Fig. 6.1
An illustration showing the aortic arch and its branches: (1) brachiocephalic trunk, (2) right subclavian artery, (3) right vertebral artery, (4) right common carotid artery, (5) right external carotid artery, (6) right internal carotid artery, (7) aortic arch, (8) left subclavian artery, (9) left vertebral artery, (10) left common carotid artery, (11) left external carotid artery, (12) left internal carotid artery, (13) the basilar artery
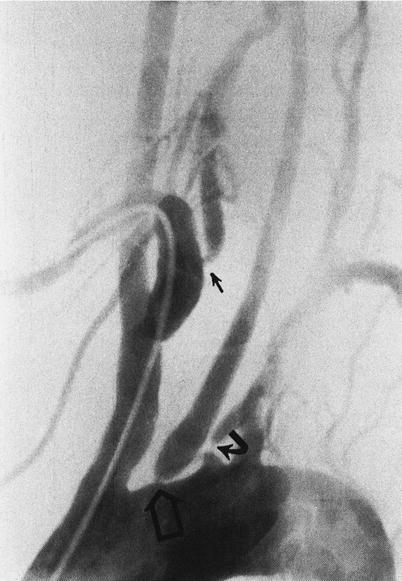
Fig. 6.2
Arch aortogram showing left vertebral artery originating from the arch of the aorta with tight stenosis at its origin (curved arrow) and the right vertebral artery (coming off the right subclavian artery) with a tight stenosis at its origin (straight arrow)
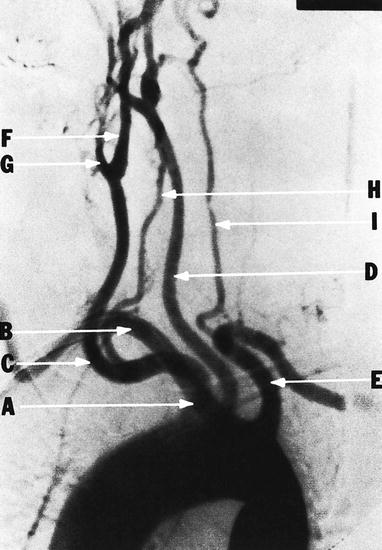
Fig. 6.3
Abnormal origin of the vertebral artery (H) from the right common carotid artery (C) in a patient with retroesophageal right subclavian artery (B). (A) innominate artery; (B) right subclavian artery; (C) right common carotid artery; (D) left common carotid artery; (E) left subclavian artery; (F) right internal carotid artery; (G) right external carotid artery; (H) right vertebral artery; (I) left vertebral artery (Courtesy of Springer-Verlag. Berguer and Kieffer [20])
The common carotid arteries on each side travel in the carotid sheath up to the neck before branching into internal and external carotid arteries just below the level of the mandible. The external carotid artery supplies the face. Important branches of the external carotid artery include the superior thyroid, which can actually arise from the common carotid artery and is important in that it accompanies the external branch of the superior laryngeal nerve and the ascending pharyngeal, lingual, and occipital arteries that have a close association with the hypoglossal nerve (Fig. 6.4). No branches of the internal carotid artery are located in the neck.
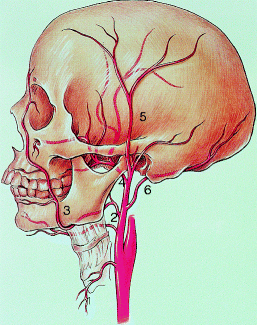
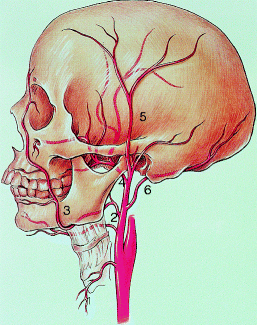
Fig. 6.4
Main branches of the external carotid artery: (1) superior thyroid artery, (2) lingual artery, (3) facial artery, (4) internal maxillary artery, (5) superficial temporal artery, and (6) occipital artery (Courtesy of Springer-Verlag. Berguer and Kieffer [20])
The carotid sinus, a baroreceptor, is located in the crotch of the bifurcation of the internal and external carotid arteries. It is innervated by the nerve of Hering, which branches from the glossopharyngeal nerve. The carotid body is a very small structure that also lies in the crotch of the bifurcation and functions as a chemoreceptor, responding to low oxygen or high carbon dioxide levels in the blood. It is also innervated by the glossopharyngeal nerve via the nerve of Hering.
The corticotympanic artery and the artery to the pterygoid canal are branches of the internal carotid artery in its petrous portion. The cavernous, hypophyseal, semilunar, anterior meningeal, and ophthalmic arteries are branches of the cavernous portion of the internal carotid artery. The ophthalmic artery is clinically important since it communicates with the external carotid system, which is the basis of the periorbital Doppler study. The remaining branches of the internal carotid artery arise from the cerebral portion, i.e., the anterior and middle cerebral, posterior communicating, and choroidal branches (Fig. 6.5).
