process [34–36].
The Monoclonal Hypothesis: Smooth Muscle Proliferation
The monoclonal hypothesis states that the smooth muscle proliferation characteristic of the plaque is similar to a benign neoplasm arising from a single progenitor cell from the monocyte–macrophage lineage. Evidence for this is provided by the observance of a monotypic enzyme pattern in the plaques of heterozygotic individuals, as opposed to the bimorphic pattern seen in the undiseased arterial wall [37]. The aforementioned risk factors function as theoretical mutagens.
This hypothesis considers events that cause smooth muscle proliferation as critical in atherogenesis. Actions of other growth factors may either stimulate or inhibit cell proliferation, depending on the circumstances, as well as on macrophage-derived cytokine activity, such as the finding of transforming growth factor-β receptors in human atherosclerosis [38] provides evidence for an acquired resistance to apoptosis. Resistance to apoptosis may lead to proliferation of a resistant cell subset associated with progression of stenotic lesions. This theory once again focuses on the smooth muscle proliferation but fails to account for the other features of the atherosclerotic lesion.
Lipid Hypothesis
The relatively simple lipid hypothesis states that the lipids within the atherosclerotic lesion are derived from circulating lipoproteins in the bloodstream. Support for this theory has been provided by the Association of Atherosclerosis with elevated levels of LDL [39]. This hypothesis, however, fails to account for other features of the lesion, including smooth muscle proliferation and thrombotic events.
In summary, obviously no one theory provides an adequate explanation for all the pathologic changes observed in atherogenesis. This subject remains a focus of continual investigation worldwide, and it is hoped that with improved understanding of the atherogenic process, better preventive strategies might be developed.
Vascular Hemodynamic/Atherosclerosis
Vascular hemodynamics are described in Chap. 5. Atherosclerotic occlusive disease primarily affects circulatory flow through energy losses at fixed arterial stenoses . The reasons for this arise from knowledge of the physical properties of blood as a fluid. As blood flow enters an area of stenosis, its velocity increases across the stenosis to maintain constant flow. Energy is then lost with the change in velocity at both the entry to and exit from the stenotic area. The greater the degree of stenosis, the more severe the change in velocity and thus the greater the energy loss will be. In general, flow studies have demonstrated that significant changes in flow and velocity do not occur until the degree of stenosis approaches 50%, which in turn corresponds to an area reduction of approximately 75%. This is termed critical stenosis.
Clinical Manifestations of PAD
The clinical manifestations of lower extremity atherosclerotic occlusive disease occur along a well-defined spectrum of severity, ranging from asymptomatic to intermittent claudication to the observation of trophic changes in critical limb ischemia suggesting impending limb loss.
Claudication is defined as pain (or discomfort) produced with brisk use of an extremity and relieved with rest. We have also observed that the location of the discomfort is typically experienced one joint distal to the stenotic area. For example, disease involving the superficial femoral artery manifests itself via calf claudication. Blood flow is adequate in the resting state; thus pain occurs only when the increased metabolic demand created by exercising muscle exceeds the supply available due to the degree of fixed arterial obstruction [42]. Aside from diminished distal pulses, significant physical findings are usually absent at this stage.
The differential diagnosis of intermittent claudication should include other conditions, which may be neurologic or musculoskeletal in origin. Calf pseudoclaudication can be caused by venous claudication, chronic compartment syndrome, Baker’s cyst, and nerve root compression. The tight bursting pain of compartment syndrome is generally typical, and venous claudication is relieved by leg elevation. Hip or buttock claudication should be differentiated from pain related to hip arthritis and spinal cord compression. The persistent aching pain caused by variable amounts of exercise and associated symptoms in other joints may distinguish arthritis from claudication. Patients with spinal cord compression frequently present with a history of back pain and have symptoms on standing, but require a change in position as well as rest to obtain relief. Foot claudication should be distinguished from other causes and can be related to arthritis or inflammatory processes.
Ischemic rest pain develops when the degree of circulatory impairment progresses to the point at which the blood supply is inadequate to meet the metabolic demand of the muscle even in the resting state. Frequently, the pain increases during periods of lower extremity elevation (lying in a bed) and is relieved with dependency. Physical findings at this stage typically include decreased skin temperature and delayed capillary refill, as well as Buerger’s signs (dependent rubor and pallor on elevation). Often patients can have neuropathic pain from diabetic neuropathy, and this too needs to be distinguished from ischemic rest pain with some patients having a combination of both.
Trophic changes represent the most severe manifestations of chronically impaired lower extremity circulation. In this stage of the disease, the lower extremity displays actual changes related to ischemia that are visible to the naked eye. These changes range from subtle signs such as dependent rubor, atrophy of skin and muscle, and loss of hair or nail substance to frank ulceration, cyanosis, and gangrene. The presence of trophic changes is suggestive of impending limb loss and necessitates urgent intervention for limb salvage to be possible [43].
Natural History and Staging
Several studies over the past 40 years have concluded that approximately 75% of all patients with claudication experience symptom stabilization or improvement over their lifetime without the need for any intervention [43, 44]. This clinical improvement or stabilization holds true, despite arteriographic evidence for disease progression in most of these patients. In 25% of claudicant patients, symptoms worsen, particularly during the first year, in approximately 8%, and subsequently at the rate of 2–3% per year. It has been estimated that around 5% of these patients will undergo an intervention within 5 years of their initial diagnosis. Several large studies estimate that 2–4% of these patients will require a major amputation [45, 46]. Diabetes and smoking are the most significant primary risk factors for disease progression and higher intervention and amputation rates. Dormandy and Murray [47] concluded that an ABI of 0.5 on initial diagnosis was the most significant predictor for peripheral arterial disease deterioration requiring intervention. They also observed that men were at higher risk for disease progression than women. Other studies have confirmed that the presence of peripheral arterial disease significantly increased the risk of myocardial infarction, stroke, ischemia of splanchnic organs, and the risk of cardiovascular death. Criqui et al. [48] noted a relative risk of 3.3 for total mortality and a relative risk of 5.8 for coronary artery disease mortality in men with peripheral arterial disease over a 10-year study. They also noted a twofold higher relative risk of a total, coronary, and cardiovascular mortality in symptomatic versus asymptomatic peripheral artery disease patients. It has been estimated that the average life expectancy of patients with intermittent claudication was decreased by about 10 years [44]. A review of over 20 studies by TASC places the 5-, 10-, and 15-year mortality rate for patients with intermittent claudication at 30%, 50%, and 70%, respectively [21].
Determination of the ABI has proven to be a powerful clinical tool. An ABI of <0.5 has been associated with more severe coronary artery disease and increased mortality [49]. It has been demonstrated that patients with an ABI of <0.3 had a significantly lower survival rate than those with a range of 0.31–0.9 [49].
Sheikh et al. reported on the usefulness of post-exercise ABI to predict all-cause mortality. They conducted an observational study of consecutive patients referred for ABI measurement before and after fixed-grade treadmill or symptom-limited exercise component to a noninvasive vascular laboratory during a 10-year period. The patients were classified into two groups: Group 1 included patients with an ABI of ≥0.85 before and after exercise, and Group 2 included patients with a normal ABI at rest, but <0.85 after exercise. A total of 6292 patients were analyzed. The 10-year mortality rate for Groups 1 and 2 was 33% and 41%, respectively. An abnormal post-exercise ABI result independently predicted mortality (a hazard ratio of 1.3, p = 0.008). Additional independent predictors of mortality were age, male gender, hypertension, and diabetes. After the exclusion of patients with a history of cardiovascular events, the predictive value of an abnormal post-exercise ABI remained statistically significant (a hazard ratio of 1.67, p < 0.0001). They concluded that the post-exercise ABI was a powerful independent predictor of all-cause mortality and provides additional risk stratification beyond resting ABI [50].
Aboyans et al. assessed the general prognosis of patients with PAD according to the disease location in 400 patients. Aortoiliac disease (proximal PAD) and infrailiac disease (distal PAD) were noted in 211 (53%) and 344 (86%) cases, respectively. Male sex and smoking were prevalent in proximal PAD, whereas an older age, hypertension, diabetes, and renal failure were more prevalent in distal PAD (p < 0.05). At a mean follow-up of 32 months, the event-free survival curves differed according to the PAD localization (p < 0.03). Adjusted for sex, age, cardiovascular disease history, cardiovascular disease risk factors, critical leg ischemia status, and treatment, proximal PAD was significantly associated with a worse prognosis (primary outcome hazard 3.28; death hazard ratio 3.18; p < 0.002) versus distal PAD. This was the first study to report a poorer general prognosis for patients with proximal aortoiliac disease compared to those with more distal disease [51].