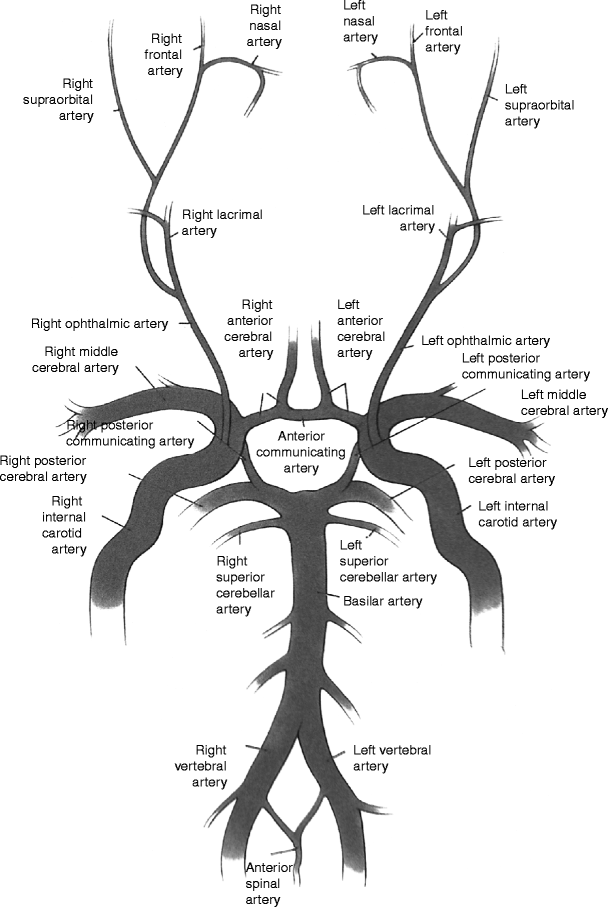
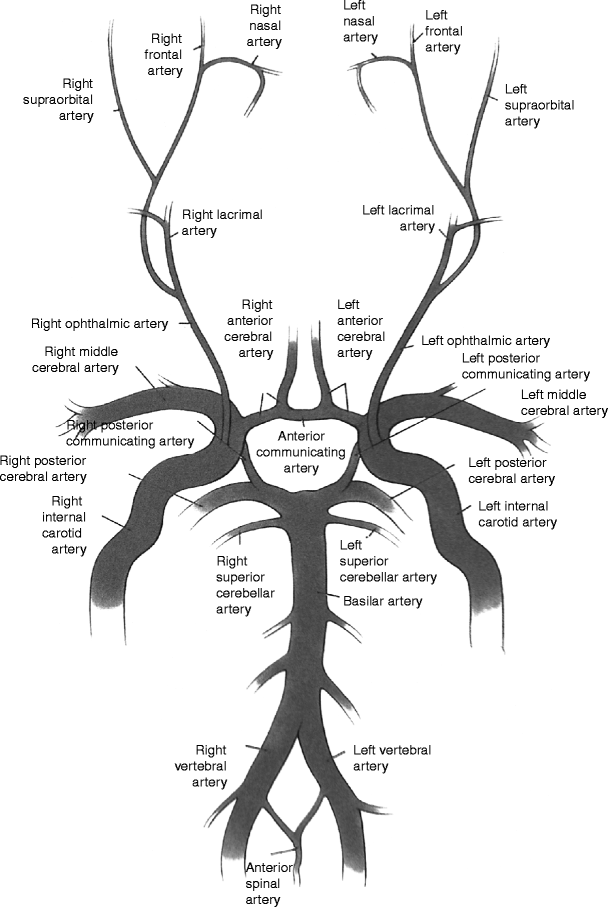
Fig. 6.5
Major branches of the internal carotid artery (i.e., middle cerebral, anterior cerebral, posterior communicating, and ophthalmic arteries) and vertebrobasilar arteries
The vertebral artery leaves the subclavian artery and pushes upward through the foramina of the transverse processes of the cervical vertebrae into the cranium through the foramen magnum (Fig. 6.6). The neck spinal branches enter the vertebral canal through the intervertebral foramen, and muscular branches are given off to the deep muscles of the neck. These latter branches anastomose with branches of the external carotid artery.
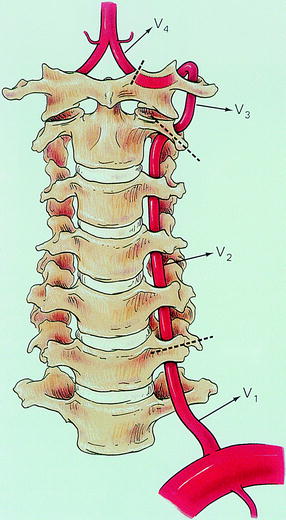
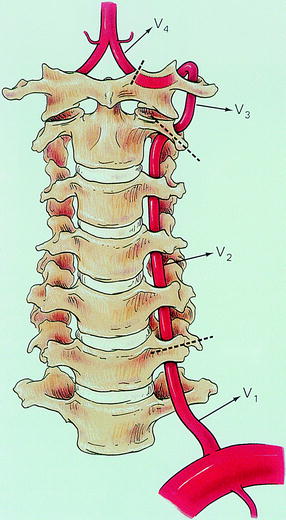
Fig. 6.6
Relation of the vertebral artery and cervical spine. The dotted line indicates the level at which the vertebral artery becomes intradural. As noted, the vertebral artery is divided into four portions (V 1, V 2, V 3, and V 4). V1 is the segment between its origin and the level where it enters the transverse process of the sixth cervical vertebra. V2 is the segment of the artery through the foramina of the transverse processes of the cervical spines until the dotted line. V3 is the segment between C2 and C1. V4 is the segment prior to joining the other vertebral artery to form the basilar artery
Intracranially, the vertebral arteries give off the posterior inferior cerebellar and spinal arteries before they are united at the pontomedullary junction to form the basilar artery. The basilar artery terminates as the posterior cerebral artery after giving off the anterior inferior cerebellar, superior cerebellar, pontine, and internal auditory arteries.
Blood flows through each internal carotid artery at about 350 ml/min, accounting for approximately 85% of the blood supply to the brain, and the vertebral arteries account for about 15% of the total blood supply to the brain [22]. Therefore, the carotid arteries, both from the standpoint of accessibility and functioning, become the system of importance for noninvasive testing.
Morphologic Variations of the Internal Carotid Artery
Tortuosity of the internal carotid artery (or loop) is generally defined as an S- or C-shaped elongation or curving in the course of the artery (Fig. 6.7a). Coiling is a term used to describe an exaggerated, redundant S-shaped curve, or a complete circle, in the longitudinal axis of the artery (Fig. 6.7b). Tortuosity and coiling are thought to be congenital developmental abnormalities that may become exaggerated with aging. They usually do not produce clinical symptoms.