After the initial diagnosis of chronic lower extremity ischemia is made, it is important to stage the severity of the disease process accurately. This is crucial because it is the stage of the disease and the natural history of each stage that ultimately determines which therapy is most appropriate.
The most common staging system used by vascular surgeons today is that devised by the Society of Vascular Surgery and the International Society of Cardiovascular Surgery SVS/ISCVS). Rutherford et al. [52] defined peripheral arterial disease into Grade and Categories defined as asymptomatic (Grade 0 and Category 0); claudication as mild, moderate, and severe (Grade I and Categories 1–3); ischemic rest pain (Grade II and Category 4); and tissue loss (Grade III and Categories 5 and 6). See Table 21.1 for details. Critical limb ischemia encompasses Grades II and III and Categories 4–6. Rutherford’s classification is presently the recommended standard when describing clinical assessment and progress [21]. The other classification that is used by our medical colleagues is the Fontaine classification. In this classification, the stages of the disease are categorized into Stages I–IV (Stage I corresponds to Grade 0 and Category 0 in Rutherford’s classification, and Stage IV corresponds to Grade III and Categories 5 and 6 in Rutherford’s classification).
Table 21.1
Classification of peripheral arterial disease (PAD): Rutherford categories
Grade | Category | Clinical description |
---|---|---|
0 | 0 | Asymptomatic |
I | 1 | Mild claudication |
I | 2 | Moderate claudication |
I | 3 | Severe claudication |
II | 4 | Ischemic rest pain |
III | 5 | Minor tissue loss |
III | 6 | Major tissue loss |
Stage 0 (Asymptomatic )
In stage 0, the patient is symptom-free. The natural history of Stage 0 disease is such that few patients will progress to limb loss over a period of 5 years. For this reason, the only treatment recommended for this stage involves risk factor modification such as cessation of smoking, reduction of serum lipids, and improved control of diabetes mellitus. The use of statin therapy has been demonstrated to decrease major adverse cardiovascular events as well as all-cause mortality in patients with asymptomatic PAD [53]. Close observation is also important since any progression to a more advanced stage necessitates a change in the treatment strategy.
Stage I (Claudication)
Stage I has been further subdivided into two groups based on the severity of claudication considered mild and moderate to severe. Stage IA disease is defined as claudication that occurs after walking more than 200 m pain-free. Stage IB is defined as claudication that occurs after walking less than 200 m. The natural history of patients with claudication is such that very few patients will progress to limb loss. The actual percentages in the literature vary somewhat, but the 5-year limb loss rate seems to average approximately 5% [42, 54].
Because of the relatively benign natural history of Stage I disease (Rutherford Grade I, Categories 1–3), the cornerstone of therapy remains aggressive medical management, particularly for patients with Stage IA disease. Stage IB (Rutherford Categories 2 and 3) disease may be considered a relative indication for invasive intervention if medical therapy fails or if the degree of disability is intolerable for the patient. Once again, progression to a more advanced stage necessitates a change in the treatment plan.
Stage II (Ischemic Rest Pain )
Stage II is generally viewed as the earliest phase of limb-threatening ischemia. The natural history at this stage carries a far worse prognosis. Patients with Stage II disease are far more likely to have progression of their disease process and ultimately to suffer limb loss [55]. For this reason, invasive intervention is indicated at this stage.
Stage III (Trophic Changes)
The presence of trophic changes indicates the most severe underlying circulatory impairment, as evidenced by actual tissue loss or gangrenous changes. Untreated, most patients will progress limb loss. For this reason, an aggressive interventional approach is indicated to limit additional tissue loss and allow limb salvage [55].
It should be noted that progression of patients with PAD from asymptomatic to claudication to rest pain to gangrene or limb loss is generally unpredictable. It has been shown that more than one-half of patients having a below-knee major amputation for ischemic disease had no prior symptoms of leg ischemia as recent as 6 months previously [56]. The incidence of major amputations, according to large population studies, varies from 120 to 500 per million per year, and the ratio of below-knee to above-knee amputation in large surveys is around 1:1. It has also been reported that approximately 60% of below-knee amputations heal by primary intention, while 15% heal after secondary procedures, and 15% will need above-knee amputation, while 10% die in the perioperative period.
Diagnostic Investigation
History and Physical Examination
As with any condition, evaluation begins with a comprehensive history and physical examination prior to any objective testing.
The initial history should be directed toward the occurrence of pain in the lower extremities. As defined previously, claudication typically manifests itself as pain that occurs with brisk use of an extremity (via walking) and is relieved consistently by several minutes of rest. The location and character of the pain should be reproducible, as should be the claudication distance. Rest pain should also be inquired about and, once again, may be most prominent at night when the legs are elevated. Any history of ulceration, loss of hair, nail substance, or skin/muscle atrophy should be sought. The presence of impotence, frequently present in aortoiliac disease, should be carefully documented.
Because of the systemic nature of atherosclerosis, the possibility of multi-vessel involvement exists and should be considered in all patients. A careful history should be elicited regarding symptoms of coronary artery disease (angina or myocardial infarction), cerebrovascular disease (transient ischemic attacks or prior strokes), or previous vascular procedures.
Physical examination begins with a comprehensive head-to-toe assessment. When attention is directed toward the lower extremities, thorough visual inspection should reveal evidence of any trophic changes. It is important to look between the toes as well, to avoid missing subtle areas of skin loss. Skin temperature and capillary refill should be assessed and may be diminished with more advanced disease. A leg lift test to assess for pallor with elevation should also be implemented. Auscultating for abdominal and femoral bruits should be carried out, and pulses should be palpated at all levels and their strength documented as follows: +2 (normal), +1 (diminished), and 0 (no palpable pulse). Others classify pulses as 3+, 2+, 1+, and 0.
If the pulse is non-palpable, a handheld Doppler probe should be used to determine the presence or absence of flow in the vessel, and its flow characteristics documented (monophasic, biphasic, triphasic).
Noninvasive Tests
A variety of noninvasive tests are available for assessment of the lower extremity vascular system. These are discussed in detail in subsequent chapters.
Perhaps the most useful bedside noninvasive test available to the clinician is the ABI. This test may be performed with only a sphygmomanometer and a Doppler probe. To perform the test, Doppler systolic pressure measurements are taken at the level of the ankle (use the higher of posterior tibial or dorsalis pedis artery pressure) and compared with those in the brachial artery (both upper extremity pressures should be measured and the highest used) as follows:


A ratio of less than or equal to 0.9 is abnormal. Generally, patients with claudication have indices of 0.5–0.8, whereas those with rest pain have indices of 0.5 or less. When trophic changes are present, the ABI is frequently less than 0.3 [57].
In general, noninvasive testing should precede the ordering of any more invasive evaluations.
Overview of Noninvasive Testing in PAD
Noninvasive vascular tests help the physician to evaluate the presence or absence of significant arterial occlusive disease, severity of disease, location of disease, and in the presence of multi-segmental disease, which arterial segment is mostly affected.
General indications for obtaining noninvasive assessment of the peripheral arterial system include absence of normal pulses, suboptimal examiner reliability or experience, a clinical history or examination potentially consistent with peripheral arterial occlusive disease, and a planned vascular procedure.
Several noninvasive diagnostic techniques have been used in the diagnosis of peripheral arterial disease: continuous-wave (CW) Doppler ultrasound, pulsed Doppler ultrasound, B-mode ultrasound, various plethysmographic techniques, including pulse volume recording, thermography, electromagnetic flowmeter, radionuclide angiography, and the use of radioisotopes. The most commonly used methods for diagnosis of peripheral arterial disease of the lower extremity at present are segmental Doppler pressures (with or without Doppler wave analysis) using CW Doppler, pulse volume recording, and duplex ultrasonography [58–62]. These three commonly used methods will be described in detail in the next chapters.