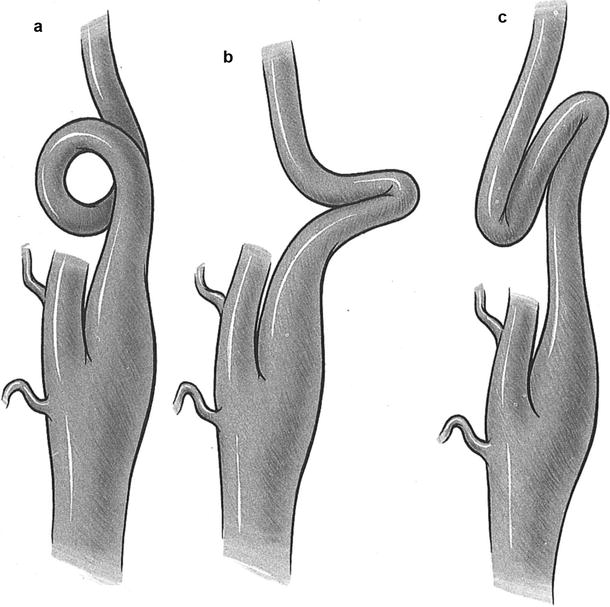
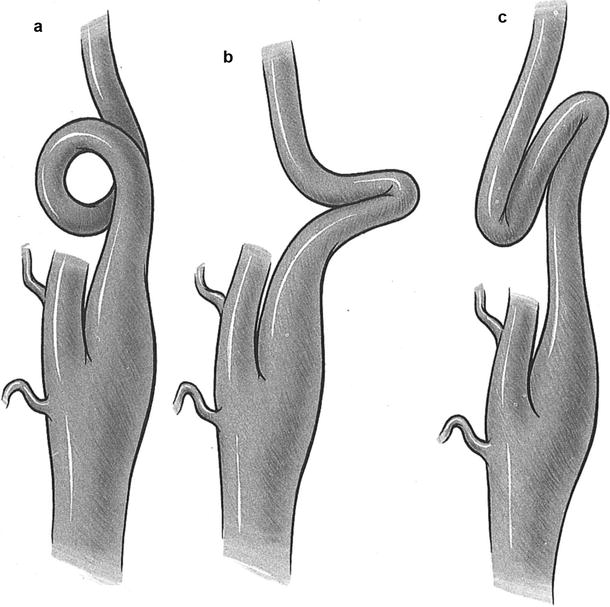
Fig. 6.7
Three types of redundancy of the internal carotid artery. (a) Coil. (b) Kink. (c) Loop (Courtesy of Springer-Verlag. Berguer and Kieffer [20])
Kinking is a sharp angulation with stenosis of segments of the internal carotid artery (Fig. 6.7c) and appears to be somewhat different than tortuosity and coiling. Kinking is less frequently bilateral and usually affects a few centimeters or more above the carotid bifurcation.
Poststenotic dilatation may be present. Frequently, an atherosclerotic plaque on the concave side of the kink further narrows the lumen.
Unlike tortuosity and coiling, kinking is thought to be an acquired abnormality, occurring mostly in older people in whom arteriosclerotic disease and hypertension are important factors. Changes in head and neck positions may produce cerebral ischemia in patients with carotid kinking, whereas tortuosity and coiling are usually asymptomatic.
Cerebral Collateral Pathways
Single stenotic lesions may or may not produce carotid territory symptoms (hemispheric) or vertebrobasilar territory symptoms (nonhemispheric). The collateral system comes into play when resistance in the stenotic major artery is greater than the resistance in the smaller circulative collateral channel. As stenosis becomes more severe and the collateral channel arteries dilate in response to increased flow, the collateral channels will increase flow up to the point of capacity. It can then be seen that the more proximal the obstruction, the greater the potential for collateral pathways to exist. Proximal occlusion of the arteries arising from the aortic arch is, therefore, rarely associated with the stroke syndrome because of this collateral flow. Branches of each subclavian artery may anastomose with collaterals of the opposite subclavian artery and the branches of the external carotid system.
The subclavian steal syndrome [23] occurs with proximal subclavian occlusion and retrograde flow down the vertebral artery (Figs. 6.8 and 6.9a). In innominate artery obstruction, the retrograde flow may be down to the carotid artery (Fig. 6.9b). Therefore, vascular surgeons may use the mechanism of collateral flow in a constructive manner; a patient who has both a carotid stenosis and subclavian steal syndrome may be completely relieved of steal symptoms by CEA.
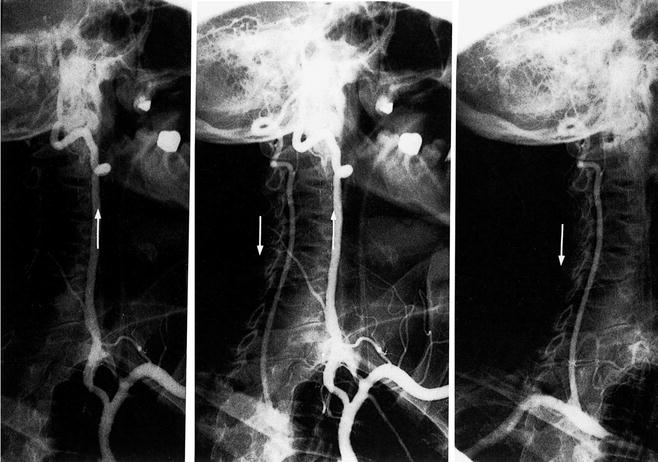
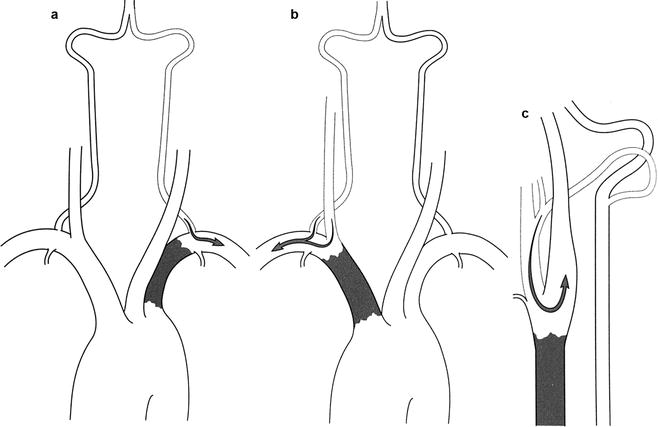
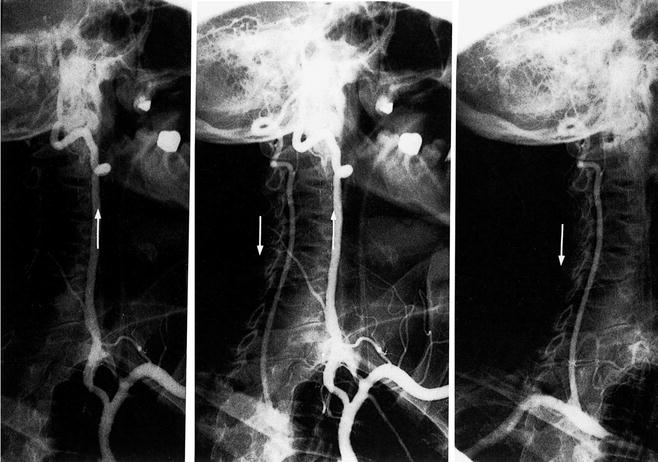
Fig. 6.8
Right subclavian steal secondary to occlusion of the first segment of this artery. The left vertebral artery is filling from the left subclavian artery as seen on the left side of this figure (arrow). In the middle of this figure, flow is seen retrograde in the right vertebral artery (arrow), and as seen on the right side of this figure, the right subclavian artery is filling retrograde via the left vertebral artery (arrow) (Courtesy of Springer-Verlag. Berguer and Kieffer [20])