Doppler Ultrasound
The use of ultrasound in the range of 1–10 MHz for medical diagnosis has become widespread since its introduction by Satomura in 1959 [63]. Current instruments either use the Doppler effect to detect flow velocity or rely on tissue reflectance of transmitted sound waves (B-mode ultrasound) to produce acoustic images of blood vessels or a combination of both Doppler and B-mode imaging (duplex ultrasound).
Although Satomura [63] was credited for developing the first Doppler flow detector in 1959, its clinical application was pioneered by Strandness et al. in 1966 [64]. Since then, the instrumentation has been further improved and refined. The principle of this device depends on the observation made by the Austrian physicist, Christian Doppler (1803–1853), who demonstrated that the frequency of light or sound emitted by a source moving toward the observer is higher (shorter wavelength) than the transmitted frequency and lower (longer wave length) when the source is moving away from the observer, e.g., the pitch of the train whistle sounds higher as the train approaches and lower as the train moves away. The Doppler effect can be stated as


where
average flow velocity; C = velocity of sound in tissue; Δf = Doppler frequency shift; fo = transmitting frequency of ultrasound beam; and θ = angle of the incident sound beam to the blood vessel being examined. Since transmitting frequency, angle of incidence, and sound velocity in tissue can be kept constant, frequency shift (Δf) becomes proportional to the velocity of blood flow.

There are two types of Doppler ultrasound detectors: CW Doppler ultrasound and pulsed Doppler ultrasound. Both instruments work on the principle of the Doppler effect. This is described in detail in Chap. 4.
Instrumentation
Many types of Doppler instruments are commercially available, varying from small, portable, pocket-sized models to more sophisticated instruments. The CW detectors emit an ultrasound beam without interruption. Such devices are not range specific, i.e., they will detect blood flow at any depth within the range of the instrument up to several centimeters, depending upon the frequency of the instrumentation. The pulsed Doppler detectors transmit intermittent bursts of ultrasound that can be sampled for retained signals at various times after transmission, permitting range resolution of detected flow at a given point from the transducer. As mentioned earlier, CW Doppler units can be directional or nondirectional. Figure 21.5 shows a commonly used pocket Doppler unit (Dopplex D900, Huntleigh Diagnostics, Eatontown, NJ); Figure 21.6 shows a commonly used directional Doppler unit (FALCON/Pro, Viasonix Ltd., Raanana, Israel).



Fig. 21.5
Handheld Doppler ultrasound unit

Fig. 21.6
FALCON/Pro, Viasonix Ltd., Advanced Medical Technologies, Raanana, Israel, a commonly used directional Doppler unit. Photo courtesy of Viasonix Ltd
Duplex Ultrasound and Color Flow Imaging
When pulsed ultrasound Dopplers and B-mode scanners are combined, the resulting duplex scan is capable of not only imaging the vessel under study but also of detecting blood flow velocity at multiple points within its lumen. Presently, color duplex ultrasonography is the most common instrumentation used in modern vascular laboratories.
Color flow imaging provides the duplex information described above, i.e., combined real-time B-mode imaging (grayscale evaluation) and Doppler spectral analysis. In addition, it evaluates the Doppler flow information for its phase (direction toward or away from the transducer, and color is assigned on this basis) and its frequency content (which determines the hue or shade of the assigned color).
Plethysmography
Principle
The principle of plethysmography is based on graphic recordings of a change in dimension of a portion of the body in response to each heartbeat or in response to temporary obstruction of the venous system (venous occlusion plethysmography) [65]. Most plethysmographs directly or indirectly record the change in column of a digit, limb, or other part of the body. An exception to this is the photoplethysmograph (PPG) that records the change in reflection of light from the change in number of red blood cells in the cutaneous microcirculation.