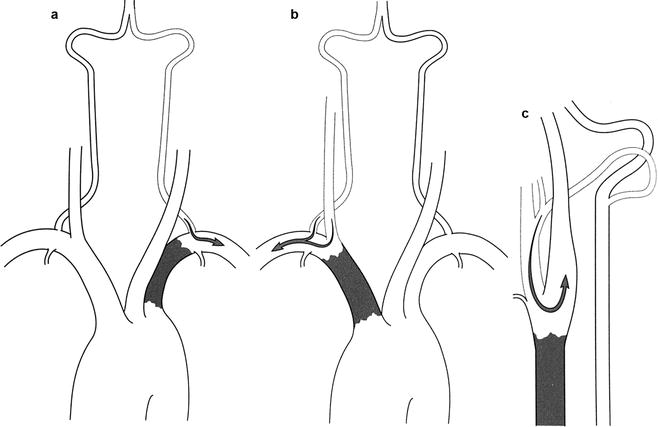
Fig. 6.9
Three patterns of flow reversal. (a) Subclavian steal distal to an occluded left subclavian artery. (b) Reversal of flow in the carotid and vertebral arteries distal to an occluded innominate artery (innominate steal). (c) Reversal of flow in the occipital artery and proximal external carotid distal to an occluded common carotid artery (carotid steal) (Courtesy of Springer-Verlag. Berguer and Kieffer [20])
An interesting collateral pathway is the retrograde flow that may occur in the external carotid system by proximal occlusion of the common carotid artery (Fig. 6.9c). Collateral flow to the internal carotid artery occurs by way of the ophthalmic artery or the circle of Willis. Patients with a proximal internal carotid occlusion might have blood flow up the external carotid artery and retrograde through the supraorbital and frontal arteries into the ophthalmic artery and, finally, antegrade in the distal internal carotid artery and the circle of Willis. This important pathway is the basis of the supraorbital Doppler cerebrovascular examination. Patients with proximal internal carotid occlusion and external carotid stenosis may be relieved of symptoms with correction of the external carotid occlusion, which alleviates symptoms of hypoperfusion [24]. Finally, emboli may course via the external carotid artery to the eye, producing amaurosis fugax in patients with a functional collateral pathway [25].
The major collateral pathway, of course, is the circle of Willis (Figs. 6.10 and 6.11). This unique circle provides the major pathway between the internal carotid, the external carotid, and the vertebrobasilar systems. The circle of Willis is anatomically complete in only one-third of patients, but it is physiologically adequate in around two-thirds of patients (Fig. 6.12). Collateral arteries between the occipital branches of the external carotid arteries may bypass stenosis of the origin of both vertebral arteries and the muscular branches of the vertebral arteries (Figs. 6.13, 6.14, and 6.15), or between the cervical branches of the thyrocervical trunk and vertebral arteries. Figures 6.16, 6.17, and 6.18 summarize the important collateral pathways in patients with an occluded internal carotid artery.
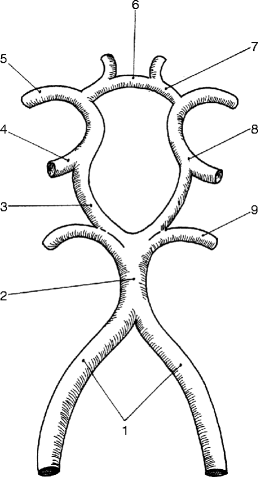
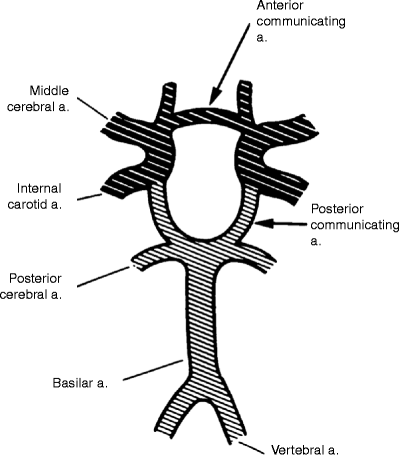
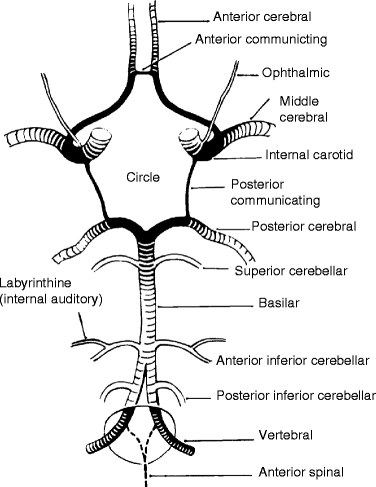
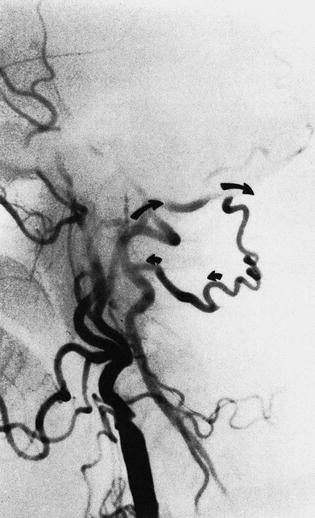
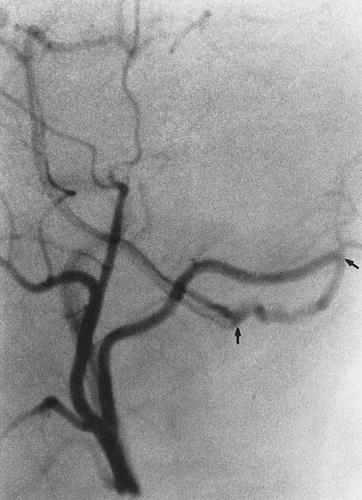
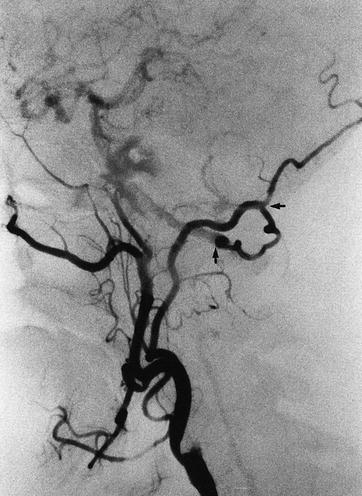
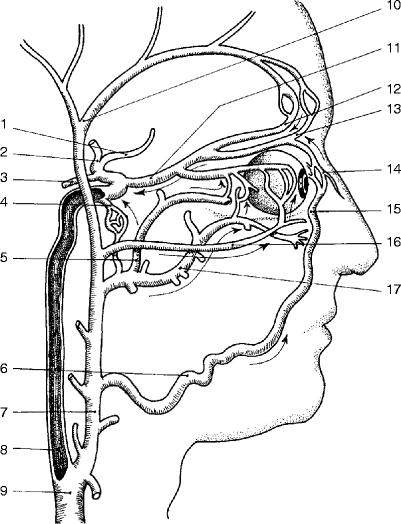
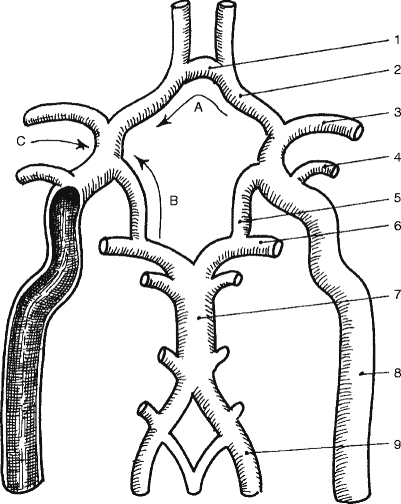
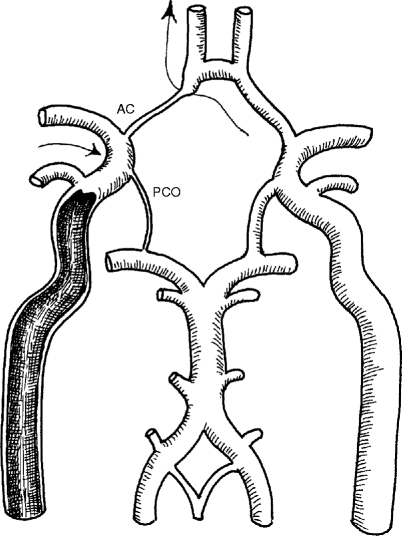
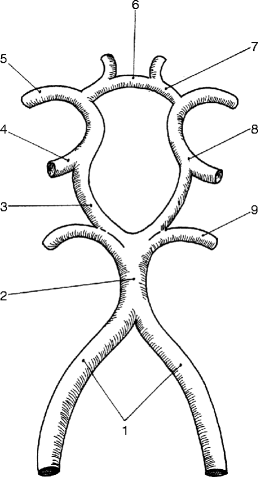
Fig. 6.10
The circle of Willis: (1) right and left vertebral arteries, (2) basilar artery, (3) right posterior communicating artery, (4) right internal carotid artery, (5) right middle cerebral artery, (6) anterior communicating artery, (7) left anterior cerebral artery, (8) left internal carotid artery, (9) left posterior cerebral artery
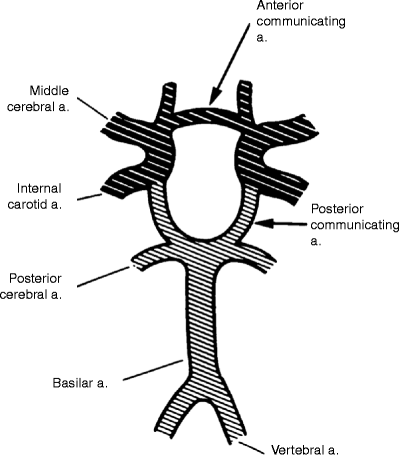
Fig. 6.11
Circle of Willis showing the anterior cerebral circulation (shaded black) and the posterior circulation (shaded gray). a artery
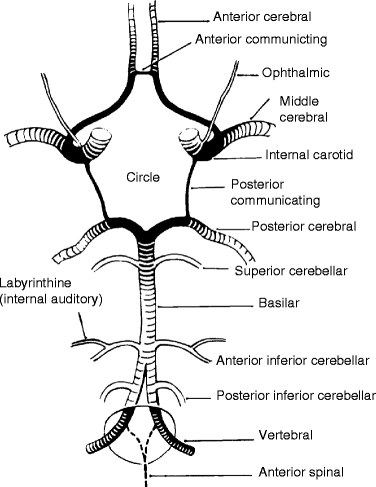
Fig. 6.12
The circle of Willis demonstrating rudimentary posterior communicating branches
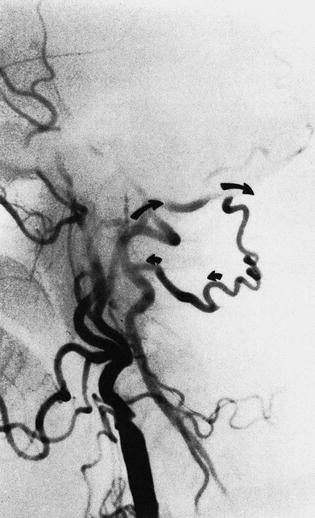
Fig. 6.13
Occipital connection of the vertebral artery. In this patient with an occluded internal carotid artery, the collaterals (arrow) from the occipital artery fill the vertebral artery anterogradely toward the basilar artery and retrogradely toward the base of the neck, where the vertebral artery is occluded
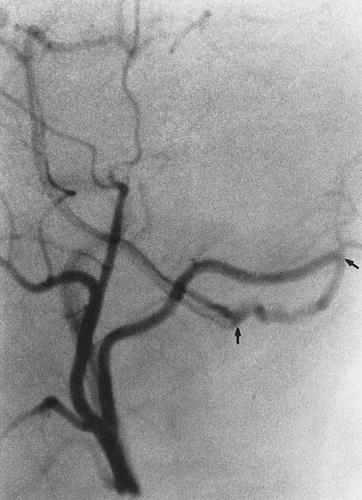
Fig. 6.14
Occipital collateral may enter the vertebral artery at the level of C1, (arrow) as seen in this selective external carotid injection
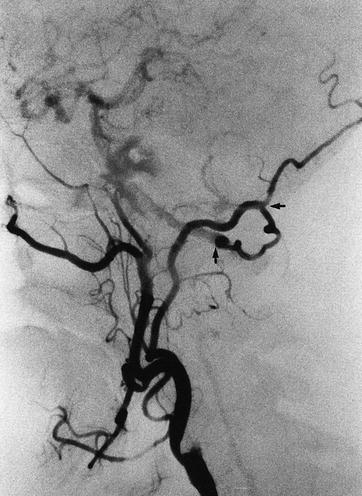
Fig. 6.15
The importance of the occipital collateral is seen in this patient with an occluded internal carotid (arrows)
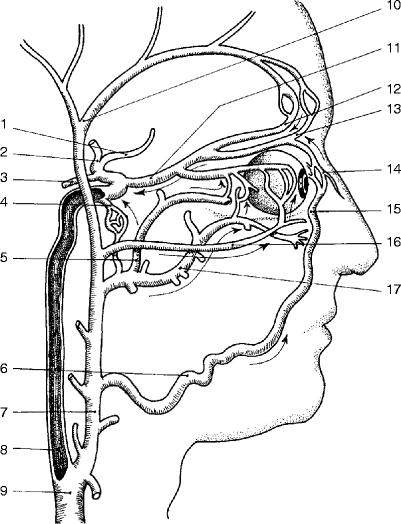
Fig. 6.16
A patient with an occluded internal carotid artery with a reversal of flow through the ophthalmic artery via periorbital branches of the external carotid artery. (1) Anterior cerebral artery, (2) middle cerebral artery, (3) posterior communicating artery, (4) caroticotympanic branch of the internal carotid artery, (5) middle meningeal artery, (6) fascial artery, (7) external carotid artery, (8) occluded internal carotid artery, (9) common carotid artery, (10) superficial temporal artery, (11) ophthalmic artery, (12) supraorbital artery, (13) supratrochlear artery, (14) dorsal nasal artery, (15) angular artery, (16) transverse fascial artery, (17) internal maxillary artery
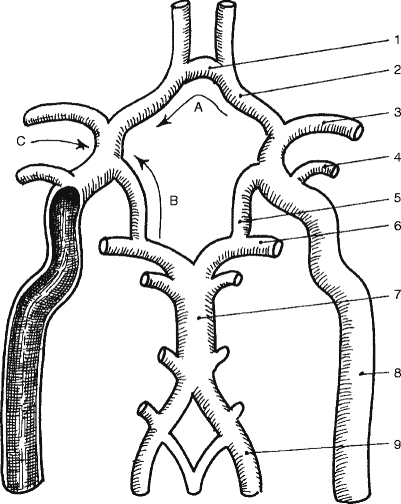
Fig. 6.17
This illustration is a patient with an internal carotid artery occlusion. The flow to the corresponding cerebral hemisphere is maintained by flow from the opposite internal carotid artery (A), the vertebral basilar system (B), and the ophthalmic artery via the periorbital branches of the external carotid artery of the same side (C). (1) Anterior communicating artery, (2) anterior cerebral artery, (3) middle cerebral artery, (4) ophthalmic artery, (5) posterior communicating artery, (6) posterior cerebral artery, (7) basilar artery, (8) internal carotid artery, (9) vertebral artery
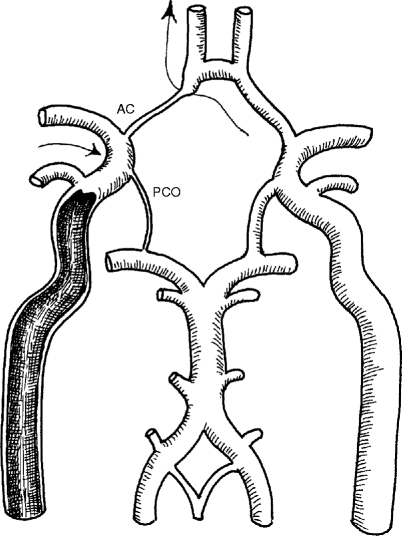
Fig. 6.18
A patient with an internal carotid artery occlusion where the collateral flow may be reduced by the incomplete circle of Willis (rudimentary right posterior communicating artery [PCO] and anterior cerebral artery [AC])
Pathology
Numerous theories exist as to the mechanism by which atherosclerosis develops in the carotid arteries, but whether you prescribe to the mechanical, sheer stress, chemical injury, or infectious theory [26], the basic lesion is essentially the same. Atherosclerosis accounts for approximately 90% of extracranial cerebrovascular disease, with the remaining 10% being attributed to such disease processes as fibromuscular dysplasia, traumatic or spontaneous dissection, aneurysms, and arteritis, including Takayasu’s arteritis.