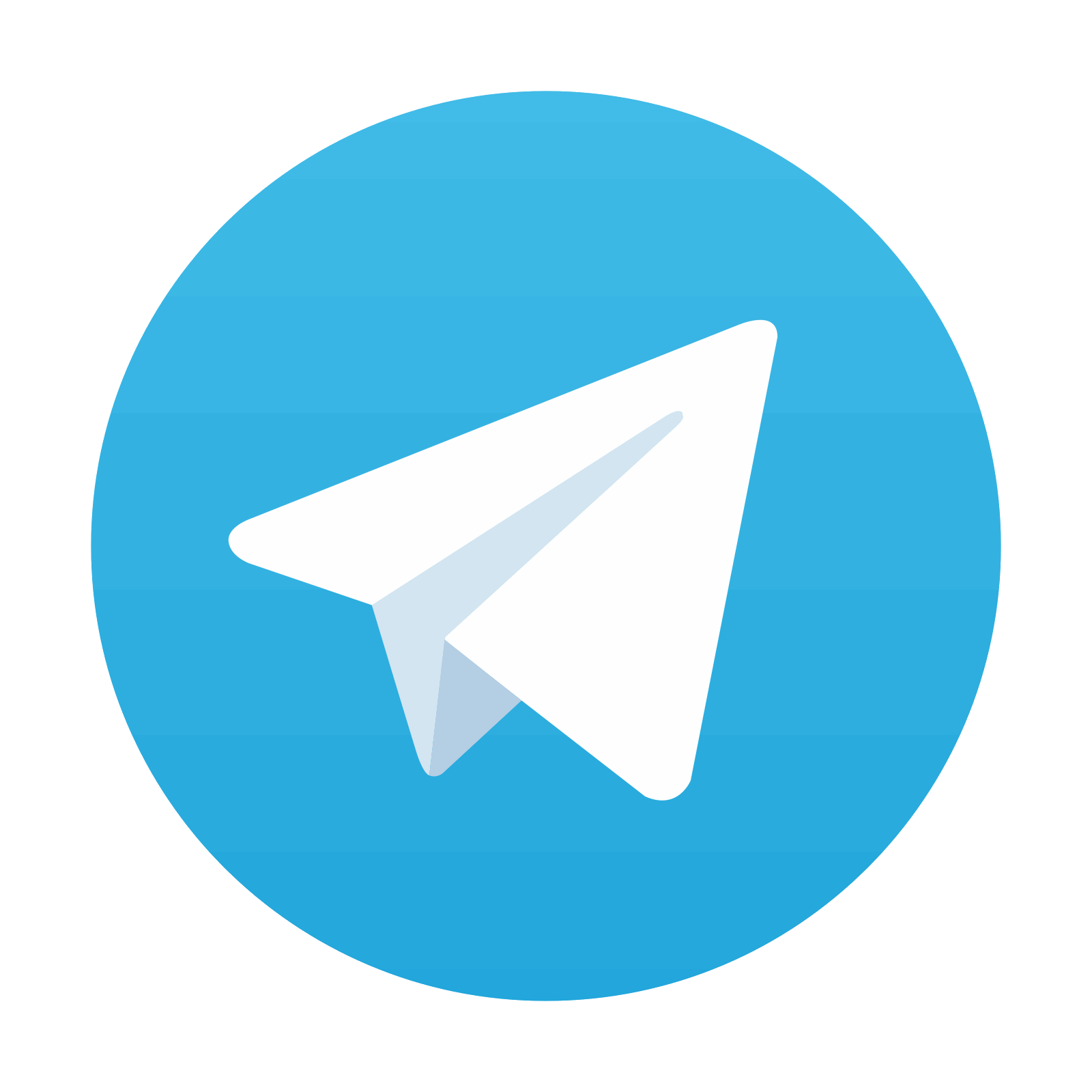
Stay updated, free articles. Join our Telegram channel

Full access? Get Clinical Tree
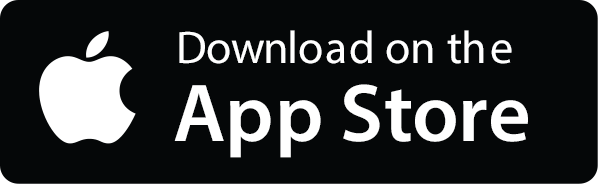
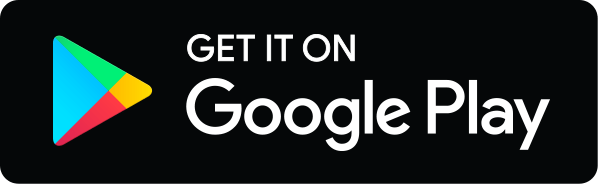