The pathology of carotid and vertebral artery atherosclerosis is similar to the process of atherosclerosis affecting coronaries and other arteries. The early stage of the lesion is initiated by intimal accumulation of lipoprotein particles which will undergo oxidative modification and elaborate cytokines that cause expression of adhesion molecules and chemoattractants; this will facilitate the uptake and migration of monocytes into the arterial wall. These monocytes become lipid-laden macrophages (foam cells) as a consequence of accumulation of modified lipoproteins and subsequently release additional cytokines, matrix metalloproteinases, and oxidants. This is followed by smooth muscle cell migration from the media to the intima. These will proliferate and elaborate extracellular matrix as extracellular lipid accumulates in a central core surrounded by a layer of connective tissue, which is called the fibrous cap, which in many advanced plaques becomes calcified. The atherosclerotic lesion initially grows in an outward direction; however, once advanced, it will grow inward and encroach on the lumen, causing stenosis.
These plaques occur preferentially at areas of vessel bifurcations, and the process is similar to that seen with coronary artery disease. It can begin in the bulbous portion of the internal carotid artery on its posterior lateral wall. The atherosclerosis appears as a fatty strip subintimally with a collection of fat cells that progresses to a fibrous plaque in the subendothelial layer, causing gradually decreasing flow. These plaques can enlarge in several ways. They may just continue to slowly enlarge from an accumulation of cholesterol and fibroblasts, leading to a central necrosis and rupture of the intimal lining of the vessel. This will lead to discharge of atheromatous debris into the lumen of the vessel, which can embolize. The exposed necrotic core of the lesion can then become a nidus for platelet deposition and further embolization to the brain. Progressive accumulation of the arteriosclerotic process, often with thrombotic debris, may result in stenosis or total occlusion in the carotid artery, with subsequent thrombosis of the internal carotid artery distal to the lesion (Fig. 6.19). Another mechanism by which there may be sudden plaque enlargement is intraplaque hemorrhage [27], which may produce acute narrowing of the lumen. If the intima overlying the site of the plaque hemorrhage ulcerates, the necrotic contents of the atheromas escape into the lumen and cause cerebral embolization with transient ischemic attacks (TIA) or cerebral infarcts. Nonulcerated lesions may alter flow and produce mural thrombi, which may fragment, causing embolization (Fig. 6.20). The friability of these lesions is often not appreciated until seen by the vascular surgeon (Fig. 6.21).
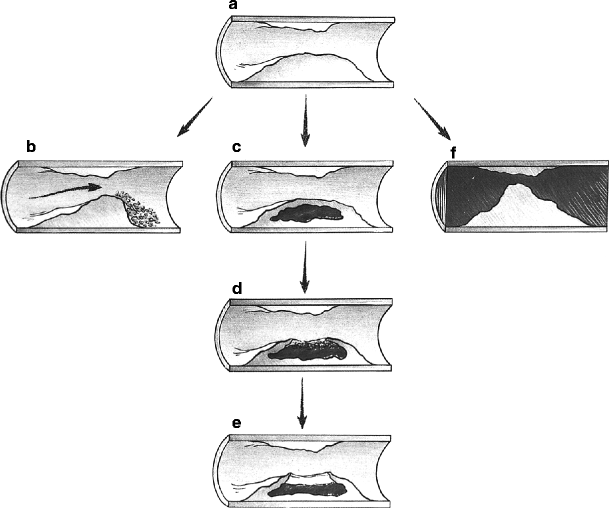
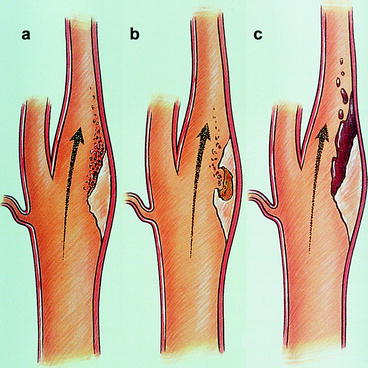
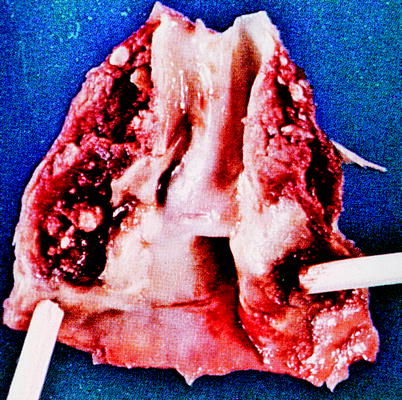
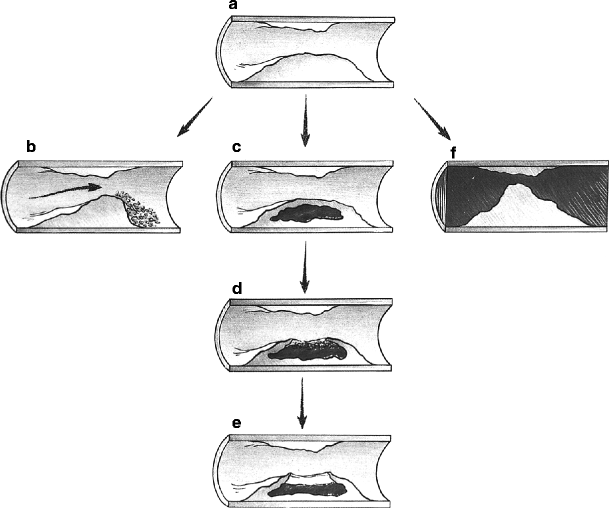
Fig. 6.19
(a) Development of pathologic features in a plaque. (b) Partial obstruction created by a plaque may result in accumulation of a fibrin-platelet thrombus downstream from the obstruction. (c) The plaque may “soften” in its core. (d) This softening may break through to the surface, exposing the contents of the soft core to the bloodstream. (e) An ulceration may become covered by a fibrin deposit and even heal. (f) The increased size of the obstruction created by the plaque may result in thrombosis of the vessel lumen (Courtesy of Springer-Verlag. Berguer and Kieffer [20])
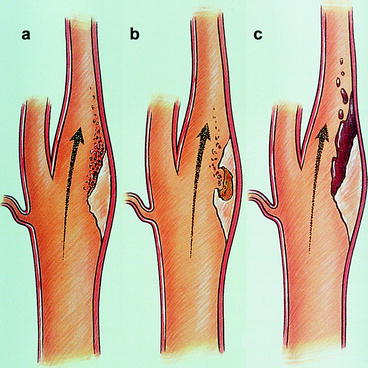
Fig. 6.20
(a–c) Three mechanisms for thromboembolization from an internal carotid plaque. (a) Fibrin-platelet aggregates associated with an obstructing plaque. (b) Atheromatous contents. (c) Thrombus forming on the surface (Courtesy of Springer-Verlag. Berguer and Kieffer [20])
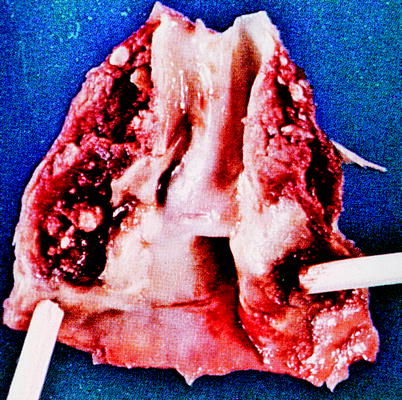
Fig. 6.21
Carotid endarterectomy plaque
Blaisdell et al. [28] and Hass et al. [29] studied the distribution of the atherosclerotic lesions involved in cerebrovascular disease and found that approximately one-third of responsible lesions that were surgically inaccessible occurred in the intracranial distribution. The remaining two-thirds of the lesions were in extracranial locations.
The common carotid bifurcation and the proximal internal carotid artery account for 50% of the lesions. Vertebral artery lesions account for 20%, left subclavian arterial lesions account for 10–15%, and lesions of the innominate and right subclavian arteries account for 15%; and more than one lesion may be present (Fig. 6.22).
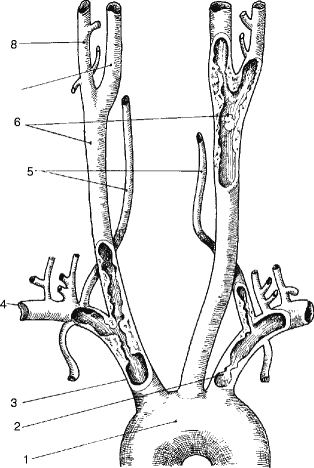
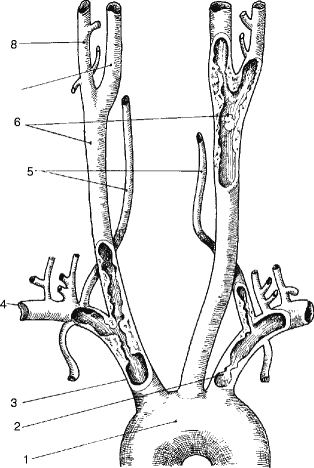
Fig. 6.22
Sites of atherosclerosis of brachiocephalic vessels. (1) Aortic arch, (2) left subclavian artery, (3) innominate artery, (4) right subclavian artery, (5) right and left vertebral arteries, (6) right and left common carotid arteries, (7) right internal carotid artery, (8) right external carotid artery (note atherosclerosis at left subclavian, left vertebral, innominate with proximal right common carotid and subclavian arteries and left carotid bifurcation)
Generally, the most common cause of cerebral ischemic events is embolic phenomena, primarily arterial in origin (carotid) and secondary to cardiac sources. The irregular plaque surface produces turbulence, which will act as a stimulus for platelet aggregation. If the platelet aggregates become large enough and embolize to an important vessel in the brain, symptoms will occur. If the platelet aggregates break up quickly from mechanical forces or from the effect of arterial prostacyclin, the symptoms will be transient, i.e., TIAs. If the embolic fragment persists, however, it can lead to focal infarction (Fig. 6.23). As noted in Fig. 6.24, the end result of the atherosclerotic plaque might be an internal carotid artery thrombosis. When an arteriosclerotic plaque expands to produce a critical reduction in blood flow, the vessel will ultimately undergo thrombosis. In the case of the internal carotid artery, this column of thrombus stops at the ophthalmic artery and remains stable, and if there is sufficient collateral circulation via the circle of Willis, the thrombotic event may be entirely asymptomatic (Fig. 6.24). However, if small thrombi rather than a thrombotic column form and are subsequently carried to the intracranial vessels by continuous blood flow, then the patient will experience cerebral symptoms that can vary from transient amaurosis fugax or hemispheric events to a profound hemiplegia, depending upon the extent of the propagated thrombus or embolus (Fig. 6.25). In addition, if the collateral circulation to the circle of Willis is inadequate, the sudden loss of blood flow through a diseased internal carotid artery may induce a sudden drop in flow to the cerebral hemisphere, resulting in ischemic infarction as a consequence of inadequate proximal blood flow.
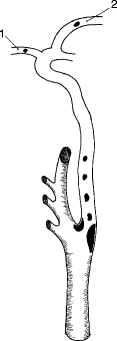
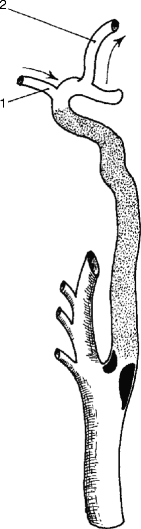
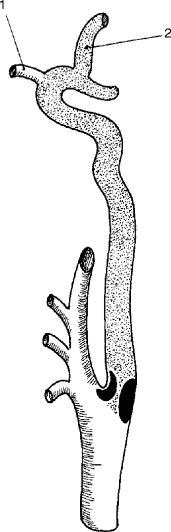
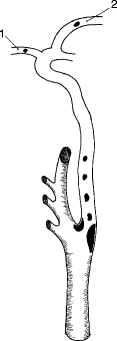
Fig. 6.23
Embolization from internal carotid artery stenotic lesion to ophthalmic artery (1) and middle cerebral artery (2)
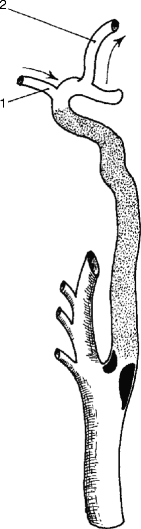
Fig. 6.24
Internal carotid artery thrombosis with retrograde flow via ophthalmic artery (1) to terminal internal carotid artery and middle cerebral artery (2)
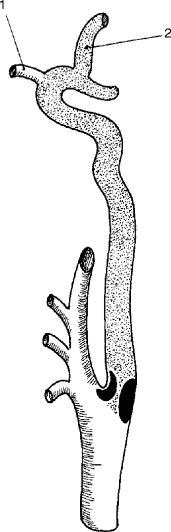
Fig. 6.25
Internal carotid artery thrombosis which extends to ophthalmic artery (1) and terminal internal carotid artery and middle cerebral artery (2)
A less common cause of cerebrovascular ischemia is fibromuscular dysplasia (FMD), which is usually more distally located in the internal carotid artery. The most common form of this disease is characterized by hyperplasia of the media producing alternating bands of thinned areas, leading to a beady appearance on angiography. Often, the internal carotid artery is involved at long lengths. Always look for evidence of fibromuscular dysplasia elsewhere. It will involve both internal carotid arteries in 65% of patients [30].
Pathophysiology
It has been stated that a 70–80% reduction of the cross-sectional area of the arterial lumen must be present to produce a hemodynamically significant drop in the usual flow rate in the cerebrovascular system [31]. However, this may not always be the case. Important mechanisms of collateral flow and hemodynamic events that decrease cardiac output or systemic blood pressure, such as postural hypotension and cardiac arrhythmia, may produce transient episodes of ischemia. Embolization has been a documented cause of TIAs by proven ophthalmologic examination. Intraluminal particles of platelet fibrin aggregates, cholesterol fragments, and small clots have been noted. Most TIAs are probably caused by this mechanism. There is a permanent neurologic deficit as a result of permanently disrupted flow. A pale infarct occurs with focal cell necrosis and cerebral softening. The blood vessels lose integrity at the periphery of the infarct at a relatively ischemic spot. However, where neural death has not occurred, neurologic function may be altered and blood flow, while slow, may still be present.
Clinical Syndromes
The following well-defined syndromes of cerebrovascular ischemia have emerged:
TIAs are focal neurologic symptoms or deficits that usually clear completely within 2 h. Some last for only a few moments, and others last for a few hours, but no longer than 24 h.Stay updated, free articles. Join our Telegram channel
Full access? Get Clinical Tree